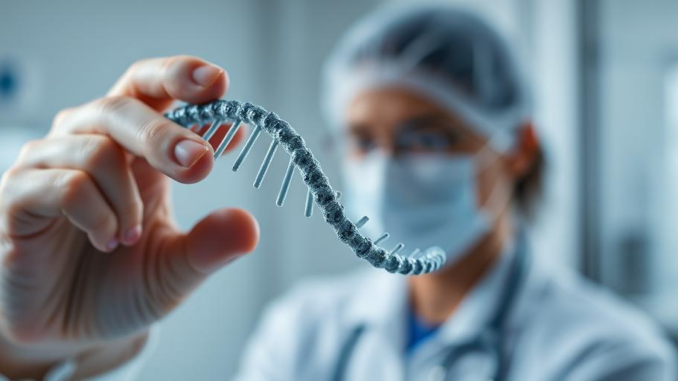
Abstract
Gene therapy, a revolutionary approach to treating diseases by modifying a patient’s genes, has rapidly evolved from theoretical possibility to clinical reality. This report provides a comprehensive overview of gene therapy, encompassing its underlying mechanisms, various delivery methods, success rates in diverse disease contexts beyond Maple Syrup Urine Disease (MSUD), potential risks and side effects, cost and accessibility challenges, and the complex ethical landscape it navigates. Furthermore, it delves into the regulatory pathways governing gene therapy development and commercialization, and explores the future trajectory of gene therapy as a mainstream therapeutic modality. The report aims to provide an expert-level analysis of the current state of gene therapy, highlighting both its transformative potential and the challenges that remain to be addressed.
Many thanks to our sponsor Esdebe who helped us prepare this research report.
1. Introduction
Gene therapy represents a paradigm shift in medicine, moving beyond symptomatic treatment to address the root cause of genetic disorders. By introducing, silencing, or modifying genes within a patient’s cells, gene therapy offers the promise of long-lasting or even curative effects. The increasing number of approved gene therapies and the expanding range of clinical trials underscore the growing momentum in this field. While the early years of gene therapy were marked by significant setbacks, advancements in vector design, gene editing technologies, and our understanding of the immune response have led to safer and more effective therapies.
This report aims to provide a detailed and critical analysis of gene therapy, examining its various facets, from the underlying scientific principles to the ethical implications and future prospects. It will build upon the context of MSUD as a disease that gene therapy can potentially treat and expand to other areas where gene therapy is used.
Many thanks to our sponsor Esdebe who helped us prepare this research report.
2. Types of Gene Therapy
Gene therapy can be broadly categorized into two main types based on the cells targeted: somatic gene therapy and germline gene therapy. A third category, ex vivo versus in vivo further refines the types of gene therapy that can be delivered.
2.1. Somatic Gene Therapy
Somatic gene therapy involves the transfer of genetic material into somatic cells, which are non-reproductive cells. This means that the therapeutic effect is limited to the individual receiving the treatment and is not passed on to future generations. Somatic gene therapy is the most common type of gene therapy currently being used in clinical trials and approved therapies.
2.2. Germline Gene Therapy
Germline gene therapy involves the introduction of genetic material into germ cells (sperm or eggs) or early embryos. This type of gene therapy would result in the genetic modification being passed on to future generations. Due to significant ethical concerns and technical challenges, germline gene therapy is currently not permitted for clinical use in most countries. The long-term consequences of altering the human germline are largely unknown, raising concerns about unintended effects on future generations.
2.3. Ex vivo vs. In vivo Gene Therapy
Another classification distinguishes between ex vivo and in vivo gene therapy. In ex vivo gene therapy, cells are modified genetically outside the body (e.g., in a laboratory) and then transplanted back into the patient. In vivo gene therapy involves the direct delivery of the therapeutic gene into the patient’s body, where it is taken up by the target cells. Each approach has its own advantages and disadvantages in terms of efficiency, safety, and feasibility for different diseases.
Many thanks to our sponsor Esdebe who helped us prepare this research report.
3. Gene Delivery Vectors
The efficient and safe delivery of therapeutic genes to target cells is a critical aspect of gene therapy. Several types of vectors are used for gene delivery, each with its own strengths and limitations.
3.1. Viral Vectors
Viral vectors are the most commonly used gene delivery vehicles due to their natural ability to infect cells and deliver genetic material. Different types of viruses are used as vectors, including:
- Adeno-associated viruses (AAVs): AAVs are small, non-pathogenic viruses that can infect a wide range of cell types. They are considered relatively safe and elicit a minimal immune response. AAVs can be engineered to carry therapeutic genes and are widely used in clinical trials and approved gene therapies.
- Adenoviruses: Adenoviruses are double-stranded DNA viruses that can infect both dividing and non-dividing cells. They are relatively easy to produce in large quantities but can elicit a stronger immune response compared to AAVs.
- Lentiviruses: Lentiviruses are retroviruses that can integrate their genetic material into the host cell’s genome, providing long-term gene expression. Lentiviruses are particularly useful for transducing hematopoietic stem cells and other cell types that require stable gene transfer.
- Herpes simplex viruses (HSVs): HSVs are large DNA viruses that can infect a wide range of cell types, including neurons. They are often used for gene therapy applications targeting the nervous system.
3.2. Non-Viral Vectors
Non-viral vectors offer an alternative to viral vectors and can overcome some of the limitations associated with viral delivery, such as immunogenicity and insertional mutagenesis. Common non-viral vectors include:
- Plasmid DNA: Plasmid DNA is a circular DNA molecule that can be engineered to carry therapeutic genes. It can be delivered into cells via various methods, such as electroporation, lipofection, or gene gun.
- Liposomes: Liposomes are spherical vesicles composed of lipid bilayers that can encapsulate therapeutic genes and deliver them to cells.
- Nanoparticles: Nanoparticles made of various materials, such as polymers or lipids, can be used to encapsulate and deliver therapeutic genes to target cells. Nanoparticles can be engineered to target specific cell types and improve gene delivery efficiency.
3.3. Vector Selection Considerations
The choice of vector depends on several factors, including the target cell type, the size of the therapeutic gene, the desired duration of gene expression, and the potential for immune response. Viral vectors generally offer higher transduction efficiency compared to non-viral vectors, but they also carry a higher risk of immunogenicity and insertional mutagenesis. Non-viral vectors are generally safer but less efficient in gene delivery.
Many thanks to our sponsor Esdebe who helped us prepare this research report.
4. Gene Editing Technologies
Gene editing technologies have revolutionized gene therapy by enabling precise and targeted modification of genes within the genome. These technologies allow for the correction of disease-causing mutations, the insertion of therapeutic genes, or the disruption of unwanted genes.
4.1. CRISPR-Cas9
CRISPR-Cas9 (Clustered Regularly Interspaced Short Palindromic Repeats and CRISPR-associated protein 9) is a revolutionary gene editing technology that has transformed the field of gene therapy. CRISPR-Cas9 uses a guide RNA molecule to direct the Cas9 enzyme to a specific DNA sequence in the genome. The Cas9 enzyme then cuts the DNA at the target site, allowing for the insertion, deletion, or modification of genes. CRISPR-Cas9 is relatively easy to use, highly efficient, and can be applied to a wide range of cell types and organisms. However, off-target effects, where the Cas9 enzyme cuts DNA at unintended sites, remain a concern.
4.2. Zinc Finger Nucleases (ZFNs) and Transcription Activator-Like Effector Nucleases (TALENs)
ZFNs and TALENs are earlier gene editing technologies that use engineered proteins to target specific DNA sequences and induce DNA cleavage. While less versatile and more complex to design than CRISPR-Cas9, ZFNs and TALENs offer greater specificity and reduced off-target effects in some applications.
4.3. Base Editing
Base editing is a more recent gene editing technology that allows for the precise conversion of one DNA base to another without creating double-strand breaks in the DNA. This approach reduces the risk of off-target effects and is particularly useful for correcting point mutations that cause genetic diseases.
4.4. Prime Editing
Prime editing is another advanced gene editing technology that can precisely insert, delete, or replace DNA sequences without requiring double-strand breaks or donor DNA templates. Prime editing offers greater precision and versatility compared to other gene editing methods.
Many thanks to our sponsor Esdebe who helped us prepare this research report.
5. Success Rates in Various Diseases
Gene therapy has shown promising results in treating a variety of diseases, including genetic disorders, cancer, and infectious diseases. Success rates vary depending on the disease, the gene therapy approach used, and the patient population.
5.1. Spinal Muscular Atrophy (SMA)
Zolgensma (onasemnogene abeparvovec) is an AAV-based gene therapy approved for the treatment of spinal muscular atrophy (SMA), a severe genetic disorder that causes muscle weakness and paralysis. Zolgensma delivers a functional copy of the SMN1 gene to motor neurons, improving muscle function and survival rates in infants with SMA. The therapy is given as a single intravenous infusion. Clinical trials have demonstrated significant improvements in motor function and survival rates in infants treated with Zolgensma. The efficacy is well-documented and the therapy is considered a major breakthrough.
5.2. Beta-Thalassemia
Zynteglo (betibeglogene autotemcel) is a lentiviral vector-based gene therapy approved for the treatment of beta-thalassemia, a genetic blood disorder that causes severe anemia. Zynteglo involves the transplantation of autologous hematopoietic stem cells that have been genetically modified to produce functional beta-globin. Clinical trials have shown that Zynteglo can reduce or eliminate the need for regular blood transfusions in patients with beta-thalassemia.
5.3. Severe Combined Immunodeficiency (SCID)
Gene therapy has been successful in treating certain forms of severe combined immunodeficiency (SCID), a group of genetic disorders that impair the immune system. For example, Strimvelis is a retroviral vector-based gene therapy approved for the treatment of adenosine deaminase-deficient SCID (ADA-SCID). Strimvelis involves the transplantation of autologous hematopoietic stem cells that have been genetically modified to express the ADA enzyme. Clinical trials have demonstrated that Strimvelis can restore immune function in children with ADA-SCID.
5.4. Leber’s Congenital Amaurosis (LCA)
Luxturna (voretigene neparvovec) is an AAV-based gene therapy approved for the treatment of Leber’s congenital amaurosis (LCA), a genetic eye disorder that causes severe vision loss. Luxturna delivers a functional copy of the RPE65 gene to retinal cells, improving vision in patients with LCA. Clinical trials have shown that Luxturna can significantly improve visual function in children and adults with LCA.
5.5. Cancer
CAR-T cell therapy, a type of gene therapy, has shown remarkable success in treating certain types of blood cancers, such as leukemia and lymphoma. CAR-T cell therapy involves the genetic modification of a patient’s T cells to express a chimeric antigen receptor (CAR) that targets specific cancer cells. The modified T cells are then infused back into the patient, where they can recognize and kill cancer cells. Examples include Kymriah and Yescarta. CAR-T therapy is typically reserved for patients who have failed other treatments.
Many thanks to our sponsor Esdebe who helped us prepare this research report.
6. Potential Risks and Side Effects
While gene therapy holds great promise, it is not without potential risks and side effects. The safety of gene therapy is a major concern, and researchers are working to minimize the risks associated with gene delivery and gene editing.
6.1. Immunogenicity
The immune system can recognize viral vectors and genetically modified cells as foreign and mount an immune response against them. This immune response can lead to inflammation, organ damage, and reduced efficacy of the gene therapy. Pre-existing immunity to viral vectors, especially AAV, is a significant challenge, as many individuals have been exposed to AAV in the past. Strategies to overcome immunogenicity include the use of immunosuppressants, the development of less immunogenic vectors, and the use of gene editing to delete or modify immune-related genes.
6.2. Insertional Mutagenesis
Viral vectors, particularly retroviruses, can insert their genetic material into the host cell’s genome in a random manner. This can lead to insertional mutagenesis, where the viral vector disrupts a critical gene or activates an oncogene, potentially leading to cancer. The risk of insertional mutagenesis can be minimized by using self-inactivating vectors and by targeting gene insertion to specific locations in the genome using gene editing technologies.
6.3. Off-Target Effects
Gene editing technologies, such as CRISPR-Cas9, can sometimes cut DNA at unintended sites in the genome, leading to off-target effects. Off-target effects can cause unintended mutations, potentially leading to adverse effects. The risk of off-target effects can be minimized by using highly specific guide RNAs, optimizing Cas9 enzyme activity, and using advanced gene editing technologies, such as base editing and prime editing.
6.4. Toxicity
Some gene therapy vectors and gene editing components can be toxic to cells. Toxicity can lead to cell death and organ damage. The risk of toxicity can be minimized by using non-toxic vectors and by optimizing the dosage and delivery method of gene therapy.
6.5. Germline Transmission (Germline Gene Therapy)
As stated before, germline gene therapy carries the risk of transmitting genetic modifications to future generations. The effects of such modifications are largely unknown and could have unintended consequences. Most regulatory bodies prohibit germline gene therapy due to ethical concerns.
Many thanks to our sponsor Esdebe who helped us prepare this research report.
7. Cost and Accessibility
Gene therapies are often very expensive, due to the complex manufacturing processes, extensive clinical trials, and personalized nature of the treatments. The high cost of gene therapy raises concerns about accessibility and equity, as many patients may not be able to afford these potentially life-saving treatments. For example, Zolgensma is one of the most expensive drugs in the world.
7.1. Pricing Models
Various pricing models are being explored to address the cost and accessibility challenges of gene therapy, including:
- Value-based pricing: This model ties the price of the gene therapy to its clinical value, based on the outcomes achieved in patients. This approach requires robust data collection and analysis to accurately assess the value of the therapy.
- Installment payments: This model allows payers to spread the cost of the gene therapy over several years, reducing the financial burden on patients and healthcare systems.
- Outcome-based reimbursement: This model ties reimbursement to the achievement of pre-defined clinical outcomes. If the gene therapy fails to achieve the desired outcomes, the payer may not reimburse the full cost of the treatment.
- Government subsidies and public funding: Government subsidies and public funding can help to reduce the cost of gene therapy and improve access for patients.
7.2. Access Barriers
In addition to cost, other factors can limit access to gene therapy, including:
- Geographic location: Gene therapy is often only available at specialized treatment centers, which may be located in major cities or developed countries. This can create geographic barriers for patients who live in rural areas or developing countries.
- Insurance coverage: Insurance coverage for gene therapy can be limited or variable, depending on the insurance plan and the specific gene therapy. Patients may need to navigate complex insurance approval processes to access these treatments.
- Patient awareness: Many patients are not aware of the availability of gene therapy or its potential benefits. Increased patient awareness and education are needed to improve access to these treatments.
Many thanks to our sponsor Esdebe who helped us prepare this research report.
8. Ethical Considerations
Gene therapy raises a number of complex ethical considerations, including:
8.1. Safety and Risk
The safety of gene therapy is a paramount concern, and researchers must carefully weigh the potential benefits of gene therapy against the potential risks and side effects. Patients must be fully informed about the risks and benefits of gene therapy before making a decision about treatment.
8.2. Equity and Access
The high cost of gene therapy raises concerns about equity and access. Gene therapy should be available to all patients who could benefit from it, regardless of their socioeconomic status or geographic location. Strategies are needed to ensure that gene therapy is affordable and accessible to all.
8.3. Enhancement vs. Therapy
Gene therapy is primarily intended for the treatment of diseases, but it could also be used for enhancement purposes, such as improving athletic performance or cognitive abilities. The use of gene therapy for enhancement raises ethical concerns about fairness, social justice, and the potential for unintended consequences.
8.4. Germline Modification
Germline gene therapy raises profound ethical concerns about the potential for unintended consequences in future generations. Most regulatory bodies prohibit germline gene therapy due to these concerns. The long-term effects of altering the human germline are largely unknown, raising concerns about the potential for unforeseen harm.
8.5. Informed Consent
Patients must provide informed consent before undergoing gene therapy. Informed consent requires that patients understand the risks and benefits of gene therapy, the alternatives to gene therapy, and the potential for long-term consequences.
Many thanks to our sponsor Esdebe who helped us prepare this research report.
9. Regulatory Pathways
The development and commercialization of gene therapies are subject to strict regulatory oversight by government agencies, such as the Food and Drug Administration (FDA) in the United States and the European Medicines Agency (EMA) in Europe. These agencies evaluate the safety and efficacy of gene therapies before they can be approved for clinical use.
9.1. Clinical Trials
Gene therapies must undergo rigorous clinical trials to demonstrate their safety and efficacy. Clinical trials are typically conducted in phases, starting with Phase 1 trials to assess safety and dosage, followed by Phase 2 trials to assess efficacy and side effects, and finally Phase 3 trials to confirm efficacy and monitor long-term safety. The success of clinical trials is crucial for regulatory approval.
9.2. FDA Approval Process (US)
The FDA reviews data from clinical trials to determine whether a gene therapy is safe and effective for its intended use. If the FDA determines that the benefits of the gene therapy outweigh the risks, it may approve the therapy for marketing. The FDA also monitors the safety and efficacy of gene therapies after they have been approved for marketing.
9.3. EMA Approval Process (Europe)
The EMA reviews data from clinical trials to determine whether a gene therapy is safe and effective for its intended use in Europe. If the EMA determines that the benefits of the gene therapy outweigh the risks, it may grant a marketing authorization for the therapy. The EMA also monitors the safety and efficacy of gene therapies after they have been authorized for marketing.
Many thanks to our sponsor Esdebe who helped us prepare this research report.
10. Future Directions
The field of gene therapy is rapidly evolving, with ongoing research focused on improving the safety, efficacy, and accessibility of these treatments. Several promising areas of research include:
10.1. Improved Vector Design
Researchers are working to develop new and improved viral and non-viral vectors that are more efficient, less immunogenic, and more targeted to specific cell types. This includes engineering AAV capsids with improved tropism and reduced off-target effects, as well as developing novel non-viral delivery systems that can efficiently deliver genes to target cells.
10.2. Enhanced Gene Editing Technologies
Continued development of gene editing technologies, such as CRISPR-Cas9, base editing, and prime editing, is focused on improving their precision, reducing off-target effects, and expanding their applications. This includes developing more sophisticated guide RNA design tools, optimizing Cas9 enzyme activity, and developing new gene editing enzymes with improved specificity.
10.3. Personalized Gene Therapy
Advances in genomics and personalized medicine are paving the way for personalized gene therapy approaches, where treatments are tailored to the individual patient’s genetic makeup and disease characteristics. This includes developing gene therapies that target specific mutations or gene expression patterns in individual patients.
10.4. Expanding Disease Applications
Gene therapy is being explored for the treatment of a growing number of diseases, including neurological disorders, cardiovascular diseases, and autoimmune diseases. Clinical trials are underway to evaluate the safety and efficacy of gene therapy in these new disease areas.
10.5. Combination Therapies
Gene therapy is increasingly being combined with other therapies, such as immunotherapy and chemotherapy, to improve treatment outcomes. These combination therapies aim to harness the synergistic effects of different treatment modalities to achieve greater efficacy.
Many thanks to our sponsor Esdebe who helped us prepare this research report.
11. Conclusion
Gene therapy represents a transformative approach to treating diseases by addressing the underlying genetic causes. While significant progress has been made in recent years, challenges remain in terms of safety, efficacy, cost, and ethical considerations. Ongoing research and development efforts are focused on improving vector design, enhancing gene editing technologies, and expanding the applications of gene therapy to a wider range of diseases. As gene therapy continues to evolve, it holds the potential to revolutionize the treatment of many diseases and improve the lives of countless patients. The future of gene therapy hinges on addressing the remaining challenges and ensuring that these potentially life-saving treatments are accessible to all who could benefit from them.
Many thanks to our sponsor Esdebe who helped us prepare this research report.
References
- Anderson, W. F. (1998). Human gene therapy. Nature, 392(6679 Suppl), 25-30.
- Cavazzana-Calvo, M., Hacein-Bey-Abina, S., Fischer, A. (2005). Gene therapy for severe combined immunodeficiency: where do we stand?. The Journal of Gene Medicine, 7(5), 505-517.
- Doudna, J. A., & Charpentier, E. (2014). Genome editing. Science, 346(6213), 1258096.
- High, K. A., & Roncarolo, M. G. (2019). Gene therapy. New England Journal of Medicine, 381(5), 455-464.
- Keeler, A. M., & Flotte, T. R. (2019). Recombinant adeno-associated virus gene therapy in inherited retinal disease. Human Molecular Genetics, 28(R2), R141-R149.
- Lusis, E., & Stein, D. (2020). The current landscape of gene therapies in the United States. Journal of Managed Care & Specialty Pharmacy, 26(9), 1100-1107.
- Naldini, L. (2015). Gene therapy returns to centre stage. Nature, 526(7573), 351-360.
- National Human Genome Research Institute. (n.d.). Gene Therapy. Retrieved from https://www.genome.gov/genetics-glossary/Gene-Therapy
- Samaranch, L., & Bankiewicz, K. S. (2020). Clinical gene therapy for neurodegenerative disorders. Lancet Neurology, 19(12), 1029-1042.
- Walters, L., & Palmer, A. (1997). The ethics of human gene therapy. Nature Genetics, 15(1), 5-8.
- Ylä-Herttuala, S. (2012). Endgame: gene therapy for cardiovascular disease. European Heart Journal, 33(14), 1764-1772.
Germline modification? Sounds like we’re playing God, but with slightly better safety regulations. What happens when someone decides enhanced intelligence or athleticism becomes a “right,” not a privilege?