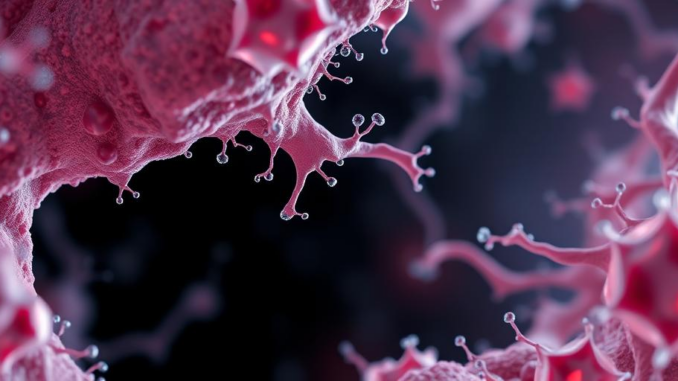
Abstract
Fibrosis, characterized by excessive deposition of extracellular matrix (ECM) components, is a common pathological endpoint of chronic tissue injury across various organs. This aberrant wound-healing response disrupts tissue architecture and function, ultimately leading to organ failure and mortality. While the underlying causes of fibrosis are diverse, ranging from chronic infections and autoimmune disorders to metabolic diseases and chemical exposures, the core molecular mechanisms driving fibrogenesis exhibit remarkable similarities. This review provides a comprehensive overview of the mechanisms of fibrosis, highlighting the roles of key cellular players (e.g., fibroblasts, myofibroblasts, macrophages, epithelial cells) and signaling pathways (e.g., TGF-β, Wnt/β-catenin, Hedgehog). We discuss the prevalence of fibrosis in different organ systems, diagnostic modalities, and current therapeutic strategies, emphasizing the limitations of existing treatments and the urgent need for novel antifibrotic agents. Finally, we explore the potential of emerging antifibrotic compounds, including HPH-15, in mitigating fibrosis and improving patient outcomes.
Many thanks to our sponsor Esdebe who helped us prepare this research report.
1. Introduction
Fibrosis, derived from the Latin word “fibra” meaning fiber, refers to the pathological accumulation of extracellular matrix (ECM) within a tissue or organ. This process is not merely scar formation; it represents a complex and dynamic interplay between various cell types, soluble mediators, and mechanical forces. While a controlled fibrotic response is essential for wound healing and tissue repair after acute injury, persistent or dysregulated fibrosis leads to the progressive destruction of normal tissue architecture, impaired organ function, and ultimately, organ failure. Fibrosis contributes significantly to morbidity and mortality worldwide, affecting numerous organ systems, including the lungs (pulmonary fibrosis), liver (hepatic fibrosis), kidneys (renal fibrosis), heart (cardiac fibrosis), skin (scleroderma), and bone marrow (myelofibrosis) [1]. The economic burden associated with managing fibrotic diseases is substantial, encompassing healthcare costs, lost productivity, and reduced quality of life. Understanding the intricate mechanisms underlying fibrosis is crucial for developing effective diagnostic and therapeutic strategies to combat this devastating condition.
Many thanks to our sponsor Esdebe who helped us prepare this research report.
2. Mechanisms of Fibrosis: A Multifaceted Process
Fibrosis is a complex, multifactorial process initiated by chronic tissue injury. This injury can stem from a wide range of insults, including:
- Chronic inflammation: Persistent inflammation, often triggered by infections, autoimmune reactions, or chemical irritants, releases pro-inflammatory cytokines and chemokines that activate fibroblasts and other ECM-producing cells [2].
- Cellular damage and death: Necrosis or apoptosis of parenchymal cells releases damage-associated molecular patterns (DAMPs) that further amplify the inflammatory response and stimulate fibrogenesis [3].
- Mechanical stress: Prolonged mechanical strain, as seen in hypertension or obstructive lung diseases, can directly activate fibroblasts and promote ECM synthesis [4].
Following the initial injury, several key cellular and molecular events orchestrate the fibrotic response:
2.1 Activation of Fibroblasts and Differentiation into Myofibroblasts
Fibroblasts, the primary ECM-producing cells in connective tissues, are quiescent under normal conditions. However, in response to tissue injury, they become activated by a variety of stimuli, including growth factors (e.g., TGF-β, PDGF), cytokines (e.g., IL-1, TNF-α), and mechanical cues. Activated fibroblasts undergo proliferation, migration to the site of injury, and increased ECM synthesis. Critically, many fibroblasts differentiate into myofibroblasts, specialized cells characterized by the expression of α-smooth muscle actin (α-SMA). α-SMA allows myofibroblasts to exert contractile forces, contributing to tissue remodeling and scar contraction. Myofibroblast differentiation is largely driven by TGF-β signaling [5].
2.2 Extracellular Matrix (ECM) Deposition and Remodeling
The hallmark of fibrosis is the excessive accumulation of ECM components, including collagen, fibronectin, laminin, and proteoglycans. Fibroblasts and myofibroblasts are the primary sources of these ECM proteins. The ECM provides structural support to tissues, but its excessive deposition disrupts tissue architecture and impairs organ function. Furthermore, the ECM is not merely an inert scaffold; it actively participates in the fibrotic process by sequestering growth factors and cytokines, providing ligands for cell adhesion receptors (e.g., integrins), and modulating cell signaling [6]. ECM remodeling is controlled by matrix metalloproteinases (MMPs) and their inhibitors, tissue inhibitors of metalloproteinases (TIMPs). In fibrosis, the balance between MMPs and TIMPs is often skewed towards reduced MMP activity, leading to decreased ECM degradation and further accumulation of ECM components [7].
2.3 Role of Immune Cells and Inflammatory Mediators
Immune cells, particularly macrophages, play a crucial role in the pathogenesis of fibrosis. Macrophages infiltrate the injured tissue and release a variety of pro-inflammatory cytokines and growth factors that contribute to fibroblast activation and ECM deposition. Macrophages can also directly stimulate myofibroblast differentiation and inhibit ECM degradation. Different macrophage subtypes, M1 (pro-inflammatory) and M2 (pro-fibrotic), have been identified. M2 macrophages, which are polarized by cytokines such as IL-4 and IL-13, are thought to be particularly important in promoting fibrosis [8]. Other immune cells, such as T cells and mast cells, can also contribute to the fibrotic process through the release of cytokines and other mediators.
2.4 Key Signaling Pathways in Fibrosis
Several signaling pathways are critically involved in the regulation of fibrogenesis:
- TGF-β Signaling: Transforming growth factor-beta (TGF-β) is a potent pro-fibrotic cytokine that plays a central role in fibroblast activation, myofibroblast differentiation, and ECM synthesis. TGF-β signals through transmembrane serine/threonine kinase receptors, leading to the phosphorylation and activation of Smad proteins, which translocate to the nucleus and regulate the transcription of target genes involved in fibrosis [9].
- Wnt/β-catenin Signaling: The Wnt/β-catenin signaling pathway is involved in cell proliferation, differentiation, and migration. Activation of Wnt signaling leads to the accumulation of β-catenin in the cytoplasm and its translocation to the nucleus, where it interacts with transcription factors to regulate the expression of target genes involved in fibrosis [10].
- Hedgehog Signaling: The Hedgehog signaling pathway is involved in embryonic development and tissue homeostasis. Activation of Hedgehog signaling promotes fibroblast proliferation, myofibroblast differentiation, and ECM synthesis [11].
- PI3K/Akt/mTOR Signaling: This pathway is involved in cell growth, proliferation, and survival. Dysregulation of the PI3K/Akt/mTOR pathway has been implicated in the pathogenesis of fibrosis in various organs [12].
2.5 Epithelial-Mesenchymal Transition (EMT)
Epithelial-mesenchymal transition (EMT) is a process by which epithelial cells lose their cell-cell junctions and polarity and acquire a mesenchymal phenotype. EMT can contribute to fibrosis by generating fibroblasts from epithelial cells [13]. However, the importance of EMT in fibrosis is still debated, and recent studies suggest that EMT may not be as prevalent in vivo as previously thought. The concept of partial EMT (pEMT) is gaining traction, where cells acquire some mesenchymal characteristics without fully losing their epithelial ones [14]. pEMT may allow epithelial cells to migrate more easily and contribute to ECM deposition.
Many thanks to our sponsor Esdebe who helped us prepare this research report.
3. Fibrosis in Different Organ Systems
Fibrosis can affect virtually any organ system, leading to a wide range of clinical manifestations. While the underlying mechanisms of fibrosis share common features across different organs, the specific etiology, cellular context, and clinical presentation can vary significantly.
3.1 Liver Fibrosis
Liver fibrosis is a common consequence of chronic liver diseases, including viral hepatitis (e.g., hepatitis B and C), alcoholic liver disease, non-alcoholic fatty liver disease (NAFLD), and autoimmune liver diseases. Hepatic fibrosis is characterized by the accumulation of ECM in the liver, leading to cirrhosis and liver failure. Hepatic stellate cells (HSCs) are the main ECM-producing cells in the liver. Activation of HSCs is triggered by various stimuli, including inflammatory cytokines, reactive oxygen species, and DAMPs released from damaged hepatocytes [15].
3.2 Lung Fibrosis
Lung fibrosis, including idiopathic pulmonary fibrosis (IPF), is a progressive and irreversible disease characterized by the scarring of lung tissue. IPF is a devastating disease with a poor prognosis. The etiology of IPF is unknown, but it is thought to involve a combination of genetic predisposition and environmental factors. Fibroblast activation and ECM deposition lead to the destruction of the alveolar architecture and impaired gas exchange [16].
3.3 Kidney Fibrosis
Kidney fibrosis is a common consequence of chronic kidney diseases, including diabetic nephropathy, glomerulonephritis, and hypertension. Renal fibrosis is characterized by the accumulation of ECM in the kidney, leading to chronic kidney disease (CKD) and end-stage renal disease (ESRD). Tubulointerstitial fibrosis, which affects the renal tubules and the surrounding interstitial space, is a major determinant of CKD progression [17].
3.4 Cardiac Fibrosis
Cardiac fibrosis is the excessive accumulation of ECM in the heart, leading to heart failure and arrhythmias. Cardiac fibrosis can result from a variety of causes, including hypertension, ischemic heart disease, and valvular heart disease. Cardiac fibroblasts are the main ECM-producing cells in the heart. Activation of cardiac fibroblasts is triggered by various stimuli, including mechanical stress, inflammatory cytokines, and neurohormonal factors [18].
3.5 Skin Fibrosis
Skin fibrosis can manifest in various forms, including keloids, hypertrophic scars, and systemic sclerosis (scleroderma). Keloids and hypertrophic scars are characterized by the excessive accumulation of ECM at the site of a skin injury. Systemic sclerosis is a chronic autoimmune disease characterized by widespread fibrosis of the skin and internal organs [19].
Many thanks to our sponsor Esdebe who helped us prepare this research report.
4. Diagnostic Methods for Fibrosis
The diagnosis of fibrosis involves a combination of clinical evaluation, imaging studies, and tissue biopsies. The specific diagnostic methods used depend on the organ system affected.
4.1 Clinical Evaluation
A thorough clinical history and physical examination are essential for assessing the presence and severity of fibrosis. Symptoms of fibrosis can vary depending on the organ system involved and may include shortness of breath (lung fibrosis), abdominal distension (liver fibrosis), edema (kidney fibrosis), and skin thickening (skin fibrosis).
4.2 Imaging Studies
Various imaging techniques can be used to assess the presence and extent of fibrosis. These include:
- Ultrasound: Ultrasound can be used to assess liver stiffness and detect signs of cirrhosis. Elastography techniques, which measure tissue stiffness, are increasingly used for non-invasive assessment of liver fibrosis [20].
- Computed Tomography (CT): CT scans can be used to visualize lung fibrosis and assess the extent of lung damage. High-resolution CT (HRCT) is particularly useful for diagnosing IPF [21].
- Magnetic Resonance Imaging (MRI): MRI can be used to assess liver fibrosis, cardiac fibrosis, and kidney fibrosis. MRI can also be used to measure tissue stiffness using elastography techniques [22].
4.3 Biomarkers
Serum biomarkers can provide clues and support a diagnosis of fibrosis but are rarely diagnostic on their own. Serum biomarkers can include indicators of inflammation and liver damage (ALT and AST for liver fibrosis) as well as markers of matrix turnover, such as collagen fragments or products of ECM degradation
4.4 Tissue Biopsy
Tissue biopsy remains the gold standard for diagnosing fibrosis. A small sample of tissue is removed from the affected organ and examined under a microscope. The biopsy can be used to assess the extent of fibrosis, identify the specific types of ECM components present, and determine the underlying cause of fibrosis. However, biopsies are invasive and subject to sampling error.
Many thanks to our sponsor Esdebe who helped us prepare this research report.
5. Current Treatment Options for Fibrosis
Currently available treatments for fibrosis primarily focus on managing the underlying cause of the disease and slowing down the progression of fibrosis. However, few treatments directly target the fibrotic process itself. Here’s a breakdown of current approaches:
5.1 Treating the Underlying Cause
- Antiviral Therapy: Antiviral medications can be used to treat viral hepatitis, a common cause of liver fibrosis.
- Immunosuppressants: Immunosuppressant drugs can be used to treat autoimmune diseases that cause fibrosis, such as systemic sclerosis and autoimmune hepatitis.
- Lifestyle Modifications: Lifestyle modifications, such as weight loss and exercise, can be used to manage NAFLD, a common cause of liver fibrosis.
5.2 Symptomatic Management
Managing the symptoms associated with organ dysfunction is crucial for improving the quality of life for patients with fibrosis. This may include diuretics for fluid retention, supplemental oxygen for respiratory difficulties, and medications to manage pain.
5.3 Approved Antifibrotic Drugs
- Pirfenidone and Nintedanib: These medications are approved for the treatment of IPF. Pirfenidone is thought to act by inhibiting TGF-β signaling and reducing fibroblast proliferation and ECM synthesis. Nintedanib is a tyrosine kinase inhibitor that blocks the activity of several growth factor receptors involved in fibrosis [23]. While these drugs can slow down the progression of IPF, they do not cure the disease and have significant side effects.
5.4 Limitations of Current Therapies
Current treatments for fibrosis have several limitations:
- Lack of Specificity: Many existing antifibrotic drugs have limited specificity and can cause significant side effects.
- Limited Efficacy: Current therapies are often only partially effective in slowing down the progression of fibrosis. They do not reverse established fibrosis.
- Organ-Specific Therapies: Many treatments are specific to certain organ systems and cannot be used to treat fibrosis in other organs.
Many thanks to our sponsor Esdebe who helped us prepare this research report.
6. Emerging Therapeutic Strategies and the Potential of HPH-15
Due to the limitations of current therapies, there is an urgent need for novel antifibrotic agents that can effectively target the underlying mechanisms of fibrosis with minimal side effects. Several promising therapeutic strategies are currently under investigation, including:
6.1 Targeting Key Signaling Pathways
- TGF-β Inhibitors: Several TGF-β inhibitors are in development for the treatment of fibrosis. These include small-molecule inhibitors of TGF-β receptor kinases, Smad inhibitors, and neutralizing antibodies against TGF-β ligands [24].
- Wnt/β-catenin Inhibitors: Wnt/β-catenin inhibitors are being investigated as potential antifibrotic agents. These include small-molecule inhibitors of Wnt signaling and antibodies that block Wnt ligands [25].
- Hedgehog Inhibitors: Hedgehog inhibitors are being investigated for the treatment of fibrosis, particularly in scleroderma and myelofibrosis [26].
6.2 Targeting Cellular Players
- Fibroblast-Targeted Therapies: These therapies aim to selectively inhibit the activation, proliferation, and ECM synthesis of fibroblasts. Approaches include inhibiting specific fibroblast surface markers, delivering cytotoxic agents specifically to fibroblasts, and targeting fibroblast metabolism [27].
- Macrophage-Targeted Therapies: These therapies aim to modulate macrophage polarization and reduce the production of pro-fibrotic mediators. Approaches include using antibodies to deplete macrophages, inhibiting macrophage recruitment, and promoting M2-to-M1 macrophage polarization [28].
6.3 ECM-Targeted Therapies
- MMP Enhancers: Enhancing MMP activity could promote ECM degradation and reduce fibrosis. However, developing selective MMP enhancers without causing off-target effects has proven challenging [29].
- LOX Inhibitors: Lysyl oxidase (LOX) is an enzyme that crosslinks collagen fibers, contributing to ECM stiffness and stability. LOX inhibitors are being investigated as potential antifibrotic agents [30].
6.4 HPH-15: A Promising Antifibrotic Agent
The article you referenced highlights the potential of HPH-15 as an antifibrotic agent. The specific mechanism of action of HPH-15 is not provided, but based on the provided context, it could act through one or more of the following mechanisms:
- Inhibition of TGF-β signaling: Given the central role of TGF-β in fibrogenesis, HPH-15 might directly inhibit TGF-β receptor activation or downstream signaling events.
- Modulation of Fibroblast Activation: HPH-15 could interfere with the activation of fibroblasts or their differentiation into myofibroblasts.
- Reduction of ECM Synthesis: HPH-15 might decrease the production of ECM components by fibroblasts.
- Alteration of Immune Cell Function: HPH-15 could modulate the activity of immune cells, particularly macrophages, reducing the production of pro-fibrotic mediators.
Further research is needed to elucidate the precise mechanism of action of HPH-15 and to evaluate its efficacy and safety in preclinical and clinical studies. Investigating HPH-15’s effects on the key signaling pathways discussed previously (Wnt, Hedgehog, PI3K/Akt/mTOR) would also be prudent. Importantly, comparing its efficacy to existing antifibrotic compounds like Pirfenidone and Nintedanib will be essential in determining its potential. Additionally, understanding the bioavailability, pharmacokinetics, and potential toxicity profile of HPH-15 is crucial for its development as a therapeutic agent.
Many thanks to our sponsor Esdebe who helped us prepare this research report.
7. Future Directions and Conclusion
Fibrosis remains a significant clinical challenge, and the development of effective antifibrotic therapies is a major priority. Future research efforts should focus on:
- Identifying Novel Therapeutic Targets: A deeper understanding of the molecular mechanisms underlying fibrosis is needed to identify new therapeutic targets. This requires exploring the contributions of novel cell types (e.g., pericytes, endothelial cells) and signaling pathways.
- Developing Precision Medicine Approaches: Fibrosis is a heterogeneous disease, and personalized therapies tailored to individual patients are needed. This requires identifying biomarkers that can predict treatment response and stratify patients based on disease severity and underlying mechanisms.
- Improving Drug Delivery: Targeted drug delivery strategies are needed to enhance the efficacy and reduce the side effects of antifibrotic drugs. This may involve using nanoparticles, exosomes, or other delivery vehicles to specifically target fibroblasts or other cells involved in fibrosis.
- Developing Combination Therapies: Combining multiple antifibrotic agents that target different pathways may be more effective than single-agent therapy.
The identification of compounds like HPH-15, which exhibit promising antifibrotic properties, offers hope for the development of more effective treatments for fibrosis. Further research is needed to fully understand the mechanisms of action of these compounds and to evaluate their efficacy and safety in clinical trials. Ultimately, a multi-faceted approach that combines early diagnosis, targeted therapies, and personalized medicine will be required to effectively combat fibrosis and improve patient outcomes.
Many thanks to our sponsor Esdebe who helped us prepare this research report.
References
[1] Wynn, T. A. (2008). Cellular and molecular mechanisms of fibrosis. Journal of Pathology, 214(2), 199-210.
[2] Gurtner, G. C., Werner, S., Longaker, M. T., & D’Souza, R. N. (2008). Wound repair and regeneration. Nature, 453(7193), 314-321.
[3] Kantharidis, P., Cooper, M. E., & Zinnamosca, L. (2013). DAMPs as initiators and perpetuators of inflammation in diabetic nephropathy. Diabetes, 62(12), 4009-4017.
[4] Tschumperlin, D. J., Oswari, J., & Margulies, S. S. (2011). Mechanotransduction in the lung: how mechanical forces influence cellular processes. American Journal of Physiology-Lung Cellular and Molecular Physiology, 300(6), L889-L899.
[5] Hinz, B., Phan, S. H., Thannickal, V. J., Galli, A., Bochaton-Piallat, M. L., & Gabbiani, G. (2007). The myofibroblast: one function, multiple origins. American Journal of Pathology, 170(6), 1807-1816.
[6] Theocharis, A. D., Skandalis, S. S., Gialeli, C., & Karamanos, N. K. (2016). Extracellular matrix structure. Advanced Drug Delivery Reviews, 97, 4-27.
[7] Visse, R., & Nagase, H. (2003). Matrix metalloproteinases and tissue inhibitors of metalloproteinases: structure, function, and biochemistry. Circulation Research, 92(8), 827-839.
[8] Wynn, T. A., & Ramalingam, T. R. (2012). Mechanisms of fibrosis: parasite perspectives. Nature Reviews Immunology, 12(8), 551-563.
[9] Massagué, J. (2012). TGFβ signalling in control of cell behavior, cancer, and immunity. Cell, 134(2), 215-230.
[10] Clevers, H., & Nusse, R. (2012). Wnt/β-catenin signaling and disease. Cell, 149(6), 1192-1205.
[11] Katoh, Y., & Katoh, M. (2009). Hedgehog signaling pathway and gastrointestinal stem cell research. World Journal of Gastroenterology, 15(42), 5244-5251.
[12] Huang, W. J., Choi, Y. J., Yoon, H. E., & Hong, Y. A. (2015). Targeting the PI3K/Akt/mTOR signaling pathway in diabetic nephropathy. Kidney International, 88(6), 1189-1197.
[13] Kalluri, R., & Weinberg, R. A. (2009). The basics of epithelial-mesenchymal transition. Journal of Clinical Investigation, 119(6), 1420-1428.
[14] Nieto, M. A., Huang, R. Y. J., Jackson, R. A., & Thiery, J. P. (2016). Emt: 2016. Cell, 166(1), 21-45.
[15] Friedman, S. L. (2008). Mechanisms of hepatic fibrosis. Gastroenterology, 134(6), 1655-1669.
[16] Raghu, G., Collard, H. R., Egan, J. J., Martinez, F. J., Behr, J., Brown, K. K., … & du Bois, R. M. (2011). An official ATS/ERS/JRS/ALAT statement: idiopathic pulmonary fibrosis: evidence-based guidelines for diagnosis and management. American Journal of Respiratory and Critical Care Medicine, 183(6), 788-824.
[17] Meng, X. M., Tang, P. M., Li, J., Lan, H. Y. (2015). TGF-β/Smad signaling in renal fibrosis. Front Physiol. 6:82.
[18] Travers, J. G., Kamal, F. A., Robbins, N., Yutzey, K. E., & Blaxall, B. C. (2016). Cardiac Fibrosis: The Fibroblast Awakens. Circulation Research, 118(6), 1021-1040.
[19] Varga, J., & Abraham, D. (2007). Systemic sclerosis: a prototypic multisystem fibrotic disorder. Journal of Internal Medicine, 261(1), 1-17.
[20] Friedrich-Rust, M., Wunder, K., Kriener, S., Sotoudeh, F., Richter, J., Bojunga, J., … & Herrmann, E. (2009). Liver fibrosis in viral hepatitis: noninvasive assessment with acoustic radiation force impulse imaging versus transient elastography. Radiology, 252(2), 595-604.
[21] Lynch, D. A., Travis, W. D., Muller, N. L., Galvin, J. R., Brown, K. K., & Colby, T. V. (2018). Idiopathic pulmonary fibrosis: diagnosis and staging. American Journal of Respiratory and Critical Care Medicine, 198(4), 419-442.
[22] Banerjee, S., Gencturk, M., Motosugi, U., Machann, J., Horger, M., Schraml, C., … & Beer, M. (2015). Assessment of liver fibrosis with magnetic resonance elastography: a prospective multicenter study. Hepatology, 62(5), 1460-1470.
[23] Richeldi, L., du Bois, R. M., Behr, J., Crestani, B., Wuyts, W., Fischer, A., … & on behalf of the INPULSIS Trial Investigators. (2014). Efficacy and safety of nintedanib in idiopathic pulmonary fibrosis. New England Journal of Medicine, 370(22), 2071-2082.
[24] Akhurst, R. J., & Hata, A. (2012). Targeting the TGFβ signalling pathway for the treatment of fibrosis. Nature Reviews Drug Discovery, 11(10), 790-811.
[25] Henderson, W. R., Chi, E. Y., Li, H., Pan, T. Y., Link, J. M., Boyce, R., … & Wight, T. N. (2010). Inhibition of Wnt/β-catenin signaling ameliorates allergen-induced airway remodeling. American Journal of Respiratory and Critical Care Medicine, 182(3), 313-324.
[26] Akhmetshina, A., Dees, C., Busch, N., Beyer, C., Wermuth, P. J., Metze, D., … & Distler, O. (2009). The GLI1 transcription factor inhibits collagen expression in systemic sclerosis fibroblasts via direct interaction with Smad3. Arthritis & Rheumatism, 60(9), 2785-2794.
[27] Rockey, D. C., Bell, P. D., & Hill, J. A. (2015). Fibrosis–a common pathway to organ injury and failure. New England Journal of Medicine, 372(12), 1138-1149.
[28] Mantovani, A., Sica, A., Sozzani, S., Allavena, P., Vecchi, A., & Locati, M. (2004). The chemokine system in diverse forms of macrophage activation and polarization. Trends in Immunology, 25(12), 677-686.
[29] Parks, W. C., Wilson, C. L., & López-Boado, Y. S. (2004). Matrix metalloproteinases as modulators of inflammation and immunity. Nature Reviews Immunology, 4(8), 617-629.
[30] Barry-Hamilton, V., Spangler, R., Borges, E., Manjunath, S. H., Carter, D., Vallon, R., … & Hyde, D. M. (2010). Allosteric inhibition of lysyl oxidase-like 2 impedes the development of a fibrotic microenvironment. Nature Medicine, 16(9), 1009-1017.
This is a comprehensive overview of fibrosis. The discussion around macrophage polarization (M1 vs. M2) is particularly interesting. Emerging research suggests that targeting macrophage metabolism could be a novel therapeutic approach to re-polarize these cells and reduce fibrosis. What are your thoughts on this strategy?