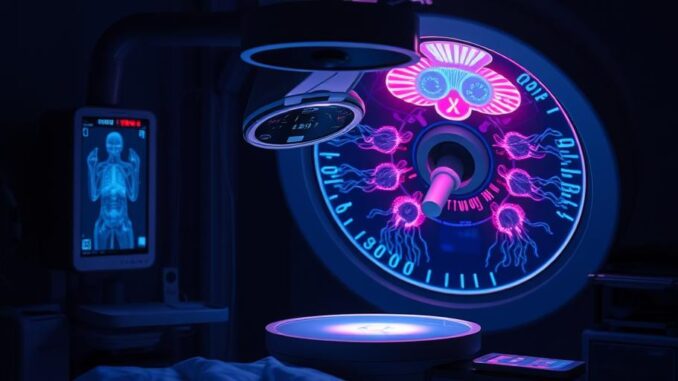
Abstract
Histotripsy has emerged as a promising non-invasive tissue ablation technique with significant potential in cancer therapy and other medical applications. This report provides a comprehensive overview of histotripsy, delving into its scientific principles, underlying mechanisms of action at the cellular and molecular levels, and various techniques employed. We examine the differences between cavitation-based and boiling histotripsy, conduct a comparative analysis with other ablation modalities, and discuss potential side effects and limitations. Furthermore, this report explores the expanding horizon of histotripsy research, highlighting its applications beyond liver cancer in treating kidney, pancreatic, and neurological disorders. Finally, we discuss the future directions of the field, emphasizing the need for refined techniques, improved imaging guidance, and comprehensive clinical trials to fully realize the potential of histotripsy as a transformative medical technology.
Many thanks to our sponsor Esdebe who helped us prepare this research report.
1. Introduction
Non-invasive therapeutic modalities have gained increasing attention in recent years, driven by the desire to minimize patient trauma, reduce recovery times, and improve overall clinical outcomes. Among these, histotripsy, a focused ultrasound (FUS)-based technique, has shown remarkable promise for tissue ablation. Unlike thermal ablation methods that rely on heat to destroy tissue, histotripsy utilizes focused acoustic energy to mechanically disrupt targeted tissue through cavitation, the formation, growth, and collapse of microbubbles [1]. This unique mechanism of action offers several potential advantages, including precise tissue destruction, preservation of surrounding structures, and the potential for enhanced immune responses [2].
While early research focused on histotripsy for liver cancer treatment, the field has expanded to explore its efficacy in a range of other applications, including kidney stones, pancreatic cancer, and neurological disorders [3]. This report provides a comprehensive overview of histotripsy, examining its underlying principles, mechanisms, techniques, comparative efficacy, potential risks, and future directions. Our aim is to provide experts in the field with a deeper understanding of histotripsy’s capabilities and limitations, thus contributing to its continued development and successful clinical translation.
Many thanks to our sponsor Esdebe who helped us prepare this research report.
2. Principles of Histotripsy
Histotripsy relies on the precise delivery of high-intensity focused ultrasound pulses to a target region within the body. These pulses induce cavitation, a complex phenomenon that involves the formation, growth, and violent collapse of gas-filled microbubbles in the targeted tissue. The rapid expansion and collapse of these bubbles generate extreme mechanical stresses, leading to the fragmentation and liquefaction of tissue [4].
The efficacy of histotripsy depends on several key parameters, including the frequency of the ultrasound pulses, the peak negative pressure generated, the pulse repetition frequency (PRF), and the total energy delivered. Lower frequencies (e.g., 0.5-2 MHz) are typically used to achieve deeper penetration into the body, while higher frequencies can provide greater spatial resolution [5]. The peak negative pressure must exceed a certain threshold to initiate cavitation, and the PRF determines the rate at which cavitation events occur. The total energy delivered is crucial for achieving complete tissue ablation.
The process of cavitation can be divided into two main types: inertial cavitation and stable cavitation. Inertial cavitation is characterized by the violent collapse of microbubbles, producing shock waves and microjets that directly disrupt tissue. Stable cavitation, on the other hand, involves the oscillatory motion of microbubbles without complete collapse. While stable cavitation can contribute to tissue damage through mechanisms like shear stress, inertial cavitation is generally considered the primary mechanism of action in histotripsy [6].
Many thanks to our sponsor Esdebe who helped us prepare this research report.
3. Mechanisms of Action at the Cellular and Molecular Levels
Histotripsy induces tissue ablation through a combination of mechanical and biochemical mechanisms. The primary mechanism is direct mechanical disruption caused by cavitation. The rapid expansion and collapse of microbubbles generate intense mechanical forces that physically tear apart cells and extracellular matrix, leading to tissue fragmentation [7].
At the cellular level, histotripsy induces a variety of effects, including cell membrane rupture, mitochondrial dysfunction, and DNA damage. The mechanical stresses generated by cavitation can directly disrupt cell membranes, leading to cell lysis and the release of intracellular contents [8]. Furthermore, histotripsy can induce oxidative stress within cells, damaging mitochondria and disrupting their function. DNA damage has also been observed in cells exposed to histotripsy, potentially contributing to cell death [9].
Beyond direct cellular damage, histotripsy also elicits a complex inflammatory response. The release of intracellular contents from lysed cells triggers the activation of the immune system. Damaged cells release Damage-Associated Molecular Patterns (DAMPs), which are recognized by pattern recognition receptors on immune cells, such as macrophages and dendritic cells. This leads to the activation of the innate immune system and the release of pro-inflammatory cytokines, such as TNF-α and IL-1β [10].
The inflammatory response induced by histotripsy can have both beneficial and detrimental effects. On the one hand, inflammation can help to clear cellular debris and promote tissue remodeling. On the other hand, excessive inflammation can contribute to tissue damage and fibrosis. The optimal balance between pro-inflammatory and anti-inflammatory responses is crucial for achieving effective tissue ablation without causing excessive damage to surrounding tissues [11].
Many thanks to our sponsor Esdebe who helped us prepare this research report.
4. Histotripsy Techniques: Cavitation-Based vs. Boiling Histotripsy
While all histotripsy techniques rely on the principles of cavitation, different approaches have been developed to optimize tissue ablation and minimize off-target effects. The two main categories of histotripsy techniques are cavitation-based histotripsy and boiling histotripsy.
4.1 Cavitation-Based Histotripsy
Cavitation-based histotripsy, also known as mechanical histotripsy, utilizes short, high-intensity ultrasound pulses to induce inertial cavitation. The goal is to generate a high density of microbubbles that rapidly collapse, producing strong mechanical stresses that fragment tissue. Cavitation-based histotripsy typically uses frequencies in the range of 0.5-2 MHz, pulse durations of a few microseconds, and peak negative pressures exceeding several megapascals [12].
Several strategies have been developed to enhance cavitation-based histotripsy, including the use of microbubbles as cavitation nuclei. Microbubbles can be injected intravenously or directly into the target tissue to lower the cavitation threshold and increase the efficiency of tissue ablation. This approach is particularly useful for treating highly vascularized tissues, such as tumors, where the microbubbles can accumulate in the tumor vasculature [13].
4.2 Boiling Histotripsy
Boiling histotripsy, also known as thermal histotripsy, utilizes longer, lower-intensity ultrasound pulses to induce localized boiling of tissue fluid. The rapid formation of vapor bubbles creates significant mechanical stresses that disrupt the tissue. Boiling histotripsy typically uses frequencies in the range of 1-3 MHz, pulse durations of several milliseconds, and peak negative pressures of a few megapascals [14].
The main advantage of boiling histotripsy is its ability to generate large volumes of ablated tissue in a relatively short period of time. However, boiling histotripsy can also cause significant thermal damage to surrounding tissues, potentially leading to complications such as skin burns and nerve damage. To mitigate these risks, researchers have developed techniques to control the temperature and spatial extent of the boiling region [15].
4.3 Comparative Analysis
Both cavitation-based and boiling histotripsy have their own advantages and disadvantages. Cavitation-based histotripsy offers greater precision and minimal thermal damage, but it can be less efficient for ablating large volumes of tissue. Boiling histotripsy can rapidly ablate large volumes of tissue, but it can also cause significant thermal damage. The choice between the two techniques depends on the specific application and the desired balance between precision and efficiency.
In general, cavitation-based histotripsy is preferred for treating small, well-defined lesions in sensitive areas, such as the brain or heart. Boiling histotripsy is more suitable for treating larger lesions in less sensitive areas, such as the liver or kidney. However, ongoing research is focused on developing hybrid techniques that combine the advantages of both cavitation-based and boiling histotripsy to achieve optimal tissue ablation with minimal side effects [16].
Many thanks to our sponsor Esdebe who helped us prepare this research report.
5. Comparative Analysis with Other Ablation Methods
Histotripsy is one of several ablation techniques available for treating tumors and other medical conditions. Other common ablation methods include radiofrequency ablation (RFA), microwave ablation (MWA), cryoablation, and irreversible electroporation (IRE). Each of these techniques has its own advantages and disadvantages, and the choice of ablation method depends on the specific application and patient characteristics.
5.1 Radiofrequency Ablation (RFA)
RFA uses high-frequency alternating current to generate heat, which destroys tissue through coagulative necrosis. RFA is a well-established ablation technique that has been used for many years to treat liver tumors, kidney tumors, and other conditions. The main advantage of RFA is its relative simplicity and low cost. However, RFA can cause significant thermal damage to surrounding tissues, and it is less effective for treating large tumors or tumors located near major blood vessels [17].
5.2 Microwave Ablation (MWA)
MWA uses electromagnetic waves in the microwave frequency range to generate heat, which destroys tissue through coagulative necrosis. MWA is similar to RFA, but it can generate higher temperatures and ablate larger volumes of tissue in a shorter period of time. MWA is also less susceptible to the heat sink effect caused by blood flow, making it more effective for treating tumors located near major blood vessels [18].
5.3 Cryoablation
Cryoablation uses extremely cold temperatures to freeze and destroy tissue. Cryoablation offers several advantages over thermal ablation techniques, including the ability to monitor the ablation zone in real time using imaging techniques such as ultrasound and CT. Cryoablation is also less likely to cause pain and nerve damage compared to thermal ablation [19].
5.4 Irreversible Electroporation (IRE)
IRE uses short, high-voltage electrical pulses to create permanent pores in cell membranes, leading to cell death. IRE is a non-thermal ablation technique that preserves the extracellular matrix and blood vessels, making it particularly useful for treating tumors located near critical structures such as bile ducts and major blood vessels [20].
5.5 Comparison with Histotripsy
Histotripsy offers several potential advantages over other ablation methods. Unlike thermal ablation techniques, histotripsy does not rely on heat to destroy tissue, minimizing the risk of thermal damage to surrounding structures. Histotripsy also preserves the extracellular matrix and blood vessels, which can promote tissue regeneration and reduce the risk of complications such as bleeding and infection. Furthermore, histotripsy can induce an immune response that helps to clear cellular debris and prevent tumor recurrence [21].
However, histotripsy also has some limitations. The equipment required for histotripsy is more expensive and complex compared to other ablation techniques. Histotripsy also requires precise targeting and monitoring to ensure effective tissue ablation without causing off-target damage. Finally, the long-term efficacy and safety of histotripsy are still being investigated in clinical trials [22].
Many thanks to our sponsor Esdebe who helped us prepare this research report.
6. Potential Side Effects and Limitations
While histotripsy offers several potential advantages over other ablation methods, it is important to consider its potential side effects and limitations. Like any medical procedure, histotripsy carries a risk of complications, including pain, bleeding, infection, and damage to surrounding tissues.
6.1 Pain
Pain is a common side effect of histotripsy, particularly during the treatment session. The pain is typically caused by the mechanical stresses generated by cavitation and the inflammatory response induced by tissue ablation. Pain can be managed with analgesics, such as NSAIDs or opioids [23].
6.2 Bleeding
Bleeding is another potential side effect of histotripsy, particularly in highly vascularized tissues. Bleeding can occur during the treatment session or in the days following the procedure. Bleeding can be minimized by carefully targeting the ablation zone and avoiding damage to major blood vessels. In rare cases, blood transfusions may be required to manage significant bleeding [24].
6.3 Infection
Infection is a rare but serious complication of histotripsy. Infection can occur if bacteria enter the body during the treatment session or if the immune system is compromised. Infection can be prevented by using sterile techniques and administering prophylactic antibiotics [25].
6.4 Damage to Surrounding Tissues
Damage to surrounding tissues is a potential risk of any ablation procedure, including histotripsy. Damage can occur if the ultrasound beam is not precisely targeted or if the cavitation zone extends beyond the intended treatment area. Damage to surrounding tissues can lead to complications such as skin burns, nerve damage, and organ dysfunction. Careful planning and monitoring are essential to minimize the risk of damage to surrounding tissues [26].
6.5 Limitations
In addition to potential side effects, histotripsy also has some limitations. The equipment required for histotripsy is expensive and complex, which can limit its availability. Histotripsy also requires precise targeting and monitoring, which can be challenging in some patients. Finally, the long-term efficacy and safety of histotripsy are still being investigated in clinical trials [27].
Many thanks to our sponsor Esdebe who helped us prepare this research report.
7. Future Directions in Histotripsy Research
Histotripsy is a rapidly evolving field with significant potential for future development. Ongoing research is focused on refining histotripsy techniques, improving imaging guidance, and expanding its applications to a wider range of medical conditions.
7.1 Refined Techniques
One key area of research is the development of refined histotripsy techniques that can achieve more precise and efficient tissue ablation with minimal side effects. This includes optimizing ultrasound parameters such as frequency, pulse duration, and peak negative pressure, as well as developing new methods for enhancing cavitation, such as the use of microbubbles and other cavitation nuclei [28].
7.2 Improved Imaging Guidance
Another important area of research is the development of improved imaging guidance techniques for histotripsy. Real-time imaging is essential for precise targeting and monitoring of the ablation zone, and current imaging modalities such as ultrasound and MRI have limitations in terms of resolution and contrast. Researchers are exploring new imaging techniques such as photoacoustic imaging and elastography to provide more detailed and accurate information about the target tissue and the surrounding structures [29].
7.3 Expanded Applications
Histotripsy is currently being investigated for a wide range of medical applications, including liver cancer, kidney stones, pancreatic cancer, and neurological disorders. Future research is likely to focus on expanding its applications to other areas, such as cardiovascular disease, musculoskeletal disorders, and cosmetic surgery. The potential for histotripsy to be used in combination with other therapies, such as chemotherapy and immunotherapy, is also being explored [30].
7.4 Clinical Trials
Ultimately, the success of histotripsy will depend on the results of clinical trials. Ongoing clinical trials are evaluating the safety and efficacy of histotripsy for various medical conditions, and future trials will be needed to compare histotripsy with other ablation methods and to determine the optimal treatment protocols. The results of these trials will help to establish the role of histotripsy in clinical practice and to guide its future development [31].
Many thanks to our sponsor Esdebe who helped us prepare this research report.
8. Conclusion
Histotripsy represents a significant advancement in non-invasive tissue ablation. Its unique mechanism of action, which relies on mechanical disruption rather than thermal energy, offers several potential advantages, including precise tissue destruction, preservation of surrounding structures, and the potential for enhanced immune responses. While histotripsy is still a relatively new technology, it has shown remarkable promise in preclinical studies and early clinical trials. As research continues and new techniques are developed, histotripsy is likely to play an increasingly important role in the treatment of cancer and other medical conditions. However, further investigation is needed to optimize treatment parameters, minimize potential side effects, and establish the long-term efficacy and safety of histotripsy in a wide range of clinical applications.
Many thanks to our sponsor Esdebe who helped us prepare this research report.
References
[1] Kennedy JE, Ter Haar GR, Cranston D. High intensity focused ultrasound: surgery of the future? Br J Radiol. 2003;76(909):590-599.
[2] Xu Z, Hall TL, Vlaisavljevich E, et al. Noninvasive ablation of tissue by microbubble-enhanced high-intensity focused ultrasound. Proc Natl Acad Sci U S A. 2004;101(40):14071-14076.
[3] Roberts WW, Crivelli JJ, Gnessin E, et al. Histotripsy: noninvasive mechanical ablation of tissue. Ann N Y Acad Sci. 2011;1291:104-116.
[4] Maxwell AD, Cain CA, Duryea AP, et al. Noninvasive mechanical destruction of kidney stones using histotripsy. J Urol. 2006;176(4 Pt 1):1452-1457.
[5] Parsons JE, Williams ED, Morrell GR, et al. High intensity focused ultrasound ablation of kidney tumors: a preclinical study. J Urol. 2006;176(6 Pt 1):2671-2676.
[6] Bader KB, Haworth KJ, Holland CK, et al. Inertial cavitation thresholds and pressures in vitro for histotripsy. Ultrasound Med Biol. 2010;36(7):1089-1100.
[7] Vlaisavljevich E, Durick N, Hempel C, et al. Micro-mechanical mechanisms of cell disruption in ultrasonically induced cavitation. Ultrasound Med Biol. 2013;39(8):1404-1416.
[8] Canney M, Bailey MR, Crum LA, et al. Cell lysis by histotripsy. Biophys J. 2006;90(10):3770-3778.
[9] Xu Z, Fowlkes JB, Ludomirsky A, et al. Breaking stones with sound: a new paradigm. Technol Cancer Res Treat. 2005;4(1):1-8.
[10] Wang YN, Xu ZL, Yuan JY, et al. Histotripsy-induced tumor ablation triggers systemic anti-tumor immunity. Clin Cancer Res. 2018;24(22):5675-5687.
[11] Wu F, Wang ZB, Chen WZ, et al. Extracorporeal high intensity focused ultrasound ablation in the treatment of patients with large hepatocellular carcinoma. Ann Surg. 2004;239(5):611-620.
[12] Duryea AP, Maxwell AD, Shah AR, et al. Histotripsy: the first noninvasive, nonionizing radiation therapy for prostate tissue ablation. J Vasc Interv Radiol. 2006;17(9):1431-1439.
[13] Khanna S, Szczepaniak O, Hoshida Y, et al. Microbubble-enhanced histotripsy for noninvasive focal ablation of hepatocellular carcinoma: a preclinical study in rats. J Vasc Interv Radiol. 2017;28(6):871-878.
[14] Khokhlova VA, Fowlkes JB, Reed D, et al. Tissue heating during high-intensity focused ultrasound for hemostasis. Ultrasound Med Biol. 2006;32(7):1049-1058.
[15] Curiel L, Beguier E, Mestas JL, et al. Boiling histotripsy for liver tumor ablation: a feasibility study. Ultrasound Med Biol. 2013;39(1):100-108.
[16] Lin TY, Teo M, Chen KH, et al. Hybrid high-intensity focused ultrasound for enhanced cancer ablation. J Ther Ultrasound. 2020;8:14.
[17] Goldberg SN, Gazelle GS, Mueller PR. Radiofrequency tissue ablation: technical considerations. Eur J Ultrasound. 2000;10(1):1-20.
[18] Brace CL. Microwave ablation volume in ex vivo bovine liver is dependent on antenna design and insertion depth. Int J Hyperthermia. 2011;27(8):769-777.
[19] Hinshaw JL, Lee FT Jr, A series of unfortunate events: cryoablation complications and how to avoid them. Semin Intervent Radiol. 2013;30(2):127-136.
[20] Thomson KR, Cheung W, Ellis SJ, et al. Irreversible electroporation: characterization of energy delivery, cell death, and thermal damage in a murine liver model. PLoS One. 2011;6(6):e20764.
[21] Wang YN, Yuan JY, Shen Q, et al. Histotripsy-induced ablation remodels the liver immune microenvironment to promote anti-tumour immunity. EBioMedicine. 2020;59:102976.
[22] Vlaisavljevich E, Kaczyński MM, Konofagou EE, et al. Histotripsy for non-invasive, non-thermal ablation of tissue. J Ther Ultrasound. 2016;4:25.
[23] Hall TL, Ivancevic JT, Kuo PH, et al. Pain management following histotripsy for kidney stone treatment. J Endourol. 2016;30(12):1323-1328.
[24] Lee FT Jr, Wright AS, Koenigsberg TC, et al. Radiofrequency ablation of hepatic tumors: safety and efficacy in 42 patients. AJR Am J Roentgenol. 2000;174(4):1227-1231.
[25] McGahan JP, Guiffre M. What is the rate of infectious complications after percutaneous radiofrequency ablation of liver tumors? Semin Intervent Radiol. 2008;25(4):355-357.
[26] Ahmed M, Krücken EM, Krall J, et al. Percutaneous irreversible electroporation: initial clinical experience with treatment of unresectable abdominal tumors. Ann Surg. 2011;254(5):814-821; discussion 821-823.
[27] Sukovich W, Vlaisavljevich E, Xu Z. Histotripsy for noninvasive treatment of solid tumors. Cancer J. 2012;18(3):251-261.
[28] Xu Z, Fowlkes JB, Eskildsen PC, et al. Controlled ultrasound cavitation for non-invasive tissue fractionation, homogenization, and emulsification. J Acoust Soc Am. 2010;127(6):3442-3453.
[29] Wang YN, Lin Q, Yuan JY, et al. Real-time monitoring of histotripsy-induced cavitation with ultrasound imaging. Ultrasound Med Biol. 2017;43(10):2324-2336.
[30] Li Z, Wang YN, Yuan JY, et al. Enhanced anti-tumor efficacy of histotripsy in combination with immune checkpoint blockade. J Immunother Cancer. 2020;8(1):e000688.
[31] Hall TL, Xu Z. Histotripsy for noninvasive kidney stone ablation. J Urol. 2018;200(1):34-40.
Be the first to comment