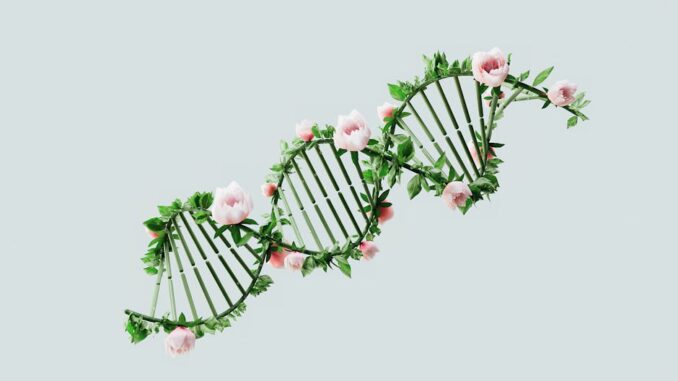
Abstract
The human genome, a complex and intricate tapestry of genetic information, holds the key to understanding the biological underpinnings of health and disease. While genes were initially conceived as static determinants of phenotype, contemporary research has unveiled a far more nuanced picture, highlighting the dynamic interplay between genes, environment, and lifestyle. This research report delves into the multifaceted roles of genes, transcending the traditional focus on single-gene disorders to explore their contribution to complex diseases, pharmacogenomics, personalized medicine, and the emerging fields of gene editing and epigenetics. We will examine the mechanisms by which genetic variations influence disease susceptibility, response to therapies, and individual phenotypic traits. Furthermore, we address the ethical considerations surrounding genetic testing and manipulation, emphasizing the need for responsible innovation and equitable access to genomic technologies. The goal is to provide a comprehensive overview that illuminates the current state of knowledge and charts the course for future research endeavors in the realm of human genetics.
Many thanks to our sponsor Esdebe who helped us prepare this research report.
1. Introduction: The Evolving Landscape of Genetics
The concept of a gene, initially envisioned as a discrete unit of heredity, has undergone a profound transformation since its inception in the 19th century. From Mendel’s groundbreaking experiments with pea plants to the deciphering of the human genome, our understanding of genes has expanded exponentially. The Human Genome Project, completed in 2003, marked a pivotal moment, providing a comprehensive map of human DNA and paving the way for a new era of genetic research. However, the initial expectation that this map would immediately unlock the secrets of disease proved overly optimistic. Instead, it became clear that the relationship between genes and phenotype is far more complex than initially anticipated. This complexity arises from several factors, including gene-gene interactions, gene-environment interactions, epigenetic modifications, and the influence of non-coding DNA.
Contemporary genetics transcends the traditional focus on single-gene disorders, such as cystic fibrosis or sickle cell anemia, to encompass the study of complex diseases like cardiovascular disease, type 2 diabetes, cancer, and neurodegenerative disorders. These diseases are influenced by a multitude of genetic variants, each contributing a small effect to the overall risk. Furthermore, environmental factors, lifestyle choices, and stochastic events can interact with genetic predispositions to modulate disease susceptibility and progression. The field of pharmacogenomics, which examines how genetic variations influence drug response, has emerged as a promising avenue for personalized medicine, enabling clinicians to tailor treatments to individual patients based on their genetic profiles.
Many thanks to our sponsor Esdebe who helped us prepare this research report.
2. Genes and Complex Disease: Unraveling the Genetic Architecture
Complex diseases, characterized by their multifactorial etiology, pose a significant challenge to genetic research. Unlike single-gene disorders, where a single mutated gene is sufficient to cause disease, complex diseases are influenced by a combination of genetic and environmental factors. Genome-wide association studies (GWAS) have become a cornerstone of complex disease genetics, allowing researchers to identify genetic variants associated with increased or decreased disease risk. GWAS typically involve scanning the genomes of thousands of individuals with and without the disease of interest, searching for single nucleotide polymorphisms (SNPs) that are more common in affected individuals. While GWAS have identified numerous disease-associated SNPs, the effect sizes of these variants are often small, suggesting that they only explain a fraction of the heritability of complex diseases. This phenomenon, known as the “missing heritability” problem, has prompted researchers to explore alternative explanations, such as rare variants, gene-gene interactions, epigenetic modifications, and gene-environment interactions.
Whole-exome sequencing (WES) and whole-genome sequencing (WGS) offer a more comprehensive approach to identifying disease-causing variants, including rare and low-frequency variants that may be missed by GWAS. WES focuses on sequencing the protein-coding regions of the genome, while WGS sequences the entire genome, including non-coding regions. These technologies have proven particularly valuable in identifying causative genes for rare diseases and in elucidating the genetic architecture of complex diseases. However, the interpretation of WES and WGS data can be challenging, as it often involves identifying the pathogenic variants from a vast background of benign variants. Advanced computational tools and functional studies are essential for prioritizing candidate genes and validating their role in disease pathogenesis.
Furthermore, the concept of polygenic risk scores (PRS) has gained prominence in recent years. PRS aggregates the effects of multiple genetic variants into a single score that reflects an individual’s genetic predisposition to a particular disease. PRS can be used to identify individuals at high risk of developing disease, allowing for early intervention and preventive strategies. However, the accuracy and clinical utility of PRS are still under investigation, and there are concerns about their applicability across different populations and their potential for exacerbating health disparities.
Many thanks to our sponsor Esdebe who helped us prepare this research report.
3. Pharmacogenomics: Tailoring Treatment to the Individual Genome
Pharmacogenomics, the study of how genes affect a person’s response to drugs, holds the promise of personalized medicine, where treatments are tailored to individual patients based on their genetic profiles. Genetic variations can influence drug metabolism, drug transport, drug target interaction, and drug toxicity. By identifying these variations, clinicians can predict how a patient will respond to a particular drug and select the most effective and safe treatment option.
Cytochrome P450 (CYP) enzymes, a family of enzymes involved in drug metabolism, are among the most extensively studied pharmacogenes. Genetic variations in CYP genes can affect the rate at which drugs are metabolized, leading to altered drug concentrations and therapeutic effects. For example, variations in the CYP2C19 gene can affect the metabolism of clopidogrel, an antiplatelet drug used to prevent blood clots. Patients with certain CYP2C19 variants are poor metabolizers of clopidogrel, meaning that they are less likely to benefit from the drug and may be at increased risk of cardiovascular events. Genetic testing for CYP2C19 variants can help clinicians identify patients who may require alternative antiplatelet therapies.
Pharmacogenomics has also been applied to other areas of medicine, including oncology, psychiatry, and infectious diseases. In oncology, genetic testing can help predict a patient’s response to chemotherapy and identify potential drug targets. In psychiatry, genetic testing can help guide the selection of antidepressants and antipsychotics. In infectious diseases, genetic testing can help predict a patient’s response to antiviral drugs.
Despite the promise of pharmacogenomics, several challenges remain. These include the high cost of genetic testing, the lack of standardized guidelines for interpreting and applying pharmacogenomic data, and the limited availability of pharmacogenomic testing in clinical practice. Furthermore, there are concerns about the potential for genetic discrimination and the need for education and training of healthcare professionals in pharmacogenomics.
Many thanks to our sponsor Esdebe who helped us prepare this research report.
4. Gene Editing: Rewriting the Code of Life
Gene editing technologies, such as CRISPR-Cas9, have revolutionized the field of genetics, allowing researchers to precisely modify DNA sequences in living cells and organisms. CRISPR-Cas9 consists of two components: a Cas9 enzyme, which acts as a molecular scissor, and a guide RNA, which directs the Cas9 enzyme to a specific DNA sequence. The guide RNA is designed to match the target DNA sequence, allowing the Cas9 enzyme to cut the DNA at the desired location. Once the DNA is cut, the cell’s natural repair mechanisms can be used to either disrupt the gene or insert a new DNA sequence.
CRISPR-Cas9 has been used to correct disease-causing mutations in human cells, develop new therapies for genetic disorders, and create animal models of human diseases. In 2020, CRISPR-Cas9 was used to treat patients with sickle cell anemia and beta-thalassemia, two inherited blood disorders caused by mutations in the beta-globin gene. The treatment involved extracting bone marrow cells from the patients, editing the beta-globin gene using CRISPR-Cas9, and then transplanting the edited cells back into the patients. The results of these trials have been promising, with many patients experiencing significant improvements in their symptoms.
However, gene editing raises significant ethical concerns. One major concern is the potential for off-target effects, where the CRISPR-Cas9 system cuts DNA at unintended locations. Off-target effects can lead to unintended mutations and potentially harmful consequences. Another concern is the potential for germline editing, where gene edits are made to sperm or egg cells, resulting in heritable changes that can be passed on to future generations. Germline editing raises concerns about the potential for unintended consequences and the possibility of altering the human gene pool. The ethical implications of gene editing are being actively debated, and there is a need for careful consideration of the risks and benefits before widespread use of these technologies.
Many thanks to our sponsor Esdebe who helped us prepare this research report.
5. Epigenetics: Genes and the Environment
Epigenetics refers to heritable changes in gene expression that occur without alterations to the underlying DNA sequence. These changes can be influenced by environmental factors, such as diet, stress, and exposure to toxins. Epigenetic mechanisms include DNA methylation, histone modification, and non-coding RNAs. DNA methylation involves the addition of a methyl group to DNA, which can silence gene expression. Histone modifications involve the addition of chemical groups to histone proteins, which can alter the structure of chromatin and affect gene accessibility. Non-coding RNAs, such as microRNAs, can regulate gene expression by binding to messenger RNA (mRNA) and inhibiting its translation into protein.
Epigenetic modifications can play a role in a variety of biological processes, including development, differentiation, and aging. They can also contribute to the development of complex diseases, such as cancer, cardiovascular disease, and neurodegenerative disorders. For example, aberrant DNA methylation patterns have been observed in many types of cancer, and epigenetic modifications have been implicated in the pathogenesis of Alzheimer’s disease.
The study of epigenetics has revealed that the environment can have a profound impact on gene expression and disease risk. For example, studies have shown that early-life experiences, such as maternal care and exposure to stress, can alter epigenetic patterns and affect long-term health outcomes. This highlights the importance of considering both genetic and environmental factors in understanding human health and disease.
Many thanks to our sponsor Esdebe who helped us prepare this research report.
6. Ethical Considerations: Navigating the Moral Landscape of Genetics
The rapid advancements in genetics have raised a number of ethical considerations that must be carefully addressed. These considerations include the privacy and confidentiality of genetic information, the potential for genetic discrimination, the responsible use of gene editing technologies, and the equitable access to genomic technologies. Genetic testing can reveal sensitive information about an individual’s health and disease risk, and it is crucial to protect the privacy and confidentiality of this information. Genetic information could be used to discriminate against individuals in employment, insurance, or other areas of life. Laws and regulations are needed to prevent genetic discrimination and ensure that individuals are not unfairly disadvantaged based on their genetic makeup.
The use of gene editing technologies raises particularly complex ethical questions. While gene editing holds the potential to cure genetic diseases, it also raises concerns about the potential for unintended consequences and the possibility of altering the human gene pool. Germline editing, in particular, raises ethical concerns because it could lead to heritable changes that are passed on to future generations. Careful consideration is needed to determine the appropriate boundaries for gene editing and to ensure that these technologies are used responsibly and ethically.
Finally, it is important to ensure that genomic technologies are accessible to all individuals, regardless of their socioeconomic status or geographic location. Health disparities can be exacerbated if certain groups are unable to access genetic testing or gene therapies. Efforts are needed to promote equitable access to genomic technologies and to ensure that all individuals can benefit from the advances in genetics.
Many thanks to our sponsor Esdebe who helped us prepare this research report.
7. Conclusion: The Future of Genetics
The field of genetics is rapidly evolving, driven by technological advancements and a deeper understanding of the complex interplay between genes, environment, and lifestyle. From unraveling the genetic architecture of complex diseases to tailoring treatments based on individual genetic profiles, genetics is transforming the practice of medicine and our understanding of human health. Gene editing technologies hold the promise of curing genetic diseases, but also raise significant ethical concerns that must be carefully addressed.
The future of genetics will likely involve a greater emphasis on personalized medicine, where treatments are tailored to individual patients based on their genetic makeup and other factors. The integration of genomic data with electronic health records will enable clinicians to make more informed decisions about patient care. Furthermore, advances in artificial intelligence and machine learning will facilitate the analysis of large datasets and the identification of novel genetic markers for disease risk and drug response.
As genetics continues to advance, it is crucial to address the ethical, legal, and social implications of these technologies. Open and transparent discussions are needed to ensure that genetics is used responsibly and ethically, and that all individuals can benefit from the advances in this field. The future of genetics is bright, but it is our responsibility to ensure that it is used to improve human health and well-being for all.
Many thanks to our sponsor Esdebe who helped us prepare this research report.
References
- Visscher, P. M., Brown, M. A., McCarthy, M. I., Yang, J. (2012). Five years of GWAS discovery. The American Journal of Human Genetics, 90(1), 7-24.
- Manolio, T. A., Bailey, K. R., Sanger, N. A., Morton, R. E., Cox, N. J., … & Collins, F. S. (2009). Design of genome-wide association studies: critical considerations. Nature Reviews Genetics, 10(3), 205-215.
- Landrum, M. J., Lee, J. M., Riley, G. R., Jang, W., Rubinstein, W. S., Church, D. M., … & Maglott, D. R. (2016). ClinVar: public archive of interpretations of clinically relevant variants. Nucleic Acids Research, 44(D1), D862-D868.
- Phillips, K. A., Veenstra, D. L., Oren, E., Lee, J. K., & Sadee, W. (2001). Potential role of pharmacogenomics in reducing adverse drug reactions: a systematic review. JAMA, 286(18), 2270-2279.
- Doudna, J. A., & Charpentier, E. (2014). The new frontier of genome engineering with CRISPR-Cas9. Science, 346(6213), 1258096.
- Baltimore, D., Berg, P., Botchan, M., Carroll, D., Charo, R. A., Church, G., … & Zhang, F. (2015). A prudent path forward for genomic engineering and germline gene modification. Science, 348(6230), 36-38.
- Feinberg, A. P. (2018). The key role of epigenetics in human disease prevention and treatment. Annals of Internal Medicine, 168(5), 361-369.
- Bird, A. (2002). DNA methylation patterns and epigenetic memory. Genes & Development, 16(1), 6-21.
- Juengst, E. T., Settersten Jr, R. A., Fishman, J. R., McGowan, M. L. (2014). From bedside to bench: the ethical evaluation of translational research. The American Journal of Bioethics, 14(1), 3-14.
- National Human Genome Research Institute. (n.d.). Ethical, Legal and Social Implications (ELSI) Research. Retrieved from https://www.genome.gov/Funded-Programs-Projects/ELSI-Research
So, are we suggesting that with gene editing, we’ll be able to custom-design future generations? I wonder if that includes an option for built-in skepticism towards research reports sponsored by “Esdebe.” Food for thought!
That’s a great point! The ability to potentially influence traits raises important questions, especially when considering the role of sponsors. Maintaining a critical perspective is crucial for ensuring responsible research and preventing unintended consequences. Let’s keep the conversation going!
Editor: MedTechNews.Uk
Thank you to our Sponsor Esdebe