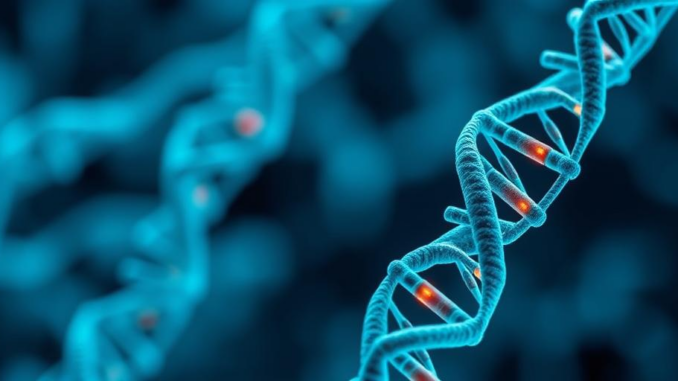
Genomics: Revolutionizing Medicine Through Personalized Insights and Precision Interventions
Many thanks to our sponsor Esdebe who helped us prepare this research report.
Abstract
Genomics, the study of an organism’s entire genome, has transformed biological research and medical practice. This report delves into the multifaceted advancements in genomics, encompassing sequencing technologies, ethical considerations, diverse disease applications, and the evolving regulatory environment. We explore the progression of sequencing from Sanger sequencing to high-throughput next-generation sequencing (NGS) and the latest long-read sequencing techniques. Furthermore, the ethical dimensions surrounding genomic data, including privacy, security, and potential for discrimination, are critically examined. This report also discusses the applications of genomics in infectious diseases and personalized medicine, including cancer, rare diseases, and pharmacogenomics. CRISPR-Cas9 gene editing is explored with its transformative potential alongside the ethical and safety considerations. Finally, we address the evolving regulatory landscape governing genomic research, diagnostic testing, and therapeutic interventions, highlighting the challenges and opportunities in ensuring responsible innovation and equitable access. This comprehensive overview aims to provide expert insights into the current state and future trajectory of genomics in shaping healthcare.
Many thanks to our sponsor Esdebe who helped us prepare this research report.
1. Introduction
The completion of the Human Genome Project (HGP) in 2003 marked a pivotal moment in the history of science, ushering in the era of genomics. Since then, rapid technological advancements, particularly in DNA sequencing technologies, have dramatically reduced the cost and increased the speed of genome analysis. This has fueled an exponential growth in genomic data, enabling unprecedented insights into the fundamental mechanisms of life, disease pathogenesis, and individual responses to therapeutic interventions. Genomics is now impacting virtually every facet of biomedical research, from basic biological discovery to clinical diagnostics and personalized medicine. The scope of genomics extends beyond simply sequencing the genome; it encompasses the study of gene structure, function, interactions, evolution, and their impact on phenotype. This report aims to provide a comprehensive overview of genomics, encompassing its technological advancements, ethical implications, disease applications, and the regulatory landscape, offering expert insights into this rapidly evolving field.
Many thanks to our sponsor Esdebe who helped us prepare this research report.
2. Advancements in Genomic Sequencing Technologies
The revolution in genomics has been primarily driven by the evolution of sequencing technologies. The journey began with Sanger sequencing, a laborious and time-consuming method that served as the workhorse of the HGP. While highly accurate, Sanger sequencing was not suitable for large-scale genomic studies. The advent of next-generation sequencing (NGS) technologies, such as Illumina sequencing, dramatically increased throughput, reduced cost, and accelerated the pace of genomic research. NGS platforms offer massively parallel sequencing, allowing for the simultaneous sequencing of millions of DNA fragments. This enabled whole-genome sequencing (WGS), whole-exome sequencing (WES), and targeted sequencing approaches to become routine procedures in research and clinical settings.
2.1 Short-Read Sequencing (NGS)
Short-read sequencing, exemplified by Illumina platforms, excels in its high accuracy and cost-effectiveness. It has become the standard for applications like variant calling, RNA sequencing (RNA-Seq), and chromatin immunoprecipitation sequencing (ChIP-Seq). However, short reads (typically 150-300 base pairs) pose challenges in resolving complex genomic regions, such as repetitive sequences and structural variants. These regions are often critical for understanding disease etiology and phenotypic diversity.
2.2 Long-Read Sequencing
To overcome the limitations of short-read sequencing, long-read sequencing technologies, such as those offered by Pacific Biosciences (PacBio) and Oxford Nanopore Technologies (ONT), have emerged. PacBio’s Single Molecule Real-Time (SMRT) sequencing can generate reads tens of thousands of base pairs long, while ONT’s nanopore sequencing can produce reads exceeding megabases in length. Long-read sequencing enables the accurate characterization of repetitive regions, structural variants, and haplotype phasing, providing a more complete and nuanced understanding of the genome. However, long-read sequencing technologies typically have higher error rates compared to short-read sequencing, although error rates are falling rapidly and can be mitigated through circular consensus sequencing and advanced algorithms. Hybrid approaches, combining short-read and long-read sequencing, are increasingly used to leverage the strengths of both technologies, providing high accuracy and comprehensive genomic information.
2.3 Single-Cell Genomics
Single-cell genomics has revolutionized our understanding of cellular heterogeneity in tissues and organisms. Single-cell sequencing technologies allow for the analysis of DNA, RNA, and proteins at the individual cell level, revealing insights into cell lineage, differentiation, and functional states. This has profound implications for understanding development, immunity, and disease, particularly in cancer, where tumor heterogeneity plays a critical role in treatment response and resistance.
Many thanks to our sponsor Esdebe who helped us prepare this research report.
3. Ethical Considerations Surrounding Genomic Data Usage
The increasing availability and accessibility of genomic data raise significant ethical considerations that must be addressed to ensure responsible innovation and protect individual rights. These ethical concerns encompass privacy, security, informed consent, potential for discrimination, and equitable access.
3.1 Privacy and Security
Genomic data is highly personal and sensitive information that can reveal not only an individual’s health status but also their ancestry and familial relationships. Maintaining the privacy and security of genomic data is paramount. Data breaches and unauthorized access can lead to discrimination, stigmatization, and psychological distress. Robust security measures, including encryption, access controls, and data anonymization techniques, are essential to protect genomic data from unauthorized use. However, anonymization alone is not sufficient, as re-identification risks exist, especially with the increasing availability of public genomic databases.
3.2 Informed Consent
Obtaining informed consent from individuals participating in genomic research or undergoing clinical genomic testing is crucial. Informed consent requires that individuals understand the purpose, risks, and benefits of genomic analysis, as well as their rights regarding data storage, sharing, and future use. The complexity of genomic information and its potential implications for individuals and their families necessitate clear and comprehensive communication between researchers, clinicians, and patients. Furthermore, the dynamic nature of genomic research requires that consent forms be updated periodically to reflect new findings and evolving practices.
3.3 Potential for Discrimination
Genomic information can be used to discriminate against individuals based on their genetic predispositions. This can occur in various contexts, including employment, insurance, and access to healthcare. The Genetic Information Nondiscrimination Act (GINA) in the United States prohibits genetic discrimination in employment and health insurance. However, GINA does not cover life insurance, disability insurance, or long-term care insurance, leaving individuals vulnerable to potential discrimination in these areas. The potential for genetic discrimination underscores the need for comprehensive legal and ethical frameworks to protect individuals’ rights and prevent unfair treatment.
3.4 Equitable Access
The benefits of genomics should be accessible to all individuals, regardless of their socioeconomic status, ethnicity, or geographic location. However, access to genomic technologies and expertise remains unevenly distributed, creating disparities in healthcare outcomes. Efforts are needed to promote equitable access to genomic testing and personalized medicine, particularly in underserved communities. This requires addressing issues such as cost, infrastructure, workforce training, and cultural sensitivity.
Many thanks to our sponsor Esdebe who helped us prepare this research report.
4. Applications of Genomics in Diverse Diseases
Genomics has revolutionized our understanding of the genetic basis of a wide range of diseases, from infectious diseases to cancer and rare genetic disorders. Genomic approaches are being used to identify disease-causing genes, develop diagnostic tests, predict disease risk, and personalize treatment strategies.
4.1 Infectious Diseases
Genomics plays a critical role in the diagnosis, surveillance, and control of infectious diseases. Whole-genome sequencing of pathogens, such as bacteria, viruses, and fungi, enables the rapid identification of infectious agents, the tracking of outbreaks, and the monitoring of antimicrobial resistance. Genomic data can be used to develop diagnostic tests, identify drug targets, and design vaccines. During the COVID-19 pandemic, genomics played a pivotal role in identifying the SARS-CoV-2 virus, tracking its evolution, and developing vaccines and therapeutics. Moreover, genomic studies of host-pathogen interactions are providing insights into disease susceptibility and severity.
4.2 Cancer
Cancer is a genetic disease driven by the accumulation of somatic mutations in genes that regulate cell growth, differentiation, and apoptosis. Genomics has transformed cancer research and clinical oncology. Whole-genome sequencing and whole-exome sequencing of tumors have revealed the complex landscape of cancer genomes, identifying driver genes and signaling pathways that are dysregulated in different cancer types. Genomic profiling of tumors is now used to guide treatment decisions, predict prognosis, and monitor response to therapy. Furthermore, genomics is facilitating the development of targeted therapies that specifically target cancer-driving mutations, as well as immunotherapies that harness the power of the immune system to fight cancer.
4.3 Rare Diseases
Rare diseases, which collectively affect millions of people worldwide, are often caused by single-gene mutations. Genomics has revolutionized the diagnosis of rare diseases. Whole-exome sequencing and whole-genome sequencing can be used to identify the causative genes in patients with unexplained medical conditions. This can lead to a definitive diagnosis, inform prognosis, and guide treatment. In many cases, a genomic diagnosis can end a diagnostic odyssey, reducing anxiety and improving quality of life for patients and their families. However, the interpretation of genomic data in the context of rare diseases can be challenging, requiring expertise in genetics, bioinformatics, and clinical medicine.
4.4 Pharmacogenomics
Pharmacogenomics, the study of how genes affect a person’s response to drugs, is a key area of personalized medicine. Genetic variations can influence drug metabolism, drug transport, and drug target interactions, leading to differences in drug efficacy and toxicity. Pharmacogenomic testing can be used to identify individuals who are likely to benefit from a particular drug, as well as those who are at increased risk of adverse drug reactions. This information can be used to personalize drug selection and dosing, optimizing treatment outcomes and minimizing side effects. Pharmacogenomics is particularly relevant in areas such as oncology, cardiology, and psychiatry, where drug response variability is high.
Many thanks to our sponsor Esdebe who helped us prepare this research report.
5. CRISPR-Cas9 Gene Editing
CRISPR-Cas9 (Clustered Regularly Interspaced Short Palindromic Repeats and CRISPR-associated protein 9) is a revolutionary gene-editing technology that allows for precise and efficient modification of DNA sequences. The CRISPR-Cas9 system consists of a Cas9 enzyme, which acts as a molecular scissor, and a guide RNA, which directs the Cas9 enzyme to a specific DNA sequence. The Cas9 enzyme cuts the DNA at the target site, and the cell’s repair mechanisms can then be used to either disrupt the gene or insert a new DNA sequence.
5.1 Applications of CRISPR-Cas9
CRISPR-Cas9 has a wide range of applications in basic research, drug discovery, and gene therapy. In basic research, CRISPR-Cas9 is used to study gene function, model diseases, and develop new research tools. In drug discovery, CRISPR-Cas9 is used to identify drug targets and develop new therapies. In gene therapy, CRISPR-Cas9 is used to correct disease-causing mutations in patients with genetic disorders. Clinical trials using CRISPR-Cas9 are underway for a variety of diseases, including sickle cell anemia, beta-thalassemia, and cancer.
5.2 Ethical and Safety Considerations
CRISPR-Cas9 raises significant ethical and safety considerations. One concern is the potential for off-target effects, where the Cas9 enzyme cuts DNA at unintended sites, leading to unintended mutations. Efforts are underway to improve the specificity of CRISPR-Cas9 and minimize off-target effects. Another concern is the ethical implications of germline editing, where CRISPR-Cas9 is used to modify the DNA of reproductive cells, leading to heritable changes. Germline editing raises concerns about unintended consequences for future generations and the potential for misuse. There is a broad consensus that germline editing should only be considered for serious genetic diseases where there are no other treatment options, and only after careful ethical review and public discussion. Furthermore, questions regarding equitable access and the potential exacerbation of existing health disparities must be addressed.
Many thanks to our sponsor Esdebe who helped us prepare this research report.
6. Evolving Regulatory Landscape
The rapid advancements in genomics necessitate an evolving regulatory landscape to ensure responsible innovation, protect patient safety, and promote equitable access. Regulations governing genomic research, diagnostic testing, and therapeutic interventions vary across countries and jurisdictions.
6.1 Genomic Research
Genomic research is typically subject to ethical review by institutional review boards (IRBs) to ensure that research protocols are ethically sound and protect the rights and welfare of research participants. Regulations also govern the storage, sharing, and use of genomic data, with a focus on protecting privacy and security. Many countries have implemented data protection laws that apply to genomic data, such as the General Data Protection Regulation (GDPR) in the European Union.
6.2 Diagnostic Testing
Genomic diagnostic tests, such as those used for prenatal screening, carrier testing, and disease diagnosis, are subject to regulatory oversight to ensure their accuracy, reliability, and clinical utility. In the United States, the Food and Drug Administration (FDA) regulates in vitro diagnostic devices, including genomic tests. The FDA requires that diagnostic tests meet certain standards for analytical validity, clinical validity, and clinical utility. However, the regulation of laboratory-developed tests (LDTs), which are developed and performed within a single laboratory, has been a subject of debate. The FDA has proposed a framework for regulating LDTs, but the implementation of this framework remains uncertain.
6.3 Therapeutic Interventions
Genomic-based therapeutic interventions, such as gene therapies and targeted therapies, are subject to rigorous regulatory review to ensure their safety and efficacy. The FDA regulates gene therapies as biological products and requires that they undergo extensive preclinical and clinical testing before they can be approved for marketing. The regulatory pathway for gene therapies is complex and can be lengthy, but the FDA has taken steps to expedite the review process for promising therapies. Similarly, targeted therapies that target specific genetic mutations in cancer cells are subject to regulatory review to ensure their safety and efficacy. Furthermore, regulatory bodies are grappling with the challenge of ensuring the affordability and accessibility of genomic-based therapeutic interventions.
Many thanks to our sponsor Esdebe who helped us prepare this research report.
7. Conclusion
Genomics has revolutionized medicine and is poised to transform healthcare in the years to come. Advancements in sequencing technologies, coupled with sophisticated bioinformatics tools, are enabling unprecedented insights into the genetic basis of disease and individual responses to therapy. Ethical considerations surrounding genomic data usage must be carefully addressed to ensure responsible innovation and protect individual rights. The applications of genomics in infectious diseases, cancer, rare diseases, and pharmacogenomics are expanding rapidly, leading to improved diagnosis, treatment, and prevention. CRISPR-Cas9 gene editing holds tremendous promise for correcting disease-causing mutations, but its ethical and safety implications must be carefully considered. The evolving regulatory landscape must adapt to the rapid pace of genomic innovation to ensure patient safety, promote equitable access, and foster responsible development. As genomics continues to evolve, it is essential to foster collaboration between researchers, clinicians, policymakers, and the public to realize its full potential to improve human health.
Many thanks to our sponsor Esdebe who helped us prepare this research report.
References
- Collins, F. S., Varmus, H., & the NIH Directors. (2015). A new initiative on precision medicine. New England Journal of Medicine, 372(9), 793-795.
- Lander, E. S., Linton, L. M., Birren, B., et al. (2001). Initial sequencing and analysis of the human genome. Nature, 409(6822), 860-921.
- Goodwin, S., McPherson, J. D., & McCombie, W. R. (2016). Coming of age: ten years of next-generation sequencing technologies. Nature Reviews Genetics, 17(6), 333-351.
- Rhoads, A., & Au, K. F. (2015). PacBio sequencing and its applications. Genomics, Proteomics & Bioinformatics, 13(5), 278-289.
- Deamer, D., Akeson, M., & Branton, D. (2016). Nanopores and nucleic acids: prospects for ultrarapid sequencing. Nature Biotechnology, 34(1), 34-44.
- Hwang, T. J., Balayan, S. N., Benjamin, R. M., et al. (2019). Genetic Information Nondiscrimination Act (GINA): Implications for genomic research. Journal of Law and the Biosciences, 6(1), 1-14.
- Ledford, H. (2020). How COVID unlocked the power of genomics. Nature, 588(7836), 26-29.
- Stratton, M. R., Campbell, P. J., & Futreal, P. A. (2009). The cancer genome. Nature, 458(7239), 719-724.
- Landrum, M. J., Lee, J. M., Riley, G. R., et al. (2018). ClinVar: improving access to variant interpretations and supporting evidence. Nucleic Acids Research, 46(D1), D1062-D1067.
- Doudna, J. A., & Charpentier, E. (2014). The new frontier of genome engineering with CRISPR-Cas9. Science, 346(6213), 1258096.
- Baltimore, D., Berg, P., Botchan, M., et al. (2015). A prudent path forward for genomic engineering and germline gene modification. Science, 348(6230), 36-38.
- Regalado, A. (2017). China’s CRISPR twins. MIT Technology Review.
- Phillips, K. A., Annas, G. J., & Elias, S. (2018). FDA regulation of laboratory-developed tests: time for a change. JAMA, 319(3), 229-230.
- Nathwani, A. C., Gray, J. T., Ng, C. Y., et al. (2014). Self-complementary adeno-associated virus vector-mediated gene transfer in hemophilia B. New England Journal of Medicine, 370(18), 1719-1728.
This report highlights the exciting potential of genomics in personalizing medicine. The section on pharmacogenomics, tailoring drug selection based on individual genetic profiles, is particularly promising for improving treatment outcomes and minimizing adverse reactions. How might advancements in AI further refine these personalized approaches?
Thanks for your insightful comment! The integration of AI with pharmacogenomics has huge potential. AI algorithms could analyze vast datasets to predict individual drug responses with greater accuracy, potentially identifying novel biomarkers and optimizing dosage recommendations. This could significantly reduce trial and error in medication management.
Editor: MedTechNews.Uk
Thank you to our Sponsor Esdebe