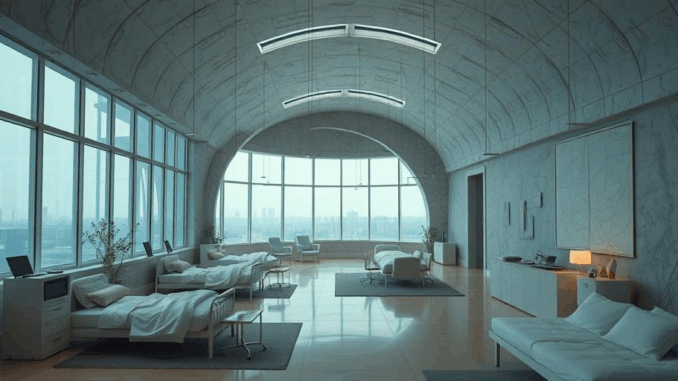
Decoding Sleep Architecture: A Comprehensive Review of Influential Factors, Assessment Techniques, and Therapeutic Interventions
Abstract
Sleep architecture, the structural organization of sleep cycles, is a complex and dynamic process vital for numerous physiological functions, including cognitive processing, immune regulation, and metabolic homeostasis. Disruptions in sleep architecture have been implicated in a wide array of health problems, ranging from cardiovascular disease and mental health disorders to neurodegenerative diseases. This review provides a comprehensive overview of sleep architecture, exploring its key components, the diverse factors that influence it, the methodologies employed for its assessment, and the current therapeutic interventions aimed at improving sleep quality. We delve into the intricate interplay of age, genetics, lifestyle choices, and underlying medical conditions in shaping sleep architecture. Furthermore, we critically evaluate the strengths and limitations of various sleep assessment techniques, including polysomnography (PSG), actigraphy, and subjective sleep questionnaires. Finally, we discuss the efficacy of pharmacological and non-pharmacological interventions, such as cognitive behavioral therapy for insomnia (CBT-I) and pharmacotherapy, in restoring healthy sleep architecture. Understanding the multifaceted nature of sleep architecture is crucial for developing targeted interventions to promote optimal health and well-being.
1. Introduction
Sleep, a fundamental biological necessity, is far from a monolithic state. It is characterized by a highly organized structure, referred to as sleep architecture, which encompasses distinct stages of sleep that cycle throughout the night. These stages, namely non-rapid eye movement (NREM) sleep (further divided into N1, N2, and N3) and rapid eye movement (REM) sleep, each play unique roles in various physiological processes (Carskadon & Dement, 2017). Understanding the dynamics of these stages, their duration, and the transitions between them is critical for comprehending the overall quality and restorative function of sleep. Disruptions in sleep architecture, characterized by reduced slow-wave sleep (SWS), fragmented sleep, or altered REM sleep latency, are increasingly recognized as potential biomarkers for a range of health conditions. For instance, age-related changes in sleep architecture, such as a decrease in SWS and an increase in sleep fragmentation, have been associated with cognitive decline and increased risk of neurodegenerative diseases. Lifestyle factors like shift work, poor sleep hygiene, and chronic stress can also significantly disrupt sleep architecture, leading to daytime dysfunction and increased vulnerability to various health problems (Riemann et al., 2017). The need for a comprehensive understanding of sleep architecture, its influencing factors, and effective interventions is paramount in addressing the growing public health burden of sleep disorders and their associated consequences.
2. Components of Sleep Architecture
Sleep architecture is characterized by a cyclical alternation between NREM and REM sleep, typically recurring every 90-120 minutes. Each stage contributes uniquely to the overall restorative function of sleep.
2.1 NREM Sleep:
NREM sleep constitutes approximately 75-80% of total sleep time in healthy adults and is characterized by a gradual slowing of brain activity and a decrease in physiological arousal. It is further divided into three stages:
- N1 (Stage 1): This is the transition stage between wakefulness and sleep, characterized by slow eye movements, decreased muscle tone, and the appearance of theta waves on electroencephalography (EEG). It typically lasts for a few minutes and is easily disrupted.
- N2 (Stage 2): This stage is marked by the presence of sleep spindles (bursts of high-frequency brain activity) and K-complexes (sharp, negative EEG deflections) on EEG. It represents a deeper level of sleep than N1 and occupies a significant portion of total sleep time.
- N3 (Stage 3): Also known as slow-wave sleep (SWS) or deep sleep, N3 is characterized by the predominance of delta waves (high-amplitude, low-frequency brain waves) on EEG. This stage is crucial for physical restoration, memory consolidation, and immune function. SWS is particularly prominent during the first third of the night and declines with age.
2.2 REM Sleep:
REM sleep is characterized by rapid eye movements, muscle atonia (except for respiratory muscles), and a brain activity pattern similar to that observed during wakefulness. It is associated with vivid dreaming, emotional processing, and memory consolidation. REM sleep latency, the time it takes to enter REM sleep after sleep onset, typically decreases as the night progresses. The proportion of REM sleep increases in the latter half of the sleep period. REM sleep is thought to be critical for cognitive functions and emotional regulation.
3. Factors Influencing Sleep Architecture
Sleep architecture is a complex trait influenced by a multitude of factors, including age, genetics, lifestyle, and underlying medical conditions. Understanding these factors is crucial for identifying individuals at risk of sleep disturbances and developing targeted interventions.
3.1 Age:
Age-related changes in sleep architecture are well-documented. As individuals age, total sleep time tends to decrease, and sleep becomes more fragmented. There is a significant reduction in SWS (N3 sleep), along with an increase in stage N1 sleep. REM sleep duration may also decrease in older adults. These changes are thought to be related to alterations in circadian rhythms, hormonal changes, and an increased prevalence of medical conditions. The decline in SWS is particularly concerning, as it is associated with cognitive decline and impaired memory consolidation (Mander et al., 2017). Aging also influences the circadian drive, with a tendency for older adults to become ‘morning larks’ due to an advanced circadian phase.
3.2 Genetics:
Genetic factors play a significant role in regulating sleep architecture. Twin studies have shown a high heritability for various sleep traits, including sleep duration, sleep latency, and sleep efficiency. Several genes have been implicated in the regulation of sleep, including genes involved in circadian rhythm control (e.g., PER3, CLOCK, CRY). Variations in these genes can influence an individual’s chronotype (morningness vs. eveningness), which in turn affects sleep timing and architecture. Furthermore, genetic predisposition to certain sleep disorders, such as restless legs syndrome and sleep apnea, can also impact sleep architecture. The field of sleep genomics is rapidly advancing, promising to unveil further genetic contributions to sleep regulation (Hublin et al., 2011).
3.3 Lifestyle:
Lifestyle factors exert a profound influence on sleep architecture. These include:
- Diet: Dietary habits, such as caffeine and alcohol consumption, can disrupt sleep architecture. Caffeine, a stimulant, can interfere with sleep onset and reduce SWS. Alcohol, while initially inducing drowsiness, can lead to fragmented sleep and reduced REM sleep in the latter half of the night. High sugar intake, especially before bed, can also disturb sleep patterns. The timing of meals is important also. Eating a large meal late at night can impact sleep quality.
- Physical Activity: Regular physical activity can improve sleep quality and promote healthy sleep architecture. However, intense exercise close to bedtime can be disruptive. The optimal timing and intensity of exercise for promoting sleep vary among individuals. Overall a sedentary lifestyle is associated with poor sleep quality.
- Stress: Chronic stress can lead to hyperarousal and disrupt sleep architecture. Elevated levels of cortisol, a stress hormone, can interfere with sleep onset and reduce SWS. Stress management techniques, such as meditation and mindfulness, can help mitigate the negative effects of stress on sleep. Mental health conditions can impact sleep. For example, Depression is often associated with increased REM sleep latency and increased REM density.
- Sleep Hygiene: Poor sleep hygiene practices, such as inconsistent sleep schedules, exposure to light before bed, and a non-conducive sleep environment, can negatively impact sleep architecture. Establishing a regular sleep-wake schedule, creating a dark, quiet, and cool sleep environment, and avoiding stimulating activities before bed are essential for promoting healthy sleep.
- Shift Work: Shift work, particularly night shifts, disrupts the body’s natural circadian rhythm and can lead to significant alterations in sleep architecture. Shift workers often experience reduced sleep duration, increased sleep fragmentation, and decreased SWS and REM sleep. Chronic shift work is associated with an increased risk of various health problems, including cardiovascular disease, metabolic disorders, and mental health issues.
3.4 Medical Conditions:
Several medical conditions can significantly impact sleep architecture. These include:
- Sleep Disorders: Primary sleep disorders, such as insomnia, sleep apnea, restless legs syndrome, and narcolepsy, are directly related to disruptions in sleep architecture. Insomnia is characterized by difficulty falling asleep or staying asleep, leading to reduced total sleep time and increased sleep fragmentation. Sleep apnea, characterized by repeated interruptions in breathing during sleep, results in frequent arousals and a reduction in SWS and REM sleep. Restless legs syndrome causes an irresistible urge to move the legs, leading to sleep disturbances and fragmented sleep. Narcolepsy, a neurological disorder characterized by excessive daytime sleepiness, is associated with abnormal REM sleep regulation and cataplexy.
- Neurological Disorders: Neurological disorders, such as Alzheimer’s disease, Parkinson’s disease, and stroke, can disrupt sleep architecture. Alzheimer’s disease is associated with reduced SWS and increased sleep fragmentation, which may contribute to cognitive decline. Parkinson’s disease can lead to REM sleep behavior disorder (RBD), characterized by the loss of muscle atonia during REM sleep, resulting in acting out dreams. Stroke can also disrupt sleep architecture, leading to insomnia and sleep apnea. As the population ages, understanding the interaction between sleep quality and the onset of dementia and cognitive decline is a critical focus.
- Psychiatric Disorders: Psychiatric disorders, such as depression, anxiety, and post-traumatic stress disorder (PTSD), are often associated with sleep disturbances. Depression is characterized by increased REM sleep latency and increased REM density. Anxiety can lead to difficulty falling asleep and staying asleep. PTSD can cause nightmares and sleep disturbances, leading to fragmented sleep.
- Cardiovascular Disease: Cardiovascular disease, including heart failure and hypertension, can disrupt sleep architecture. Heart failure can lead to sleep apnea and nocturnal dyspnea (shortness of breath), resulting in frequent arousals and fragmented sleep. Hypertension is associated with an increased risk of sleep apnea. There is growing evidence of a bidirectional relationship between sleep and cardiovascular health.
- Chronic Pain Conditions: Chronic pain conditions, such as fibromyalgia and arthritis, can disrupt sleep architecture. Pain can interfere with sleep onset and maintenance, leading to reduced total sleep time and fragmented sleep. There is a close interaction between pain processing and sleep regulation.
4. Assessment of Sleep Architecture
Accurate assessment of sleep architecture is essential for diagnosing sleep disorders, monitoring treatment response, and conducting sleep research. Several methods are available for assessing sleep architecture, each with its own strengths and limitations.
4.1 Polysomnography (PSG):
PSG is the gold standard for assessing sleep architecture. It involves the simultaneous recording of multiple physiological parameters during sleep, including electroencephalography (EEG), electrooculography (EOG), and electromyography (EMG). EEG measures brain activity, EOG measures eye movements, and EMG measures muscle activity. PSG allows for the identification of different sleep stages (N1, N2, N3, and REM) and the quantification of various sleep parameters, such as sleep latency, total sleep time, sleep efficiency, and the percentage of time spent in each sleep stage. It can detect other sleep related problems like sleep apnea events and nocturnal myoclonus. PSG is typically performed in a sleep laboratory under controlled conditions. While providing a comprehensive assessment of sleep architecture, PSG is time-consuming, expensive, and may be difficult for some individuals to tolerate. First-night effects can also be problematic.
4.2 Actigraphy:
Actigraphy is a non-invasive method for assessing sleep-wake patterns using a wrist-worn device that measures movement. Actigraphy provides an estimate of total sleep time, sleep latency, and sleep efficiency. While actigraphy is less accurate than PSG in identifying specific sleep stages, it is a convenient and cost-effective method for monitoring sleep over extended periods. Actigraphy is particularly useful for assessing sleep in real-world settings and for tracking changes in sleep patterns in response to interventions. Home-based actigraphy is commonly used for longitudinal monitoring and preliminary sleep screening.
4.3 Subjective Sleep Questionnaires:
Subjective sleep questionnaires, such as the Pittsburgh Sleep Quality Index (PSQI) and the Epworth Sleepiness Scale (ESS), are widely used for assessing sleep quality and daytime sleepiness. These questionnaires provide valuable information about an individual’s perception of their sleep, but they are subject to recall bias and may not accurately reflect objective sleep measures. Subjective sleep questionnaires are often used in conjunction with objective sleep measures, such as PSG and actigraphy. These are most effective for identifying sleep problems that might otherwise be missed, such as insomnia. Many people suffering from insomnia are unware that their sleep is not normal.
4.4 Home Sleep Apnea Testing (HSAT):
While not a direct measure of sleep architecture, HSAT can provide valuable information about sleep-disordered breathing, a common condition that disrupts sleep architecture. HSAT typically involves monitoring airflow, respiratory effort, and oxygen saturation during sleep. It is a convenient and cost-effective alternative to PSG for diagnosing sleep apnea in selected patients. However, HSAT does not provide detailed information about sleep stages and may underestimate the severity of sleep apnea in some individuals.
5. Interventions for Improving Sleep Architecture
Improving sleep architecture is essential for promoting optimal health and well-being. Several interventions are available for improving sleep quality and duration, including pharmacological and non-pharmacological approaches.
5.1 Cognitive Behavioral Therapy for Insomnia (CBT-I):
CBT-I is a non-pharmacological treatment for insomnia that focuses on changing maladaptive thoughts and behaviors that contribute to sleep disturbances. CBT-I typically involves several components, including:
- Sleep Hygiene Education: Providing education about sleep hygiene practices, such as establishing a regular sleep-wake schedule, creating a dark, quiet, and cool sleep environment, and avoiding caffeine and alcohol before bed.
- Stimulus Control: Restricting time in bed to the amount of time spent sleeping and avoiding activities in bed other than sleep and sex.
- Sleep Restriction: Limiting time in bed to improve sleep efficiency and consolidate sleep.
- Cognitive Therapy: Identifying and challenging negative thoughts and beliefs about sleep.
- Relaxation Techniques: Teaching relaxation techniques, such as progressive muscle relaxation and deep breathing exercises, to reduce arousal and promote sleep.
CBT-I has been shown to be highly effective in improving sleep quality, reducing sleep latency, and increasing sleep efficiency in individuals with insomnia. It is considered the first-line treatment for chronic insomnia.
5.2 Pharmacotherapy:
Several medications are available for treating insomnia, including:
- Benzodiazepines: Benzodiazepines, such as triazolam and temazepam, are sedative-hypnotic drugs that promote sleep by binding to GABA receptors in the brain. While effective in improving sleep, benzodiazepines can cause side effects, such as daytime sedation, cognitive impairment, and dependence. They should be used cautiously and for short-term treatment only.
- Non-Benzodiazepine Receptor Agonists (Z-drugs): Z-drugs, such as zolpidem, zaleplon, and eszopiclone, are sedative-hypnotic drugs that selectively bind to GABA receptors. They are generally considered to have a lower risk of side effects and dependence than benzodiazepines. However, they can still cause daytime sedation and cognitive impairment.
- Melatonin Receptor Agonists: Melatonin receptor agonists, such as ramelteon, promote sleep by binding to melatonin receptors in the brain. Melatonin is a hormone that regulates the sleep-wake cycle. Melatonin receptor agonists are generally well-tolerated and have a low risk of side effects. They can be particularly useful for treating insomnia in older adults.
- Orexin Receptor Antagonists: Orexin receptor antagonists, such as suvorexant, block the action of orexin, a neurotransmitter that promotes wakefulness. They can be effective in improving sleep onset and maintenance. Orexin receptor antagonists may cause daytime sleepiness and cataplexy-like symptoms in some individuals.
Pharmacotherapy for insomnia should be used under the guidance of a healthcare professional. It is important to consider the potential risks and benefits of each medication and to use the lowest effective dose.
5.3 Other Therapies:
Several other therapies have been shown to be effective in improving sleep architecture, including:
- Bright Light Therapy: Exposure to bright light in the morning can help regulate the circadian rhythm and improve sleep quality. It is particularly useful for treating seasonal affective disorder (SAD) and circadian rhythm sleep disorders.
- Exercise Therapy: Regular physical activity can improve sleep quality and promote healthy sleep architecture. However, intense exercise close to bedtime should be avoided.
- Mindfulness Meditation: Mindfulness meditation can reduce arousal and promote sleep. It involves focusing on the present moment without judgment.
- Acupuncture: Acupuncture has been shown to be effective in improving sleep quality in some individuals with insomnia.
- Supplements: Certain supplements, such as magnesium, valerian root, and chamomile, have been reported to improve sleep quality, although the evidence is limited. More research is required to determine the effectiveness and safety of these supplements. Many dietary supplements are not regulated by the FDA, so the quality can be variable.
6. Future Directions
Research on sleep architecture is rapidly evolving. Future research should focus on the following areas:
- Personalized Sleep Medicine: Developing personalized approaches to sleep management based on individual genetic profiles, lifestyle factors, and medical conditions. This includes identifying biomarkers that predict treatment response and tailoring interventions accordingly.
- Advanced Sleep Monitoring Technologies: Developing more accurate and convenient sleep monitoring technologies, such as wearable sensors and home-based PSG systems. This will facilitate the collection of large-scale sleep data and enable more efficient sleep screening and diagnosis. Artificial Intelligence is increasingly important to sleep analysis and is used to score polysomnography recordings
- The Role of Sleep in Neurodegenerative Diseases: Further investigating the relationship between sleep architecture and neurodegenerative diseases, such as Alzheimer’s disease and Parkinson’s disease. This includes identifying potential therapeutic targets for preventing or slowing the progression of these diseases.
- Sleep and Mental Health: Examining the bidirectional relationship between sleep and mental health, and developing integrated interventions for treating sleep disorders and mental health conditions.
- Social Determinants of Sleep: Considering the impact of social determinants of health, such as socioeconomic status, access to healthcare, and environmental factors, on sleep architecture. Addressing these disparities is essential for promoting sleep equity.
7. Conclusion
Sleep architecture is a complex and dynamic process that is essential for numerous physiological functions. Disruptions in sleep architecture have been implicated in a wide array of health problems. Understanding the various factors that influence sleep architecture, the methodologies employed for its assessment, and the current therapeutic interventions aimed at improving sleep quality is crucial for promoting optimal health and well-being. Future research should focus on developing personalized approaches to sleep management and further investigating the relationship between sleep and various health conditions. By improving our understanding of sleep architecture, we can develop more effective interventions for preventing and treating sleep disorders and promoting a healthier society.
References
- Carskadon, M. A., & Dement, W. C. (2017). Principles and practice of sleep medicine. Elsevier.
- Hublin, C., Kaprio, J., Partinen, M., & Koskenvuo, M. (2011). Heritability of sleep duration: A population-based twin study. Journal of Sleep Research, 20(1 Pt 1), 37-43.
- Mander, B. A., Winer, J. R., & Walker, M. P. (2017). Sleep and human aging. Neuron, 94(1), 19-36.
- Riemann, D., Spiegelhalder, K., Feige, B., Voderholzer, U., Berger, M., Perlis, M., & Nissen, C. (2017). Sleep in the elderly. Clinical Geriatric Medicine, 33(3), 299-314.
- Buysse, D. J., Reynolds III, C. F., Monk, T. H., Berman, S. R., & Kupfer, D. J. (1989). The Pittsburgh Sleep Quality Index: a new instrument for psychiatric practice and research. Psychiatry Research, 28(2), 193–213.
- Johns, M. W. (1991). A new method for measuring daytime sleepiness: the Epworth sleepiness scale. Sleep, 14(6), 540–545.
This comprehensive review highlights the critical role of sleep architecture in overall health. The discussion of personalized sleep medicine and advanced monitoring technologies points toward exciting advancements in tailoring interventions for individual needs and improving sleep disorder diagnoses.
Thanks for your comment! I’m glad you found the discussion of personalized sleep medicine interesting. The potential for tailoring interventions based on individual needs is a really exciting area of development. Imagine a future where sleep disorder diagnoses can be improved through the use of advanced monitoring technologies.
Editor: MedTechNews.Uk
Thank you to our Sponsor Esdebe