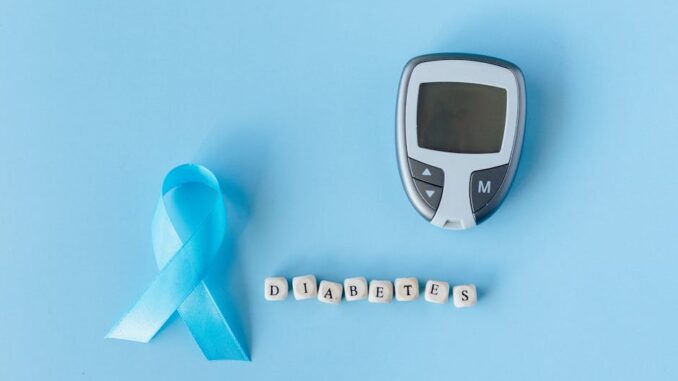
Abstract
Insulin resistance (IR), a state of impaired cellular response to insulin, is a pervasive metabolic abnormality implicated in a wide spectrum of diseases, most notably type 2 diabetes mellitus (T2DM) and cardiovascular disease (CVD). While the association of IR with these conditions is well-established in older populations, its role in promoting premature heart damage, especially in younger individuals, has garnered increasing attention. This research report provides a comprehensive review of the multifaceted aspects of IR, encompassing its intricate cellular and molecular mechanisms, the spectrum of clinical manifestations, diagnostic approaches, and both lifestyle and pharmacological interventions. The report also delves into the complexities of varying degrees of IR and their implications for long-term health outcomes, with a particular emphasis on cardiovascular risk. Furthermore, we critically examine the current understanding of the link between IR and premature CVD, highlighting potential research gaps and future directions for improving prevention and treatment strategies.
Many thanks to our sponsor Esdebe who helped us prepare this research report.
1. Introduction
Insulin, a peptide hormone secreted by pancreatic β-cells, plays a pivotal role in regulating glucose homeostasis and mediating a multitude of metabolic processes, including glucose uptake, glycogen synthesis, lipogenesis, and protein synthesis. Insulin resistance (IR) is a pathophysiological state characterized by a diminished responsiveness of target tissues, primarily skeletal muscle, liver, and adipose tissue, to the effects of insulin. Consequently, higher concentrations of insulin are required to achieve the same biological effect on glucose disposal. Initially, the pancreas compensates for IR by increasing insulin secretion, leading to hyperinsulinemia. This compensatory mechanism can maintain normal glucose tolerance for a considerable period. However, prolonged hyperinsulinemia eventually leads to β-cell dysfunction and a decline in insulin secretion, culminating in impaired glucose tolerance and, ultimately, overt T2DM [1].
Beyond its well-established role in the pathogenesis of T2DM, IR is increasingly recognized as a key driver of CVD, independent of glycemic control. The constellation of metabolic abnormalities associated with IR, including dyslipidemia (elevated triglycerides and low HDL-cholesterol), hypertension, and inflammation, collectively contribute to an increased risk of atherosclerosis and subsequent cardiovascular events [2]. Furthermore, evidence suggests that IR can directly impair endothelial function, promote vascular smooth muscle proliferation, and exacerbate myocardial dysfunction [3].
The rising prevalence of obesity and sedentary lifestyles, particularly among younger populations, has led to a concerning increase in the incidence of IR and its associated complications, including premature CVD. This report aims to provide a comprehensive overview of the current understanding of IR, encompassing its underlying mechanisms, diagnostic challenges, and therapeutic strategies, with a particular focus on its role in promoting premature heart damage. We will also address the complexities of varying degrees of IR and their long-term health implications, highlighting potential research gaps and future directions for improving prevention and treatment strategies.
Many thanks to our sponsor Esdebe who helped us prepare this research report.
2. Mechanisms of Insulin Resistance
The molecular mechanisms underlying IR are complex and multifaceted, involving a combination of genetic predisposition, environmental factors, and cellular dysfunction. The primary target tissues of insulin, namely skeletal muscle, liver, and adipose tissue, exhibit distinct pathways leading to impaired insulin signaling.
2.1 Skeletal Muscle
Skeletal muscle is the primary site of insulin-mediated glucose disposal. In individuals with IR, several factors contribute to impaired glucose uptake in muscle cells. These include [4]:
- Reduced Insulin Receptor Substrate (IRS) phosphorylation: Insulin binds to its receptor on the cell surface, leading to activation of the tyrosine kinase domain and subsequent phosphorylation of IRS proteins. In IR, defects in IRS phosphorylation impair downstream signaling.
- Impaired Phosphatidylinositol 3-Kinase (PI3K) activation: IRS phosphorylation activates PI3K, a crucial enzyme involved in glucose transporter 4 (GLUT4) translocation to the cell membrane. Reduced PI3K activity limits GLUT4-mediated glucose uptake.
- Dysregulation of GLUT4 Trafficking: GLUT4 is the primary glucose transporter responsible for insulin-stimulated glucose uptake in muscle cells. In IR, impaired GLUT4 trafficking to the cell membrane reduces glucose transport capacity.
- Intramyocellular Lipid Accumulation: Accumulation of lipids, such as diacylglycerols (DAGs) and ceramides, within muscle cells can interfere with insulin signaling by activating serine kinases that inhibit IRS phosphorylation.
- Mitochondrial Dysfunction: Impaired mitochondrial function and reduced oxidative capacity contribute to lipid accumulation and further exacerbate IR in skeletal muscle.
2.2 Liver
The liver plays a critical role in regulating glucose production and storage. In IR, the liver becomes less responsive to insulin’s suppressive effects on hepatic glucose production (HGP), leading to increased glucose output and hyperglycemia. Key mechanisms contributing to hepatic IR include [5]:
- Impaired Insulin Signaling: Similar to skeletal muscle, defects in IRS phosphorylation and PI3K activation contribute to impaired insulin signaling in the liver.
- Increased Glucagon Sensitivity: IR is often associated with increased sensitivity to glucagon, a hormone that stimulates HGP. This imbalance further exacerbates hyperglycemia.
- Hepatic Lipid Accumulation (NAFLD): Non-alcoholic fatty liver disease (NAFLD), characterized by excessive lipid accumulation in the liver, is strongly associated with IR. Hepatic steatosis promotes inflammation and oxidative stress, further impairing insulin signaling.
- Activation of Gluconeogenic Enzymes: Insulin resistance leads to failure to suppress key enzymes involved in gluconeogenesis, such as phosphoenolpyruvate carboxykinase (PEPCK) and glucose-6-phosphatase (G6Pase), increasing glucose production.
2.3 Adipose Tissue
Adipose tissue is not only a storage depot for triglycerides but also an active endocrine organ, secreting a variety of hormones and cytokines, collectively known as adipokines. In IR, adipose tissue dysfunction contributes significantly to systemic metabolic dysregulation. Key mechanisms include [6]:
- Adipokine Dysregulation: IR is associated with altered adipokine secretion, including decreased production of adiponectin (an insulin-sensitizing hormone) and increased production of pro-inflammatory cytokines, such as tumor necrosis factor-alpha (TNF-α) and interleukin-6 (IL-6). These cytokines promote systemic inflammation and contribute to IR in other tissues.
- Lipolysis and Free Fatty Acid (FFA) Release: IR promotes lipolysis in adipose tissue, leading to increased release of FFAs into the circulation. Elevated FFA levels contribute to IR in skeletal muscle and liver by interfering with insulin signaling and promoting lipid accumulation.
- Adipocyte Hypertrophy and Inflammation: Adipocyte hypertrophy (enlargement of fat cells) is associated with increased inflammation and impaired insulin signaling in adipose tissue. This creates a vicious cycle, further exacerbating IR.
2.4 Systemic Inflammation
A growing body of evidence suggests that chronic, low-grade inflammation plays a crucial role in the development of IR. Inflammatory cytokines, such as TNF-α and IL-6, activate signaling pathways that interfere with insulin signaling and promote IR in target tissues. The sources of inflammation are diverse, including adipose tissue, immune cells, and the gut microbiota [7].
- Adipose Tissue Inflammation: Visceral adipose tissue is a major source of inflammatory cytokines, contributing to systemic inflammation and IR.
- Immune Cell Activation: Activation of immune cells, such as macrophages and T cells, in response to metabolic stress and inflammation can contribute to IR.
- Gut Microbiota Dysbiosis: Alterations in the composition and function of the gut microbiota can lead to increased intestinal permeability and translocation of bacterial products into the circulation, triggering systemic inflammation and IR.
Many thanks to our sponsor Esdebe who helped us prepare this research report.
3. Insulin Resistance and Cardiovascular Disease
IR is strongly associated with an increased risk of CVD, independent of its role in T2DM. The mechanisms linking IR to CVD are complex and involve a combination of metabolic, hemodynamic, and inflammatory factors [8].
3.1 Metabolic Dyslipidemia
IR is characterized by a distinctive lipid profile, including elevated triglycerides, low HDL-cholesterol, and increased levels of small, dense LDL particles. This dyslipidemia is highly atherogenic and contributes to the development of atherosclerosis. The increased triglyceride levels are a consequence of increased VLDL production in the liver due to hepatic insulin resistance. The low HDL-cholesterol is in part due to the exchange of triglycerides from VLDL particles for cholesteryl ester from HDL particles, resulting in triglyceride-rich HDL particles which are readily cleared from circulation.
3.2 Hypertension
IR is frequently associated with hypertension. Several mechanisms contribute to this association, including:
- Sodium Retention: Insulin promotes sodium reabsorption in the kidneys. In IR, the kidneys become more sensitive to insulin’s effects on sodium retention, leading to increased blood volume and hypertension.
- Sympathetic Nervous System Activation: IR is associated with increased sympathetic nervous system activity, which can elevate blood pressure.
- Endothelial Dysfunction: IR impairs endothelial function, reducing the production of nitric oxide (NO), a potent vasodilator. This can lead to vasoconstriction and hypertension.
3.3 Endothelial Dysfunction
Endothelial dysfunction, characterized by impaired vasodilation and increased permeability of the endothelium, is an early event in the development of atherosclerosis. IR contributes to endothelial dysfunction through several mechanisms, including:
- Reduced NO Production: IR impairs insulin signaling in endothelial cells, reducing NO production.
- Increased Oxidative Stress: IR is associated with increased oxidative stress, which inactivates NO and promotes endothelial dysfunction.
- Inflammation: Inflammatory cytokines contribute to endothelial dysfunction by promoting endothelial cell activation and adhesion molecule expression.
3.4 Inflammation
Chronic, low-grade inflammation plays a crucial role in the pathogenesis of atherosclerosis. Inflammatory cytokines, such as TNF-α and IL-6, promote endothelial cell activation, leukocyte recruitment, and plaque formation. IR contributes to inflammation through several mechanisms, including:
- Adipokine Dysregulation: Dysregulation of adipokine secretion, particularly increased production of pro-inflammatory cytokines, contributes to systemic inflammation.
- Activation of Inflammatory Signaling Pathways: IR activates inflammatory signaling pathways in various tissues, including adipose tissue, liver, and immune cells.
3.5 Premature CVD
The accelerating rate of IR in younger populations, driven by factors such as sedentary lifestyles and high-calorie diets, is leading to an alarming increase in premature CVD. This phenomenon is particularly concerning because the long-term exposure to IR and its associated metabolic abnormalities can lead to more severe and difficult-to-manage CVD in later life. Studies have shown that individuals with IR, even in the absence of overt T2DM, have a significantly increased risk of developing coronary artery disease, stroke, and heart failure at a younger age [9]. This underscores the importance of early detection and intervention to prevent or delay the onset of premature CVD in individuals with IR.
Many thanks to our sponsor Esdebe who helped us prepare this research report.
4. Diagnosis of Insulin Resistance
Several methods are available for assessing insulin resistance, ranging from simple clinical measurements to more complex laboratory techniques. However, there is no single gold standard for diagnosing IR, and the choice of method depends on the clinical context and the resources available [10].
4.1 Clinical Assessment
Clinical assessment can provide valuable clues regarding the presence of IR. Risk factors associated with IR include obesity (particularly abdominal obesity), family history of T2DM, hypertension, dyslipidemia, and history of gestational diabetes. Physical examination may reveal signs of acanthosis nigricans, a skin condition characterized by dark, velvety patches in skin folds, which is often associated with IR. However, clinical assessment alone is not sufficient for diagnosing IR, and laboratory testing is usually required.
4.2 Laboratory Tests
- Fasting Plasma Glucose and Insulin: Measuring fasting plasma glucose and insulin levels can provide an estimate of IR. The homeostasis model assessment of insulin resistance (HOMA-IR) is a commonly used index calculated from fasting glucose and insulin levels:
- HOMA-IR = (Fasting Glucose (mg/dL) x Fasting Insulin (μU/mL)) / 405
- A HOMA-IR value above a certain threshold (typically >2.5) suggests IR. However, HOMA-IR is influenced by both insulin resistance and β-cell function, and its accuracy may be limited in individuals with impaired β-cell function.
- Oral Glucose Tolerance Test (OGTT): The OGTT involves measuring plasma glucose and insulin levels at baseline and at specific intervals after ingestion of a glucose load (typically 75 grams). The OGTT can provide more detailed information about glucose tolerance and insulin sensitivity than fasting measurements. The insulin sensitivity index derived from the OGTT (ISI-OGTT) is a more accurate measure of insulin sensitivity than HOMA-IR.
- Hyperinsulinemic-Euglycemic Clamp: The hyperinsulinemic-euglycemic clamp is considered the gold standard for measuring insulin sensitivity. This technique involves infusing insulin at a constant rate while maintaining euglycemia by adjusting the glucose infusion rate. The glucose disposal rate (GDR) during the clamp is a direct measure of insulin sensitivity. However, the hyperinsulinemic-euglycemic clamp is a complex and time-consuming procedure, and it is typically used only in research settings.
- Other Biomarkers: Several other biomarkers have been associated with IR, including adipokines (e.g., adiponectin, leptin), inflammatory markers (e.g., TNF-α, IL-6, C-reactive protein), and lipid metabolites (e.g., FFAs, triglycerides). However, these biomarkers are not routinely used for diagnosing IR in clinical practice.
4.3 Strengths and Limitations of Diagnostic Methods
Each diagnostic method for IR has its strengths and limitations. Clinical assessment is simple and non-invasive but lacks sensitivity and specificity. HOMA-IR is a convenient and widely used index, but it is influenced by β-cell function and may not be accurate in all individuals. The OGTT provides more detailed information about glucose tolerance and insulin sensitivity, but it is more time-consuming than fasting measurements. The hyperinsulinemic-euglycemic clamp is the gold standard for measuring insulin sensitivity, but it is complex and expensive. The choice of diagnostic method depends on the clinical context, the resources available, and the specific research question.
Many thanks to our sponsor Esdebe who helped us prepare this research report.
5. Therapeutic Strategies for Insulin Resistance
The primary goal of treating IR is to improve insulin sensitivity and prevent or delay the onset of T2DM and CVD. Therapeutic strategies for IR include lifestyle interventions and pharmacological treatments [11].
5.1 Lifestyle Interventions
Lifestyle interventions, including dietary modifications and regular physical activity, are the cornerstone of IR management.
- Dietary Modifications:
- Weight Loss: Weight loss, even modest weight loss (5-10% of body weight), can significantly improve insulin sensitivity. Weight loss can be achieved through calorie restriction, reduced intake of saturated and trans fats, and increased consumption of fruits, vegetables, and whole grains.
- Low-Glycemic Index Diet: A low-glycemic index diet, which emphasizes foods that are slowly digested and absorbed, can help to reduce postprandial glucose and insulin levels.
- Mediterranean Diet: The Mediterranean diet, which is rich in fruits, vegetables, whole grains, olive oil, and fish, has been shown to improve insulin sensitivity and reduce the risk of CVD.
- Physical Activity:
- Aerobic Exercise: Regular aerobic exercise, such as brisk walking, running, or swimming, improves insulin sensitivity and reduces the risk of CVD. Aim for at least 150 minutes of moderate-intensity aerobic exercise per week.
- Resistance Training: Resistance training, such as weightlifting, can increase muscle mass and improve insulin sensitivity. Include resistance training exercises at least two days per week.
- Combined Aerobic and Resistance Training: Combining aerobic and resistance training may provide the greatest benefits for improving insulin sensitivity and reducing the risk of CVD.
5.2 Pharmacological Treatments
Several pharmacological agents can improve insulin sensitivity and reduce the risk of T2DM and CVD in individuals with IR. However, these medications should be used in conjunction with lifestyle interventions, not as a replacement for them.
- Metformin: Metformin is a biguanide that reduces HGP and improves insulin sensitivity in the liver and skeletal muscle. Metformin is a commonly used first-line medication for T2DM and has been shown to reduce the risk of CVD.
- Thiazolidinediones (TZDs): TZDs, such as pioglitazone, are PPARγ agonists that improve insulin sensitivity in adipose tissue, liver, and skeletal muscle. TZDs can be effective in improving glycemic control, but they are associated with side effects such as weight gain, fluid retention, and increased risk of heart failure.
- Glucagon-Like Peptide-1 (GLP-1) Receptor Agonists: GLP-1 receptor agonists, such as exenatide and liraglutide, stimulate insulin secretion, suppress glucagon secretion, and slow gastric emptying. GLP-1 receptor agonists can improve glycemic control and promote weight loss. Some GLP-1 receptor agonists have been shown to reduce the risk of CVD in clinical trials.
- Sodium-Glucose Cotransporter-2 (SGLT2) Inhibitors: SGLT2 inhibitors, such as canagliflozin and empagliflozin, reduce glucose reabsorption in the kidneys, leading to increased glucose excretion in the urine. SGLT2 inhibitors can improve glycemic control and promote weight loss. Some SGLT2 inhibitors have been shown to reduce the risk of CVD and heart failure in clinical trials.
5.3 Emerging Therapies
Several novel therapies are being investigated for the treatment of IR, including:
- Farnesoid X Receptor (FXR) Agonists: FXR agonists are bile acid receptors that improve glucose and lipid metabolism. Obeticholic acid, an FXR agonist, has been approved for the treatment of primary biliary cholangitis and is being investigated for the treatment of NAFLD and T2DM.
- Fibroblast Growth Factor 21 (FGF21) Analogs: FGF21 is a hormone that improves glucose and lipid metabolism and promotes weight loss. FGF21 analogs are being developed for the treatment of T2DM and obesity.
- Gut Microbiota Modulation: Modulation of the gut microbiota, through dietary interventions or fecal microbiota transplantation, is being investigated as a potential therapeutic strategy for IR and metabolic disorders.
Many thanks to our sponsor Esdebe who helped us prepare this research report.
6. Varying Degrees of Insulin Resistance and Long-Term Health Implications
The severity of IR can vary significantly among individuals, ranging from mild IR to severe IR. The degree of IR is associated with different metabolic profiles and long-term health implications. Individuals with mild IR may have only slightly elevated glucose and insulin levels and may not experience any noticeable symptoms. However, they may still be at increased risk of developing T2DM and CVD over time. Individuals with severe IR may have markedly elevated glucose and insulin levels and may experience symptoms such as fatigue, frequent urination, and increased thirst. They are at high risk of developing T2DM, CVD, and other complications [12].
- Compensatory Hyperinsulinemia: In the early stages of IR, the pancreas compensates by increasing insulin secretion to maintain normal glucose tolerance. This compensatory hyperinsulinemia can mask the presence of IR and delay diagnosis. However, prolonged hyperinsulinemia can lead to β-cell dysfunction and eventual failure.
- Impaired Glucose Tolerance (IGT): As IR progresses, the pancreas may not be able to maintain sufficient insulin secretion to compensate for the reduced insulin sensitivity. This leads to IGT, characterized by elevated glucose levels after meals. IGT is a strong predictor of future development of T2DM.
- Type 2 Diabetes Mellitus (T2DM): Eventually, β-cell dysfunction progresses to the point where insulin secretion is insufficient to maintain normal glucose levels, leading to T2DM. T2DM is associated with a high risk of macrovascular and microvascular complications.
- Cardiovascular Disease (CVD): IR is a major risk factor for CVD, even in the absence of T2DM. The metabolic abnormalities associated with IR, including dyslipidemia, hypertension, and inflammation, contribute to the development of atherosclerosis and increase the risk of coronary artery disease, stroke, and heart failure.
- Non-Alcoholic Fatty Liver Disease (NAFLD): NAFLD is strongly associated with IR. Hepatic steatosis promotes inflammation and oxidative stress, further impairing insulin signaling and increasing the risk of liver damage.
- Polycystic Ovary Syndrome (PCOS): PCOS is a common endocrine disorder in women of reproductive age. IR is a key feature of PCOS and contributes to the development of hyperandrogenism and ovulatory dysfunction.
- Cancer: Emerging evidence suggests that IR may be associated with an increased risk of certain types of cancer, including colon cancer, breast cancer, and endometrial cancer. Insulin and insulin-like growth factor-1 (IGF-1) can stimulate cell proliferation and inhibit apoptosis, promoting tumor growth.
Many thanks to our sponsor Esdebe who helped us prepare this research report.
7. Conclusion
Insulin resistance is a pervasive metabolic abnormality with far-reaching health implications. It is a key driver of T2DM and CVD, and its increasing prevalence, particularly among younger populations, is a cause for concern. Understanding the complex mechanisms underlying IR, developing accurate diagnostic methods, and implementing effective therapeutic strategies are crucial for preventing or delaying the onset of IR-related complications. Lifestyle interventions, including dietary modifications and regular physical activity, are the cornerstone of IR management. Pharmacological treatments, such as metformin, TZDs, GLP-1 receptor agonists, and SGLT2 inhibitors, can improve insulin sensitivity and reduce the risk of T2DM and CVD. Further research is needed to identify novel therapeutic targets and develop more effective strategies for preventing and treating IR and its associated complications, especially premature CVD.
Many thanks to our sponsor Esdebe who helped us prepare this research report.
References
[1] Kahn, S. E., Hull, R. L., & Utzschneider, K. M. (2006). Mechanisms linking obesity to insulin resistance and type 2 diabetes. Nature, 444(7121), 840-846.
[2] Reaven, G. M. (2005). Insulin resistance, the insulin resistance syndrome, and cardiovascular disease. Panminerva Medica, 47(4), 201-210.
[3] Muniyappa, R., & Sowers, J. R. (2013). Insulin resistance in cardiovascular disease. Endocrine Reviews, 34(4), 575-592.
[4] DeFronzo, R. A., Triplitt, C. L., & Abdul-Ghani, M. (2021). Pathophysiologic mechanisms of type 2 diabetes: insulin resistance, beta-cell dysfunction and alpha-cell dysfunction. American Journal of Therapeutics, 28(2), e109-e126.
[5] Samuel, V. T., & Shulman, G. I. (2018). Mechanisms for insulin resistance: common threads and missing links. Cell, 175(3), 355-369.
[6] Guilherme, A., Virbasius, J. V., Puri, V., & Czech, M. P. (2008). Adipocyte dysfunctions linking obesity to insulin resistance and type 2 diabetes. Nature Reviews Molecular Cell Biology, 9(5), 367-377.
[7] Hotamisligil, G. S. (2008). Inflammation and metabolic disease. Nature, 462(7278), 954-961.
[8] Tentolouris, N., Vlachou, V., Tzeravini, E., Zacharoulis, A., & Katsilambros, N. (2006). Insulin resistance: the key feature of metabolic syndrome. Hellenic Journal of Cardiology, 47(4), 320-329.
[9] Laakso, M. (1993). Insulin resistance and coronary heart disease. Current Opinion in Lipidology, 4(4), 255-260.
[10] Matthews, D. R., Hosker, J. P., Rudenski, A. S., Naylor, B. A., Treacher, D. F., & Turner, R. C. (1985). Homeostasis model assessment: insulin resistance and beta-cell function from fasting plasma glucose and insulin concentrations in man. Diabetologia, 28(7), 412-419.
[11] Knowler, W. C., Barrett-Connor, E., Fowler, S. E., Hamman, R. F., Lachin, J. M., Walker, E. A., & Nathan, D. M. (2002). Reduction in the incidence of type 2 diabetes with lifestyle intervention or metformin. New England Journal of Medicine, 346(6), 393-403.
[12] Abdul-Ghani, M. A., Triplitt, C. L., DeFronzo, R. A., & Dagogo-Jack, S. (2021). Contributions of insulin resistance and beta-cell dysfunction to incident diabetes: a longitudinal study. Diabetes, 70(5), 1121-1130.
Wow, that’s a lot of science! So, if I understand correctly, my love of donuts might be contributing to premature heart damage? Guess I’ll just have to start blaming my sweet tooth on Esdebe from now on!
Thanks for reading! You’ve hit on a key point – diet plays a significant role. While blaming Esdebe might be fun, perhaps exploring healthier alternatives that satisfy your sweet cravings could be a win-win. Finding that balance is what it’s all about!
Editor: MedTechNews.Uk
Thank you to our Sponsor Esdebe
So, premature heart damage might be the *real* reason I can’t keep up with those Gen Z gym bunnies? Suddenly feeling less guilty about skipping leg day! Thanks for the insightful (and slightly terrifying) research!
That’s a great point! While skipping leg day might feel less guilty now, remember that physical activity, even in moderation, plays a crucial role in overall health and can combat the negative effects highlighted in the research. Finding activities you enjoy can make it sustainable and beneficial!
Editor: MedTechNews.Uk
Thank you to our Sponsor Esdebe
Given the rise of IR in younger populations, what are the most effective, scalable strategies for early screening and intervention, particularly in communities with limited access to healthcare resources?
That’s an excellent question! Exploring scalable solutions for early screening in underserved communities is crucial. Leveraging telehealth and mobile health technologies, combined with community-based education programs, could be a game-changer. What are your thoughts on incorporating AI-powered risk assessments for wider reach?
Editor: MedTechNews.Uk
Thank you to our Sponsor Esdebe