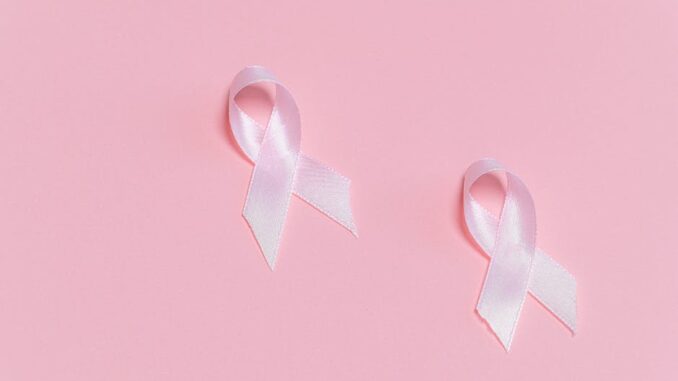
Abstract
Immunotherapy, a revolutionary approach to cancer treatment, leverages the inherent power of the immune system to recognize and eliminate malignant cells. This report provides a comprehensive overview of immunotherapy, exploring its diverse modalities, mechanisms of action, clinical applications, and potential challenges. We delve into the intricacies of immune checkpoint inhibitors, adoptive cell therapies, oncolytic viruses, cancer vaccines, and other emerging strategies. Furthermore, we critically analyze the factors influencing treatment response, the management of immune-related adverse events, and the evolving landscape of combination therapies and personalized immunotherapy approaches. Finally, we address the critical need for further research to overcome existing limitations and unlock the full potential of immunotherapy for a broader range of cancers and patients.
Many thanks to our sponsor Esdebe who helped us prepare this research report.
1. Introduction
The field of cancer immunotherapy has witnessed remarkable progress in recent years, transforming the treatment landscape for various malignancies. The premise of immunotherapy lies in harnessing the body’s own immune system to selectively target and destroy cancer cells, offering the potential for durable responses and long-term survival. Unlike conventional therapies such as chemotherapy and radiation, which often lack specificity and can damage healthy tissues, immunotherapy aims to precisely engage the immune system to eradicate cancer while minimizing off-target effects. The conceptual foundation of immunotherapy dates back to the late 19th century, with early attempts to stimulate the immune system using bacterial toxins. However, significant breakthroughs in understanding the intricate mechanisms of immune regulation have paved the way for the development of more effective and targeted immunotherapeutic strategies. This report provides a comprehensive overview of the major types of immunotherapy, their mechanisms of action, clinical applications, challenges, and future directions.
Many thanks to our sponsor Esdebe who helped us prepare this research report.
2. Types of Immunotherapy
Immunotherapy encompasses a diverse range of approaches, each with its unique mechanism of action and clinical application. The following sections provide a detailed discussion of the major types of immunotherapy:
2.1 Immune Checkpoint Inhibitors
Immune checkpoint inhibitors (ICIs) are monoclonal antibodies that block inhibitory receptors on immune cells, primarily T cells, thereby unleashing their anti-tumor activity. These receptors, such as cytotoxic T-lymphocyte-associated protein 4 (CTLA-4), programmed cell death protein 1 (PD-1), and programmed cell death ligand 1 (PD-L1), act as brakes on the immune system, preventing excessive activation and autoimmunity. Cancer cells often exploit these checkpoints to evade immune surveillance. By blocking these inhibitory pathways, ICIs enhance T cell activation, proliferation, and cytotoxicity, leading to tumor regression. The first ICI approved by the FDA was ipilimumab, an anti-CTLA-4 antibody, for the treatment of metastatic melanoma [1]. Subsequently, anti-PD-1 antibodies (e.g., pembrolizumab, nivolumab) and anti-PD-L1 antibodies (e.g., atezolizumab, durvalumab, avelumab) have been approved for a wide range of cancers, including lung cancer, kidney cancer, bladder cancer, Hodgkin lymphoma, and head and neck cancer. The success of ICIs has revolutionized cancer treatment, but it is important to note that only a subset of patients respond to these therapies, and immune-related adverse events (irAEs) can occur.
2.2 Adoptive Cell Therapies
Adoptive cell therapies (ACTs) involve the collection, modification, and expansion of a patient’s own immune cells (or donor cells) ex vivo, followed by their reinfusion into the patient to enhance their anti-tumor activity. The most prominent type of ACT is chimeric antigen receptor (CAR) T-cell therapy.
2.2.1 CAR T-Cell Therapy
CAR T-cell therapy involves genetically engineering a patient’s T cells to express a CAR, which is a synthetic receptor that recognizes a specific antigen on cancer cells. The CAR typically consists of an extracellular antigen-binding domain (e.g., a single-chain variable fragment [scFv]), a transmembrane domain, and intracellular signaling domains that activate the T cell upon antigen binding. Once infused into the patient, CAR T cells bind to the target antigen on cancer cells, leading to T cell activation, cytokine release, and ultimately, tumor cell lysis. CAR T-cell therapy has shown remarkable success in treating hematological malignancies, particularly B-cell lymphomas and acute lymphoblastic leukemia (ALL) [2]. Several CAR T-cell products have been approved by the FDA, including tisagenlecleucel, axicabtagene ciloleucel, and lisocabtagene maraleucel, all targeting the CD19 antigen on B cells. However, CAR T-cell therapy is associated with significant toxicities, such as cytokine release syndrome (CRS) and neurotoxicity, which require careful monitoring and management.
2.2.2 Tumor-Infiltrating Lymphocytes (TILs)
TIL therapy involves the isolation and expansion of T cells that have naturally infiltrated a patient’s tumor. These TILs are thought to be pre-selected for their ability to recognize tumor-associated antigens. After expansion in vitro, TILs are infused back into the patient, often following lymphodepleting chemotherapy to create space for the infused cells and enhance their activity. TIL therapy has shown promising results in treating metastatic melanoma and is being investigated in other solid tumors [3].
2.3 Oncolytic Viruses
Oncolytic viruses (OVs) are genetically engineered or naturally occurring viruses that selectively infect and kill cancer cells while sparing normal cells. OVs can induce tumor cell lysis through direct viral replication and also stimulate an anti-tumor immune response by releasing tumor-associated antigens and inflammatory cytokines. Talimogene laherparepvec (T-VEC), an oncolytic herpes simplex virus type 1 (HSV-1), was the first OV approved by the FDA for the treatment of metastatic melanoma [4]. T-VEC expresses granulocyte-macrophage colony-stimulating factor (GM-CSF), which further enhances the anti-tumor immune response. OVs are being investigated in combination with other immunotherapies and conventional therapies to improve efficacy.
2.4 Cancer Vaccines
Cancer vaccines aim to stimulate the immune system to recognize and attack cancer cells by exposing it to tumor-associated antigens. Cancer vaccines can be broadly classified into prophylactic vaccines, which prevent cancer development (e.g., HPV vaccine), and therapeutic vaccines, which treat existing cancers. Therapeutic cancer vaccines can be further categorized into peptide vaccines, protein vaccines, cell-based vaccines, and gene-based vaccines. Sipuleucel-T, an autologous cellular immunotherapy, is an FDA-approved cancer vaccine for the treatment of asymptomatic or minimally symptomatic metastatic castration-resistant prostate cancer [5]. Sipuleucel-T is prepared by culturing a patient’s peripheral blood mononuclear cells (PBMCs) with a recombinant protein consisting of prostatic acid phosphatase (PAP) fused to GM-CSF. The activated PBMCs are then infused back into the patient to stimulate an anti-tumor immune response. While cancer vaccines have shown promise in clinical trials, their efficacy has been limited by factors such as immune tolerance and tumor heterogeneity. Strategies to enhance the immunogenicity of cancer vaccines, such as the use of adjuvants and combination therapies, are being actively investigated.
2.5 Cytokines
Cytokines are signaling molecules that play a critical role in regulating the immune system. Some cytokines, such as interleukin-2 (IL-2) and interferon-alpha (IFN-α), have been used as immunotherapeutic agents to stimulate anti-tumor immune responses. High-dose IL-2 has been approved for the treatment of metastatic melanoma and renal cell carcinoma [6]. However, high-dose IL-2 is associated with significant toxicities, such as capillary leak syndrome, which limits its use. IFN-α has been used to treat hairy cell leukemia, chronic myelogenous leukemia, and Kaposi sarcoma. Other cytokines, such as tumor necrosis factor-alpha (TNF-α), have shown anti-tumor activity but are too toxic for systemic administration. Strategies to target cytokines specifically to the tumor microenvironment are being developed to improve their therapeutic efficacy and reduce systemic toxicity.
Many thanks to our sponsor Esdebe who helped us prepare this research report.
3. Mechanisms of Action
The mechanisms of action of immunotherapy are complex and multifaceted, involving interactions between immune cells, cancer cells, and the tumor microenvironment. The following sections provide a detailed discussion of the key mechanisms involved in immunotherapy:
3.1 T Cell Activation and Cytotoxicity
The activation of T cells is a central event in most immunotherapeutic strategies. T cell activation requires two signals: (1) the recognition of a tumor-associated antigen presented by major histocompatibility complex (MHC) molecules on antigen-presenting cells (APCs) or cancer cells, and (2) a costimulatory signal provided by molecules such as CD28 on T cells interacting with CD80/CD86 on APCs. Once activated, T cells proliferate, differentiate into effector cells (e.g., cytotoxic T lymphocytes [CTLs]), and migrate to the tumor site. CTLs recognize and kill cancer cells by releasing cytotoxic granules containing perforin and granzymes, which induce apoptosis. ICIs enhance T cell activation by blocking inhibitory receptors that dampen T cell responses. CAR T cells bypass the need for MHC-mediated antigen presentation by directly recognizing tumor-associated antigens through their engineered CARs.
3.2 Modulation of the Tumor Microenvironment
The tumor microenvironment (TME) is a complex ecosystem consisting of cancer cells, immune cells, stromal cells, blood vessels, and extracellular matrix. The TME can promote tumor growth and metastasis by suppressing anti-tumor immune responses, promoting angiogenesis, and facilitating immune evasion. Immunotherapy can modulate the TME by enhancing the infiltration of immune cells, promoting the polarization of macrophages and myeloid-derived suppressor cells (MDSCs) towards an anti-tumor phenotype, and inhibiting angiogenesis. Oncolytic viruses can also disrupt the TME by directly killing cancer cells and releasing inflammatory cytokines that attract immune cells.
3.3 Induction of Immunogenic Cell Death
Immunogenic cell death (ICD) is a form of cell death that triggers an anti-tumor immune response. ICD is characterized by the release of damage-associated molecular patterns (DAMPs), such as calreticulin, ATP, and high-mobility group box 1 (HMGB1), which activate APCs and promote the cross-presentation of tumor-associated antigens to T cells. Some chemotherapeutic agents and radiation therapy can induce ICD. Oncolytic viruses can also induce ICD by causing tumor cell lysis and releasing DAMPs. Strategies to enhance ICD, such as combining immunotherapy with ICD-inducing agents, are being investigated to improve treatment efficacy.
Many thanks to our sponsor Esdebe who helped us prepare this research report.
4. Clinical Applications
Immunotherapy has demonstrated remarkable clinical efficacy in a wide range of cancers. The following sections highlight some of the key clinical applications of immunotherapy:
4.1 Melanoma
Melanoma was one of the first cancers to be successfully treated with immunotherapy. Ipilimumab, an anti-CTLA-4 antibody, was the first ICI approved for the treatment of metastatic melanoma [1]. Subsequently, anti-PD-1 antibodies (e.g., pembrolizumab, nivolumab) have shown even greater efficacy in melanoma, with durable responses and improved survival rates. Combination therapy with ipilimumab and nivolumab has also been approved for melanoma, but is associated with higher rates of irAEs. T-VEC, an oncolytic virus, has been approved for the treatment of metastatic melanoma that is not amenable to surgical resection. TIL therapy has also shown promising results in melanoma, with high response rates in selected patients.
4.2 Lung Cancer
Immunotherapy has revolutionized the treatment of lung cancer, particularly non-small cell lung cancer (NSCLC). Anti-PD-1 antibodies (e.g., pembrolizumab, nivolumab) and anti-PD-L1 antibodies (e.g., atezolizumab, durvalumab) have been approved as monotherapy or in combination with chemotherapy for NSCLC [7]. The efficacy of ICIs in lung cancer is correlated with the expression level of PD-L1 on tumor cells. Patients with high PD-L1 expression are more likely to respond to ICI monotherapy. Combination therapy with ICIs and chemotherapy has shown improved efficacy in patients with low PD-L1 expression. Immunotherapy is also being investigated in small cell lung cancer (SCLC), with promising results in combination with chemotherapy.
4.3 Hematological Malignancies
CAR T-cell therapy has shown remarkable success in treating hematological malignancies, particularly B-cell lymphomas and ALL. Several CAR T-cell products have been approved by the FDA for the treatment of relapsed or refractory B-cell lymphomas and ALL [2]. These CAR T-cell therapies target the CD19 antigen on B cells. CAR T-cell therapy is associated with significant toxicities, such as CRS and neurotoxicity, which require careful monitoring and management. Immunotherapy is also being investigated in other hematological malignancies, such as multiple myeloma and acute myeloid leukemia (AML), with promising results.
4.4 Other Cancers
Immunotherapy has also shown efficacy in a variety of other cancers, including kidney cancer, bladder cancer, head and neck cancer, Hodgkin lymphoma, and Merkel cell carcinoma. Anti-PD-1 antibodies and anti-PD-L1 antibodies have been approved for the treatment of these cancers. Immunotherapy is being investigated in many other cancers, with ongoing clinical trials evaluating the efficacy of various immunotherapeutic strategies.
Many thanks to our sponsor Esdebe who helped us prepare this research report.
5. Challenges and Limitations
Despite the remarkable progress in cancer immunotherapy, several challenges and limitations remain:
5.1 Resistance to Immunotherapy
Not all patients respond to immunotherapy, and some patients develop resistance after an initial response. Mechanisms of resistance to immunotherapy include: (1) loss of tumor-associated antigen expression, (2) downregulation of MHC molecules, (3) upregulation of immune checkpoints, (4) suppression of immune cell infiltration into the tumor, (5) activation of alternative signaling pathways that promote tumor growth and survival, and (6) development of immunosuppressive TME. Strategies to overcome resistance to immunotherapy include: (1) combination therapy with other immunotherapies or conventional therapies, (2) development of novel immunotherapeutic agents that target different immune pathways, (3) modulation of the TME to enhance immune cell infiltration and activity, and (4) personalized immunotherapy approaches based on the individual characteristics of the patient and the tumor.
5.2 Immune-Related Adverse Events
Immunotherapy can cause irAEs, which are inflammatory side effects that result from the activation of the immune system. IrAEs can affect any organ system and can range from mild to severe. Common irAEs include: (1) dermatitis, (2) colitis, (3) hepatitis, (4) pneumonitis, (5) endocrinopathies (e.g., thyroiditis, hypophysitis), and (6) neurologic toxicities. The management of irAEs typically involves the use of corticosteroids and other immunosuppressive agents. In severe cases, immunotherapy may need to be discontinued. Strategies to prevent or mitigate irAEs include: (1) patient selection, (2) early detection and management of irAEs, (3) use of biomarkers to predict irAEs, and (4) development of more targeted immunotherapies that minimize off-target effects.
5.3 Biomarkers for Predicting Response
Identifying biomarkers that can predict response to immunotherapy is a major challenge. PD-L1 expression on tumor cells has been used as a biomarker for predicting response to ICIs in some cancers, but it is not a perfect predictor. Other potential biomarkers include: (1) tumor mutational burden (TMB), (2) microsatellite instability (MSI), (3) immune cell infiltration into the tumor, and (4) gene expression profiles. Developing more accurate and reliable biomarkers is crucial for selecting patients who are most likely to benefit from immunotherapy and for avoiding unnecessary treatment in patients who are unlikely to respond. Ideally, biomarkers should be both predictive of response and prognostic of overall survival. Multi-omic approaches may be required to identify comprehensive biomarker signatures that accurately predict response to immunotherapy.
5.4 Cost and Accessibility
Immunotherapy can be expensive, which limits its accessibility to many patients. The cost of CAR T-cell therapy is particularly high. Efforts to reduce the cost of immunotherapy and improve its accessibility are needed. Strategies to reduce the cost of immunotherapy include: (1) development of more efficient manufacturing processes, (2) development of off-the-shelf immunotherapies, (3) negotiation of drug prices, and (4) expansion of insurance coverage. Furthermore, accessibility is not just limited to cost. Availability of specialized centers with the expertise to administer and manage immunotherapy-related toxicities is crucial.
Many thanks to our sponsor Esdebe who helped us prepare this research report.
6. Future Directions
The field of cancer immunotherapy is rapidly evolving, with numerous promising avenues for future research and development:
6.1 Combination Therapies
Combining immunotherapy with other therapies, such as chemotherapy, radiation therapy, targeted therapy, and other immunotherapies, is a promising strategy to improve treatment efficacy. Combination therapies can overcome resistance mechanisms, enhance immune cell infiltration into the tumor, and stimulate synergistic anti-tumor responses. Clinical trials are evaluating various combination therapies, with encouraging results in several cancers. For example, combining ICIs with chemotherapy has shown improved efficacy in lung cancer and other cancers. Combining ICIs with targeted therapies has shown promise in melanoma and other cancers. Combining different immunotherapies, such as ICIs with oncolytic viruses or cancer vaccines, is also being investigated. Rationally designed combination therapies, based on a deep understanding of the underlying mechanisms of action and resistance, are needed to maximize treatment efficacy and minimize toxicity.
6.2 Personalized Immunotherapy
Personalized immunotherapy involves tailoring immunotherapy treatment to the individual characteristics of the patient and the tumor. This approach takes into account factors such as: (1) tumor mutational burden, (2) microsatellite instability, (3) immune cell infiltration into the tumor, (4) gene expression profiles, and (5) patient’s immune status. Personalized immunotherapy strategies include: (1) neoantigen-based vaccines, which target tumor-specific mutations, (2) adoptive cell therapies engineered to target patient-specific tumor-associated antigens, and (3) combination therapies selected based on the individual characteristics of the patient and the tumor. Advances in genomics, proteomics, and bioinformatics are enabling the development of more sophisticated personalized immunotherapy approaches.
6.3 Novel Immunotherapeutic Targets
Identifying novel immunotherapeutic targets is crucial for expanding the applicability of immunotherapy to a broader range of cancers and patients. Emerging immunotherapeutic targets include: (1) inhibitory receptors on immune cells beyond CTLA-4 and PD-1, (2) stimulatory receptors on immune cells, (3) molecules involved in immune cell trafficking and infiltration into the tumor, (4) molecules that regulate the TME, and (5) tumor-associated antigens that are widely expressed on cancer cells. Developing novel immunotherapeutic agents that target these pathways is a major focus of current research.
6.4 Overcoming Immunosuppression
Overcoming immunosuppression in the TME is a major challenge in cancer immunotherapy. Strategies to overcome immunosuppression include: (1) targeting immunosuppressive cells, such as Tregs and MDSCs, (2) blocking immunosuppressive cytokines, such as TGF-β and IL-10, (3) enhancing the activity of immune cells, such as T cells and NK cells, and (4) modulating the TME to promote immune cell infiltration and activity. Preclinical studies and clinical trials are evaluating various strategies to overcome immunosuppression and improve the efficacy of immunotherapy.
6.5 Next-Generation CAR T-Cell Therapies
Next-generation CAR T-cell therapies are being developed to improve the efficacy and safety of CAR T-cell therapy. These include: (1) CAR T cells with enhanced signaling domains, (2) CAR T cells that express multiple CARs, (3) CAR T cells that are resistant to immunosuppression, (4) CAR T cells that can traffic to and penetrate solid tumors, (5) allogeneic CAR T cells derived from healthy donors, and (6) CAR NK cell therapies. These next-generation CAR T-cell therapies have the potential to expand the applicability of CAR T-cell therapy to a broader range of cancers and patients.
Many thanks to our sponsor Esdebe who helped us prepare this research report.
7. Conclusion
Immunotherapy has emerged as a transformative approach to cancer treatment, offering the potential for durable responses and long-term survival. Immune checkpoint inhibitors and adoptive cell therapies have revolutionized the treatment of several malignancies. However, challenges remain, including resistance to immunotherapy, immune-related adverse events, and the need for biomarkers to predict response. Future directions include the development of combination therapies, personalized immunotherapy approaches, novel immunotherapeutic targets, and next-generation CAR T-cell therapies. Further research is needed to overcome existing limitations and unlock the full potential of immunotherapy for a broader range of cancers and patients. The future of cancer treatment will likely involve a combination of immunotherapy and other therapies, tailored to the individual characteristics of the patient and the tumor.
Many thanks to our sponsor Esdebe who helped us prepare this research report.
References
[1] Hodi, F. S., et al. “Improved survival with ipilimumab in patients with metastatic melanoma.” New England Journal of Medicine 363.8 (2010): 711-723.
[2] June, C. H., et al. “CAR T cell therapy for human cancer.” Science 359.6382 (2018): 1361-1367.
[3] Rosenberg, S. A., et al. “Durable complete responses in heavily pretreated patients with metastatic cancer using T-cell transfer immunotherapy.” Clinical Cancer Research 17.13 (2011): 4550-4557.
[4] Andtbacka, R. H. I., et al. “Oncolytic virus talimogene laherparepvec improves durable response rate in patients with advanced melanoma.” Journal of Clinical Oncology 33.25 (2015): 2780-2788.
[5] Kantoff, P. W., et al. “Sipuleucel-T immunotherapy for castration-resistant prostate cancer.” New England Journal of Medicine 363.5 (2010): 411-422.
[6] Rosenberg, S. A., et al. “Experience with the use of high-dose interleukin-2 in the treatment of 652 cancer patients.” Annals of Surgery 216.4 (1992): 456.
[7] Gandhi, L., et al. “Pembrolizumab plus chemotherapy in metastatic non–small-cell lung cancer.” New England Journal of Medicine 378.22 (2018): 2078-2092.
This report highlights the incredible potential of personalized immunotherapy. The ability to tailor treatments based on individual tumor characteristics offers a promising path toward more effective and targeted cancer therapies. Further research in this area could significantly improve patient outcomes.
Thanks for your comment! I agree, the promise of personalized immunotherapy is truly exciting. It’s encouraging to see how tailoring treatments to individual tumor profiles can lead to more effective outcomes. The ongoing research into identifying predictive biomarkers is crucial to broaden access to this form of treatment.
Editor: MedTechNews.Uk
Thank you to our Sponsor Esdebe