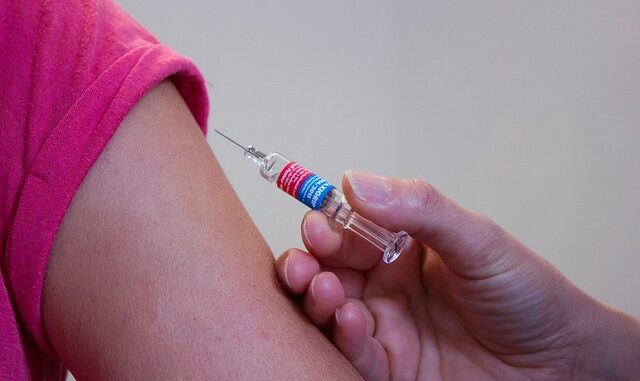
Abstract
Guillain-Barré Syndrome (GBS) is an acute, immune-mediated polyradiculoneuropathy characterized by progressive symmetrical weakness and areflexia. While often post-infectious, GBS has also been linked to certain vaccinations, raising concerns about vaccine safety. This report provides a comprehensive review of GBS, encompassing its etiopathogenesis, clinical presentation, diagnostic approaches, treatment strategies, and long-term management. We delve into the molecular mimicry hypothesis, exploring the role of specific gangliosides and antiganglioside antibodies in GBS pathogenesis. Furthermore, we critically examine the evidence linking GBS to vaccination, particularly in the context of respiratory syncytial virus (RSV) vaccines and other immunizations. We also compare GBS to other neurological disorders with overlapping symptoms, highlighting the importance of differential diagnosis. Finally, we discuss ongoing research efforts focused on understanding the intricate immunological mechanisms driving GBS and the development of novel therapeutic interventions aimed at improving patient outcomes.
Many thanks to our sponsor Esdebe who helped us prepare this research report.
1. Introduction
Guillain-Barré Syndrome (GBS) represents a significant neurological challenge, impacting individuals across all age groups and geographical locations. This autoimmune disorder primarily targets the peripheral nervous system, leading to rapidly progressive weakness, sensory disturbances, and, in severe cases, respiratory failure. The annual incidence of GBS is estimated to range from 0.4 to 4 per 100,000 individuals, with incidence increasing with age. While the exact etiology of GBS remains elusive, it is frequently associated with antecedent infections, most notably Campylobacter jejuni, cytomegalovirus (CMV), Epstein-Barr virus (EBV), and Mycoplasma pneumoniae. The syndrome’s association with infections points toward an immune-mediated pathogenesis, where the host’s immune response, intended to combat the initial infection, inadvertently cross-reacts with nerve tissue, leading to demyelination and axonal damage.
In recent years, concerns have been raised regarding a potential link between GBS and certain vaccinations. This association, though relatively rare, has been documented in connection with influenza vaccines, meningococcal vaccines, and, most recently, potential implications following RSV vaccination. The possibility of vaccine-associated GBS underscores the importance of rigorous post-market surveillance and ongoing research to identify potential risk factors and understand the underlying mechanisms. Addressing these concerns is crucial for maintaining public confidence in vaccination programs and ensuring that the benefits of immunization outweigh the potential risks. This report aims to provide a comprehensive overview of GBS, exploring its pathogenesis, clinical features, diagnostic modalities, treatment options, and the complex interplay between GBS and vaccination.
Many thanks to our sponsor Esdebe who helped us prepare this research report.
2. Etiopathogenesis of Guillain-Barré Syndrome
The prevailing hypothesis regarding GBS pathogenesis centers on molecular mimicry. This theory posits that microbial antigens, such as lipopolysaccharides (LPS) from Campylobacter jejuni, share structural similarities with gangliosides, which are glycosphingolipids present in the myelin sheath and axonal membranes of peripheral nerves. This structural homology triggers an immune response, generating antibodies that not only target the infecting organism but also cross-react with the host’s own nerve tissue.
2.1 Molecular Mimicry and Gangliosides
Gangliosides, including GM1, GD1a, GQ1b, and others, are critical components of the nerve membrane. Specific serotypes of Campylobacter jejuni, particularly those expressing lipooligosaccharides (LOS) with structures mimicking these gangliosides, have been strongly implicated in the development of GBS. For example, patients with acute motor axonal neuropathy (AMAN), a subtype of GBS, often exhibit antibodies against GM1 and GD1a gangliosides. Similarly, the Miller Fisher syndrome (MFS) variant of GBS, characterized by ophthalmoplegia, ataxia, and areflexia, is strongly associated with antibodies against GQ1b ganglioside.
The precise mechanisms by which antiganglioside antibodies cause nerve damage are still under investigation. Several pathways are thought to be involved, including:
- Complement activation: Antiganglioside antibodies can activate the complement cascade, leading to the formation of the membrane attack complex (MAC) and subsequent lysis of nerve cells.
- Antibody-dependent cell-mediated cytotoxicity (ADCC): Antiganglioside antibodies can bind to nerve cells and recruit immune cells, such as natural killer (NK) cells, to mediate cytotoxicity.
- Direct antibody-mediated damage: Antiganglioside antibodies may directly interfere with the function of ion channels or other membrane proteins, disrupting nerve conduction.
2.2 T-Cell Involvement
While antiganglioside antibodies play a central role in GBS pathogenesis, T cells also contribute to the immune-mediated nerve damage. Activated T cells, particularly CD4+ helper T cells, infiltrate the peripheral nerves and release cytokines, such as interferon-gamma (IFN-γ) and tumor necrosis factor-alpha (TNF-α), which promote inflammation and demyelination. Furthermore, CD8+ cytotoxic T cells can directly kill nerve cells expressing MHC class I molecules.
2.3 The Role of Cytokines and Chemokines
The inflammatory milieu within the peripheral nerves is further amplified by the release of various cytokines and chemokines. These signaling molecules recruit additional immune cells to the site of injury, perpetuating the inflammatory response. Key cytokines implicated in GBS pathogenesis include IL-1β, IL-6, IL-10, and IL-17. Chemokines, such as CCL2 and CXCL10, play a crucial role in attracting monocytes and T cells to the peripheral nerves.
2.4 Genetic Predisposition
While the majority of GBS cases occur sporadically, genetic factors may influence an individual’s susceptibility to developing the syndrome. Certain human leukocyte antigen (HLA) alleles, particularly HLA-B35 and HLA-DRB103, have been associated with an increased risk of GBS. Furthermore, polymorphisms in genes encoding cytokines, such as TNF-α, have also been linked to GBS susceptibility. Further research is needed to fully elucidate the role of genetic factors in GBS pathogenesis.
Many thanks to our sponsor Esdebe who helped us prepare this research report.
3. Clinical Presentation and Diagnosis
The hallmark of GBS is rapidly progressive, symmetrical weakness, typically beginning in the lower extremities and ascending upwards. The weakness often reaches its nadir within two to four weeks. Sensory symptoms, such as paresthesias (tingling or numbness), are also common, although they are usually less prominent than motor deficits. Areflexia or hyporeflexia is a characteristic finding.
3.1 Clinical Variants
GBS encompasses several clinical variants, each with distinct features:
- Acute Inflammatory Demyelinating Polyradiculoneuropathy (AIDP): The most common subtype, accounting for approximately 85% of cases in Western countries. AIDP is characterized by demyelination of peripheral nerves and slowed nerve conduction velocities.
- Acute Motor Axonal Neuropathy (AMAN): More prevalent in Asia and South America. AMAN involves primary axonal damage without significant demyelination. Patients with AMAN often have antibodies against GM1 and GD1a gangliosides.
- Acute Motor and Sensory Axonal Neuropathy (AMSAN): Similar to AMAN, but with both motor and sensory involvement. AMSAN is associated with more severe axonal damage and a poorer prognosis.
- Miller Fisher Syndrome (MFS): Characterized by the triad of ophthalmoplegia, ataxia, and areflexia. MFS is strongly associated with antibodies against GQ1b ganglioside.
3.2 Diagnostic Criteria
The diagnosis of GBS is based on a combination of clinical features, electrophysiological studies, and cerebrospinal fluid (CSF) analysis.
- Clinical criteria: Progressive, symmetrical weakness of the limbs, areflexia or hyporeflexia, and a relatively short interval between onset and nadir of symptoms are essential for diagnosis.
- Electrophysiological studies: Nerve conduction studies (NCS) are crucial for confirming the diagnosis and differentiating between demyelinating and axonal subtypes of GBS. Findings consistent with demyelination include prolonged distal latencies, slowed nerve conduction velocities, and conduction block. In axonal subtypes, NCS may show reduced compound muscle action potential (CMAP) amplitudes without significant slowing of conduction velocities.
- Cerebrospinal fluid (CSF) analysis: A characteristic finding in GBS is albuminocytologic dissociation, which refers to an elevated protein level in the CSF with a normal or only mildly elevated cell count. This finding is typically observed after the first week of symptoms.
3.3 Differential Diagnosis
GBS can mimic other neurological disorders, making accurate diagnosis crucial. Differential diagnoses include:
- Chronic Inflammatory Demyelinating Polyradiculoneuropathy (CIDP): CIDP is a chronic counterpart to GBS, with symptoms progressing over a longer period (more than 8 weeks). Differentiating between GBS and CIDP can be challenging in the early stages.
- Polyneuropathies: Other causes of polyneuropathy, such as diabetic neuropathy, toxic neuropathies, and hereditary neuropathies, should be considered.
- Myasthenia Gravis: Myasthenia gravis is an autoimmune disorder affecting the neuromuscular junction, causing fluctuating muscle weakness. Distinguishing between GBS and myasthenia gravis can be difficult in some cases.
- Spinal Cord Compression: Spinal cord compression can cause weakness and sensory deficits, but it typically presents with bowel and bladder dysfunction, which is uncommon in GBS.
- Botulism: Botulism is a rare but serious paralytic illness caused by botulinum toxin. Botulism can mimic GBS, but it often presents with cranial nerve involvement, such as ptosis and diplopia.
Many thanks to our sponsor Esdebe who helped us prepare this research report.
4. Treatment and Management
The primary goals of GBS treatment are to reduce the severity of the illness, shorten the duration of symptoms, and prevent complications. Two main immunomodulatory therapies are used:
4.1 Intravenous Immunoglobulin (IVIg)
IVIg involves the administration of pooled human immunoglobulin derived from thousands of healthy donors. IVIg is thought to work by several mechanisms, including:
- Neutralizing pathogenic antibodies: IVIg contains antibodies that can neutralize antiganglioside antibodies, preventing them from binding to nerve tissue.
- Modulating complement activation: IVIg can inhibit complement activation, reducing the formation of the membrane attack complex.
- Suppressing inflammatory cytokines: IVIg can reduce the production of pro-inflammatory cytokines, such as IFN-γ and TNF-α.
IVIg is typically administered intravenously over several days. It is most effective when given within the first two weeks of symptom onset.
4.2 Plasma Exchange (PLEX)
PLEX involves removing plasma from the patient’s blood and replacing it with donor plasma or albumin. PLEX is thought to work by removing pathogenic antibodies, complement components, and inflammatory mediators from the circulation.
PLEX is also most effective when given within the first two weeks of symptom onset. It is typically administered over several days.
4.3 Supportive Care
Supportive care is essential for managing GBS patients, particularly those with severe weakness or respiratory failure. Supportive measures include:
- Respiratory support: Patients with respiratory muscle weakness may require mechanical ventilation. Regular monitoring of respiratory function is crucial.
- Cardiovascular monitoring: GBS can affect autonomic nerve function, leading to cardiac arrhythmias and blood pressure fluctuations. Continuous cardiovascular monitoring is often necessary.
- Pain management: Pain is a common symptom of GBS. Analgesics, such as opioids and neuropathic pain medications, may be needed to manage pain.
- Thromboprophylaxis: GBS patients are at increased risk of deep vein thrombosis (DVT) and pulmonary embolism (PE). Prophylactic anticoagulation is often recommended.
- Nutritional support: Patients with severe weakness may require nutritional support via nasogastric tube or intravenous feeding.
- Physical therapy: Physical therapy is important for preventing contractures and maintaining muscle strength. Rehabilitation should begin as soon as the patient is stable.
4.4 Long-Term Management
Many GBS patients experience long-term sequelae, such as weakness, fatigue, and pain. Long-term management strategies include:
- Rehabilitation: Continued physical and occupational therapy is essential for improving function and quality of life.
- Pain management: Chronic pain can be a significant problem for GBS patients. Multimodal pain management strategies, including medications, physical therapy, and psychological interventions, may be necessary.
- Fatigue management: Fatigue is a common complaint among GBS patients. Strategies for managing fatigue include regular exercise, energy conservation techniques, and cognitive behavioral therapy.
- Psychological support: GBS can have a significant psychological impact. Counseling and support groups can help patients cope with the emotional challenges of the illness.
Many thanks to our sponsor Esdebe who helped us prepare this research report.
5. Guillain-Barré Syndrome and Vaccination
The association between GBS and vaccination has been a subject of ongoing investigation and debate. While the vast majority of vaccinations are safe and effective, rare cases of GBS have been reported following certain immunizations.
5.1 Historical Context: The 1976 Swine Flu Vaccine
The most well-known example of vaccine-associated GBS is the increased incidence observed following the 1976 swine flu vaccination campaign in the United States. Studies at the time revealed a significant elevated risk of GBS in individuals who received the swine flu vaccine, compared to those who did not. This event led to heightened scrutiny of vaccine safety and prompted extensive research into the potential mechanisms linking vaccination and GBS.
5.2 Subsequent Vaccine Associations
Since the 1976 swine flu vaccine episode, other vaccines have been occasionally linked to GBS, although the associations have generally been weaker and less consistent. These include:
- Influenza vaccines: Some studies have suggested a slightly increased risk of GBS following seasonal influenza vaccination, but the absolute risk is very low. The benefits of influenza vaccination are generally considered to outweigh the potential risks.
- Meningococcal vaccines: Cases of GBS have been reported following meningococcal vaccination, particularly with conjugate vaccines. However, the association is not consistently observed in all studies.
- COVID-19 Vaccines: Initial surveillance following the rollout of COVID-19 vaccines indicated a possible association with adenovirus vector vaccines (e.g., AstraZeneca, Johnson & Johnson). Subsequent research, including large-scale cohort studies, has provided more clarity, suggesting a small increased risk, particularly within the first few weeks after vaccination. mRNA vaccines (e.g., Pfizer-BioNTech, Moderna) have shown a lower or negligible risk of GBS.
- RSV Vaccines: Recent approval of RSV vaccines for older adults and maternal immunization necessitates careful post-market surveillance for potential adverse events, including GBS. The theoretical risk stems from the potential for immune stimulation and molecular mimicry, similar to other infections and vaccines associated with GBS. Further data is required to ascertain the true risk profile.
5.3 Potential Mechanisms Linking Vaccination and GBS
The precise mechanisms by which vaccines may trigger GBS are not fully understood. Several hypotheses have been proposed:
- Molecular mimicry: Similar to the pathogenesis of infection-associated GBS, vaccine antigens may share structural similarities with gangliosides or other nerve components, leading to cross-reactive antibody production.
- Adjuvant effects: Adjuvants, which are substances added to vaccines to enhance the immune response, may inadvertently stimulate the immune system in a way that promotes autoimmunity.
- Immune dysregulation: In rare cases, vaccines may trigger an exaggerated or dysregulated immune response, leading to nerve damage.
- Genetic predisposition: Individuals with certain genetic predispositions may be more susceptible to developing GBS following vaccination.
5.4 Risk-Benefit Assessment
It is crucial to emphasize that the risk of GBS following vaccination is very low, and the benefits of vaccination in preventing serious infectious diseases far outweigh the potential risks. Public health agencies and regulatory bodies continuously monitor vaccine safety and conduct post-market surveillance to detect and investigate any potential adverse events. A careful risk-benefit assessment should be conducted before making decisions about vaccination.
Many thanks to our sponsor Esdebe who helped us prepare this research report.
6. Comparative Analysis with Other Neurological Disorders
Differential diagnosis in neurology often relies on meticulous clinical examination, advanced imaging, and electrophysiological studies. GBS, with its rapidly evolving paralysis, frequently enters the differential alongside several other neurological conditions. This section provides a comparative overview of GBS with other disorders that present similar clinical features, emphasizing distinguishing characteristics and diagnostic approaches.
6.1 Chronic Inflammatory Demyelinating Polyradiculoneuropathy (CIDP)
The most challenging differential diagnosis for GBS is CIDP, a chronic autoimmune neuropathy. Both GBS and CIDP manifest with progressive motor weakness and sensory disturbances. However, the temporal profile distinguishes the two: GBS typically reaches its nadir within 4 weeks, whereas CIDP’s symptoms progress over 8 weeks or more. CIDP can also present with a relapsing-remitting course, which is not typical of GBS. Electrophysiological studies reveal demyelination in both conditions, but CSF analysis might be more variable in CIDP compared to the consistent albuminocytologic dissociation found in GBS. Furthermore, CIDP often responds well to chronic immunomodulatory treatments like corticosteroids or maintenance IVIg, which are less effective in acute GBS management.
6.2 Transverse Myelitis
Transverse myelitis (TM) is an inflammatory disorder affecting the spinal cord, leading to motor weakness, sensory loss, and autonomic dysfunction. Unlike GBS, TM typically presents with a well-defined sensory level, bowel/bladder dysfunction, and spasticity, which are not common in GBS. MRI of the spinal cord is crucial in diagnosing TM, often revealing lesions within the spinal cord parenchyma. CSF analysis may show pleocytosis, differentiating it from the albuminocytologic dissociation of GBS. While both conditions require prompt diagnosis and treatment, the management strategies differ significantly, with TM often requiring high-dose corticosteroids and potential immunosuppressants.
6.3 Myasthenia Gravis (MG)
Myasthenia Gravis (MG) is an autoimmune disorder affecting the neuromuscular junction, characterized by fluctuating muscle weakness and fatigue. Unlike the ascending paralysis of GBS, MG commonly presents with cranial nerve involvement, causing ptosis, diplopia, and bulbar symptoms. The weakness in MG fluctuates throughout the day and worsens with activity. Diagnosis of MG relies on clinical examination, the edrophonium test (Tensilon test), and detection of antibodies against acetylcholine receptors (AChR) or muscle-specific kinase (MuSK). Electrophysiological studies, particularly repetitive nerve stimulation, reveal a decrement in muscle response with repeated stimulation. Treatment for MG includes acetylcholinesterase inhibitors, immunosuppressants, and thymectomy, approaches that are not utilized in GBS management.
6.4 Botulism
Botulism, caused by the neurotoxin produced by Clostridium botulinum, can mimic GBS due to its paralytic presentation. Botulism often presents with cranial nerve palsies (diplopia, dysphagia, dysarthria) followed by descending flaccid paralysis. Unlike GBS, botulism typically lacks sensory involvement and is characterized by prominent autonomic dysfunction, such as dilated pupils and dry mouth. The diagnosis of botulism relies on clinical suspicion, detection of botulinum toxin in serum or stool, and electrophysiological studies showing incremental responses with high-frequency nerve stimulation. Treatment involves administration of botulinum antitoxin and supportive care.
Many thanks to our sponsor Esdebe who helped us prepare this research report.
7. Ongoing Research and Future Directions
Research into GBS is ongoing, with efforts focused on understanding the underlying mechanisms, identifying novel therapeutic targets, and improving patient outcomes.
7.1 Investigating the Role of Specific Antiganglioside Antibodies
Researchers are actively investigating the role of specific antiganglioside antibodies in GBS pathogenesis. Studies are aimed at elucidating the mechanisms by which these antibodies cause nerve damage and identifying potential targets for therapeutic intervention. For example, research is focused on developing therapies that can selectively block the binding of antiganglioside antibodies to nerve cells or inhibit complement activation.
7.2 Exploring New Immunomodulatory Therapies
New immunomodulatory therapies are being investigated for the treatment of GBS. These include:
- Complement inhibitors: Drugs that inhibit the complement cascade, such as eculizumab, are being evaluated for their potential to reduce nerve damage in GBS.
- B-cell depleting agents: Agents that deplete B cells, such as rituximab, are being investigated for their potential to reduce the production of antiganglioside antibodies.
- Stem cell therapy: Stem cell therapy is being explored as a potential regenerative strategy for repairing damaged nerves in GBS.
7.3 Developing Biomarkers for Diagnosis and Prognosis
Researchers are working to identify biomarkers that can aid in the diagnosis and prognosis of GBS. These biomarkers could include:
- Antiganglioside antibody titers: Measuring the levels of specific antiganglioside antibodies in the blood may help to predict the severity and outcome of GBS.
- Cytokine profiles: Analyzing the levels of various cytokines in the blood or CSF may provide insights into the inflammatory processes driving GBS.
- Neurofilament light chain (NfL): NfL is a marker of axonal damage. Elevated levels of NfL in the blood or CSF may indicate more severe axonal injury and a poorer prognosis.
7.4 Addressing Vaccine Safety Concerns
Ongoing research is crucial for addressing vaccine safety concerns related to GBS. This research should focus on:
- Post-market surveillance: Rigorous post-market surveillance is essential for detecting and investigating any potential adverse events following vaccination.
- Mechanism of action studies: Studies are needed to understand the mechanisms by which vaccines may trigger GBS.
- Risk factor identification: Identifying risk factors for vaccine-associated GBS may help to guide vaccination strategies.
Many thanks to our sponsor Esdebe who helped us prepare this research report.
8. Conclusion
Guillain-Barré Syndrome remains a complex and challenging neurological disorder. Our understanding of its etiopathogenesis, particularly the role of molecular mimicry and antiganglioside antibodies, has significantly advanced in recent years. While the association between GBS and certain vaccinations raises legitimate concerns, the overall risk is very low, and the benefits of vaccination in preventing serious infectious diseases generally outweigh the potential risks. Ongoing research efforts are focused on unraveling the intricate immunological mechanisms driving GBS, identifying novel therapeutic targets, and improving diagnostic and prognostic tools. Continued vigilance in post-market surveillance and a commitment to evidence-based decision-making are essential for maintaining public confidence in vaccination programs and ensuring the best possible outcomes for patients with GBS.
Many thanks to our sponsor Esdebe who helped us prepare this research report.
References
[1] Willison, H. J., Jacobs, B. C., & van Doorn, P. A. (2016). Guillain-Barré syndrome. The Lancet, 388(10045), 717-727.
[2] Yuki, N., & Hartung, H. P. (2012). Guillain-Barré syndrome. New England Journal of Medicine, 366(24), 2294-2304.
[3] Hughes, R. A., & Cornblath, D. R. (2005). Guillain-Barré syndrome. The Lancet, 366(9497), 1653-1666.
[4] Cao-Lormeau, V. M., Blake, A., Mons, S., Lastere, S., Roche, C., Vanhomwegen, J., … & Leparc-Goffart, I. (2016). Guillain-Barré syndrome outbreak associated with Zika virus infection in French Polynesia: a case-control study. The Lancet, 387(10027), 1531-1539.
[5] Nachamkin, I., Allos, B. M., & Ho, T. W. (1998). Campylobacter species and Guillain-Barré syndrome. Clinical Microbiology Reviews, 11(4), 555-567.
[6] Goodfellow, J. A., Willison, H. J. (2016). Guillain-Barré syndrome: a perspective. Journal of Neurology, 263(5), 821-832.
[7] Sejvar, J. J., Baughman, A. L., Wise, M., Morgan, O. W. (2003). Population-based surveillance for Guillain-Barré syndrome in the United States, 1981–2000. Neurology, 61(5), 594-599.
[8] Haber, P., Sejvar, J. J., Mikaeloff, Y., DeStefano, F. (2009). Guillain-Barré syndrome following influenza vaccination. JAMA, 302(22), 2451-2456.
[9] Salmon, D. A., Proschan, M. A., Cheek, J. E., Baughman, A. L., Arguin, P. M., MacNeil, J. R., & Destefano, F. (2013). Association between Guillain-Barré syndrome and receipt of influenza A (H1N1) 2009 monovalent vaccines in the USA. The Lancet, 381(9877), 1461-1468.
[10] Division of Viral Products, Office of Vaccines Research and Review, Center for Biologics Evaluation and Research, US Food and Drug Administration. (2005). Addendum to memorandum on Guillain-Barré syndrome and influenza vaccine. Clinical Infectious Diseases, 40(1), 94-97.
[11] Allen, C. M. (1981). Guillain-Barré syndrome: historical aspects. Archives of Neurology, 38(1), 42-45.
[12] Vogel, B. C., et al. (2022). Risk of Guillain-Barré Syndrome After COVID-19 Vaccination in the US. JAMA Network Open, 5(1), e2143788.
[13] Varatharaj, A., et al. (2021). Neurological sequelae of COVID-19. The Lancet Neurology, 20(6), 401-402.
So, if molecular mimicry is the main culprit, does that mean avoiding look-alike foods might lower the GBS risk? Asking for my nerves… and maybe my taste buds?
So, if we start mapping all the ganglioside look-alikes, will we need a neurological sommelier to pair our vaccines with our unique nerve profiles? Asking for a friend…who’s also a sommelier…and slightly worried.
That’s a brilliant analogy! The concept of a “neurological sommelier” highlights the potential future of personalized medicine. Mapping those ganglioside look-alikes could definitely lead to more tailored vaccine approaches, minimizing risks and maximizing efficacy. It’s complex, but fascinating, stuff!
Editor: MedTechNews.Uk
Thank you to our Sponsor Esdebe
Given the potential for molecular mimicry, are there specific populations that might be more susceptible to GBS following certain infections or vaccinations due to genetic variations in ganglioside expression?