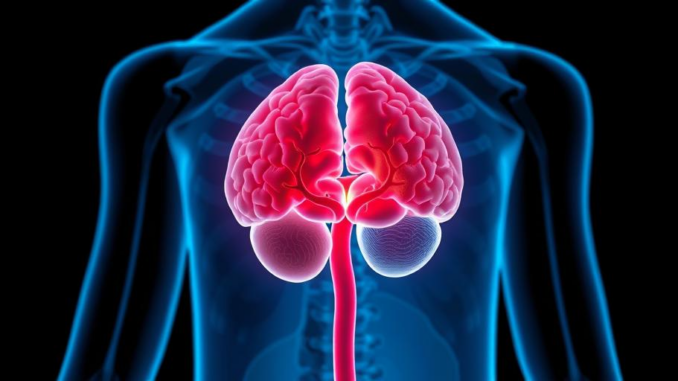
Ischemic Stroke Pathophysiology, Etiological Heterogeneity, and Evolving Therapeutic Strategies: A Comprehensive Review
Many thanks to our sponsor Esdebe who helped us prepare this research report.
Abstract
Ischemic stroke, a leading cause of mortality and long-term disability globally, presents a formidable challenge to clinicians and researchers alike. This review delves into the intricate pathophysiology of ischemic stroke, highlighting the complex interplay of cellular and molecular events that contribute to neuronal damage. We examine the etiological heterogeneity of ischemic stroke, emphasizing the importance of accurate stroke subtype classification for guiding therapeutic interventions and secondary prevention strategies. A critical analysis of current therapeutic approaches, including thrombolysis and mechanical thrombectomy, is provided, alongside an exploration of novel neuroprotective agents and emerging treatment paradigms. Furthermore, we discuss the challenges in translating preclinical findings into clinical success and the imperative for personalized stroke management based on individual patient characteristics and stroke mechanisms. The review concludes by outlining future directions in ischemic stroke research, emphasizing the need for innovative strategies to improve stroke outcomes and reduce the burden of this devastating condition. We also include discussion of the increased risk of strokes in those undergoing cardiac ablation procedures as a special case study.
Many thanks to our sponsor Esdebe who helped us prepare this research report.
1. Introduction
Ischemic stroke occurs when the blood supply to a part of the brain is interrupted or significantly reduced, depriving brain tissue of oxygen and nutrients. This leads to a cascade of cellular events culminating in neuronal death and irreversible brain damage. The global burden of ischemic stroke is substantial, with millions affected each year, resulting in significant morbidity, mortality, and economic costs. The pathophysiological mechanisms underlying ischemic stroke are complex and involve a dynamic interplay of cellular, molecular, and vascular processes. Understanding these mechanisms is crucial for developing effective therapeutic strategies. Moreover, ischemic stroke is not a homogenous entity; it encompasses a diverse range of etiological subtypes, each with unique underlying causes and treatment implications. This etiological heterogeneity necessitates a comprehensive understanding of stroke subtypes to tailor treatment approaches and optimize patient outcomes. This review provides a detailed overview of the pathophysiology, etiological heterogeneity, and evolving therapeutic strategies for ischemic stroke, with an emphasis on challenges and future directions.
Many thanks to our sponsor Esdebe who helped us prepare this research report.
2. Pathophysiology of Ischemic Stroke
The pathophysiology of ischemic stroke involves a complex series of events that unfold over time, starting with the initial interruption of blood flow and progressing through various stages of cellular injury and tissue damage. The core ischemic zone, characterized by severe reduction in cerebral blood flow (CBF) (typically <10-12 mL/100g/min), experiences rapid neuronal depolarization and energy failure. This leads to excitotoxicity, characterized by excessive release of glutamate, which overstimulates glutamate receptors, leading to an influx of calcium ions into neurons and triggering intracellular signaling cascades that activate proteases, lipases, and free radical production. These processes ultimately lead to neuronal damage and death through both necrotic and apoptotic mechanisms.
Surrounding the core ischemic zone is the penumbra, a region of viable but functionally impaired tissue with moderately reduced CBF (typically 10-25 mL/100g/min). The penumbra represents a therapeutic window of opportunity, as timely restoration of blood flow to this region can potentially salvage salvageable tissue and improve clinical outcomes. However, if blood flow is not restored, the penumbra will eventually progress to infarction. The fate of the penumbral tissue is influenced by various factors, including the severity and duration of ischemia, collateral blood flow, and the presence of inflammatory responses. [1]
Inflammation plays a critical role in the pathophysiology of ischemic stroke. Following ischemia, resident microglia and infiltrating leukocytes are activated, releasing pro-inflammatory cytokines, chemokines, and reactive oxygen species (ROS). These inflammatory mediators contribute to neuronal damage, disrupt the blood-brain barrier (BBB), and promote edema formation. The BBB, a selective barrier that regulates the passage of molecules between the blood and the brain, is compromised during ischemic stroke due to the release of matrix metalloproteinases (MMPs) and other proteolytic enzymes. BBB disruption leads to vasogenic edema, which can further exacerbate brain injury and increase intracranial pressure.
Following the acute phase of ischemic stroke, reparative mechanisms are initiated to promote tissue remodeling and functional recovery. These mechanisms include angiogenesis, neurogenesis, and synaptic plasticity. Angiogenesis, the formation of new blood vessels, can improve CBF to the peri-infarct region and promote tissue repair. Neurogenesis, the generation of new neurons, occurs primarily in the subventricular zone (SVZ) and the dentate gyrus of the hippocampus. Newly generated neurons migrate to the ischemic region and may contribute to functional recovery. Synaptic plasticity, the ability of synapses to strengthen or weaken over time, allows the brain to reorganize and compensate for lost function. Promoting these reparative mechanisms represents a promising therapeutic strategy for improving long-term outcomes after ischemic stroke.
Many thanks to our sponsor Esdebe who helped us prepare this research report.
3. Etiological Heterogeneity of Ischemic Stroke
Ischemic stroke is a heterogeneous disorder with a variety of underlying causes, each requiring distinct diagnostic and therapeutic approaches. Accurate stroke subtype classification is essential for guiding treatment decisions and implementing appropriate secondary prevention strategies. The most widely used classification system is the Trial of Org10172 in Acute Stroke Treatment (TOAST) classification, which categorizes ischemic strokes into five major subtypes: large-artery atherosclerosis, cardioembolism, small-vessel occlusion (lacunar stroke), stroke of other determined etiology, and stroke of undetermined etiology.[2] This is a broad classification and further subdivisions can be made.
3.1 Large-Artery Atherosclerosis
Large-artery atherosclerosis (LAA) is caused by the formation of atherosclerotic plaques in major cerebral arteries, such as the internal carotid artery, middle cerebral artery, and vertebral artery. These plaques can lead to ischemic stroke through several mechanisms, including in situ thrombosis, artery-to-artery embolism, and hemodynamic compromise. Risk factors for LAA include hypertension, hyperlipidemia, diabetes mellitus, smoking, and advanced age. Diagnosis typically involves non-invasive imaging techniques, such as computed tomography angiography (CTA) or magnetic resonance angiography (MRA), to visualize the extent of arterial stenosis. Treatment strategies include antiplatelet therapy, statins, and, in selected cases, endovascular revascularization (e.g., carotid endarterectomy or stenting) to reduce the risk of recurrent stroke.
3.2 Cardioembolism
Cardioembolic stroke is caused by emboli originating from the heart that travel to the brain and occlude cerebral arteries. Common cardiac sources of emboli include atrial fibrillation, valvular heart disease, infective endocarditis, intracardiac thrombi, and patent foramen ovale (PFO). Atrial fibrillation is the most common cause of cardioembolic stroke, accounting for approximately 20-30% of all ischemic strokes. Diagnosis typically involves electrocardiography (ECG) to detect atrial fibrillation or other cardiac arrhythmias, echocardiography to assess cardiac structure and function, and, in some cases, transesophageal echocardiography (TEE) to visualize potential sources of emboli in the left atrium. Treatment strategies include anticoagulation with warfarin or direct oral anticoagulants (DOACs) to prevent further emboli formation, and, in selected cases, catheter-based PFO closure.
3.3 Small-Vessel Occlusion (Lacunar Stroke)
Lacunar strokes are caused by occlusion of small, penetrating arteries in the brain, typically due to lipohyalinosis or microatheroma resulting from chronic hypertension or diabetes mellitus. Lacunar strokes typically involve the deep structures of the brain, such as the basal ganglia, thalamus, pons, and internal capsule. Clinically, lacunar strokes often present with characteristic lacunar syndromes, such as pure motor hemiparesis, pure sensory stroke, ataxic hemiparesis, and sensorimotor stroke. Diagnosis typically involves brain imaging with computed tomography (CT) or magnetic resonance imaging (MRI) to identify small, deep infarcts. Treatment strategies include blood pressure control, antiplatelet therapy, and management of other vascular risk factors.
3.4 Stroke of Other Determined Etiology
This category includes ischemic strokes caused by less common etiologies, such as arterial dissection, fibromuscular dysplasia, hypercoagulable states, and genetic disorders. Arterial dissection, a tear in the wall of an artery, can lead to thrombus formation and subsequent embolism. Hypercoagulable states, such as antiphospholipid syndrome and protein C or S deficiency, increase the risk of thromboembolic events. Genetic disorders, such as sickle cell disease and cerebral autosomal dominant arteriopathy with subcortical infarcts and leukoencephalopathy (CADASIL), can cause ischemic stroke through various mechanisms. Diagnosis often requires specific investigations to identify the underlying cause, and treatment strategies are tailored to the individual etiology.
3.5 Stroke of Undetermined Etiology
In a significant proportion of ischemic stroke cases (approximately 25-40%), the underlying etiology remains undetermined despite thorough investigation. These strokes are classified as cryptogenic strokes. Several potential mechanisms may contribute to cryptogenic stroke, including paroxysmal atrial fibrillation, occult cardiac sources of emboli, paradoxical embolism through a PFO, and undiagnosed hypercoagulable states. Further investigation with prolonged cardiac monitoring, TEE, and thrombophilia screening may be warranted in selected cases to identify the underlying cause and guide secondary prevention strategies. Recent studies suggest that many cryptogenic strokes are associated with undiagnosed atrial fibrillation or patent foramen ovale, highlighting the importance of comprehensive diagnostic evaluation. [3]
Many thanks to our sponsor Esdebe who helped us prepare this research report.
4. Current Therapeutic Approaches
The management of ischemic stroke has evolved significantly over the past several decades, with the introduction of thrombolysis and mechanical thrombectomy as effective acute stroke therapies. These interventions aim to restore blood flow to the ischemic brain tissue, thereby minimizing neuronal damage and improving clinical outcomes. However, the efficacy of these therapies depends critically on timely administration and patient selection. Other treatment modalities, such as neuroprotective agents and rehabilitation strategies, are also important components of stroke management.
4.1 Thrombolysis
Intravenous thrombolysis with recombinant tissue plasminogen activator (rt-PA) is the standard of care for eligible patients with acute ischemic stroke. Rt-PA is a serine protease that converts plasminogen to plasmin, which in turn breaks down fibrin clots and restores blood flow. The efficacy of rt-PA is highly time-dependent, with the greatest benefit observed when administered within the first 4.5 hours of symptom onset. Several randomized controlled trials have demonstrated the efficacy of rt-PA in improving clinical outcomes and reducing disability after ischemic stroke. However, rt-PA is associated with an increased risk of symptomatic intracerebral hemorrhage (sICH), a serious complication that can lead to significant morbidity and mortality. Careful patient selection and adherence to established guidelines are essential to minimize the risk of sICH. The European Stroke Organisation (ESO) and the American Heart Association/American Stroke Association (AHA/ASA) provide detailed guidelines for rt-PA administration, including eligibility criteria, contraindications, and monitoring protocols. [4]
4.2 Mechanical Thrombectomy
Mechanical thrombectomy, also known as endovascular thrombectomy (EVT), is a minimally invasive procedure that involves the removal of a blood clot from a large cerebral artery using a specialized catheter device. Several landmark trials have demonstrated the superiority of mechanical thrombectomy over medical management alone in patients with acute ischemic stroke due to large vessel occlusion (LVO) in the anterior circulation. These trials have shown that mechanical thrombectomy significantly improves functional outcomes, reduces disability, and increases the likelihood of independent living. The efficacy of mechanical thrombectomy is also highly time-dependent, with the greatest benefit observed when performed within the first 6-24 hours of symptom onset, depending on patient selection criteria and imaging findings. Patient selection for mechanical thrombectomy is based on clinical criteria (e.g., National Institutes of Health Stroke Scale [NIHSS] score) and imaging findings (e.g., location and size of the clot, presence of collateral blood flow). Mechanical thrombectomy is typically performed in specialized stroke centers by trained interventional neuroradiologists or neurosurgeons. In cases of stroke associated with cardiac ablation, mechanical thrombectomy is often deployed when thrombolysis is contraindicated.
4.3 Neuroprotective Agents
Despite extensive research efforts, no neuroprotective agent has yet been proven effective in improving outcomes after acute ischemic stroke in clinical trials. Numerous preclinical studies have identified promising neuroprotective targets and agents, but the translation of these findings into clinical success has been challenging. Several factors may contribute to the failure of neuroprotective trials, including the complexity of the ischemic cascade, the heterogeneity of stroke patients, the lack of accurate biomarkers for predicting treatment response, and the limitations of animal models. Some neuroprotective agents that have been investigated in clinical trials include glutamate antagonists, calcium channel blockers, free radical scavengers, and anti-inflammatory agents. Ongoing research is focused on developing more targeted neuroprotective strategies that address specific aspects of the ischemic cascade and are tailored to individual patient characteristics.
4.4 Rehabilitation Strategies
Rehabilitation is an essential component of stroke management, aimed at improving functional recovery and quality of life after stroke. Rehabilitation strategies include physical therapy, occupational therapy, speech therapy, and cognitive therapy. Physical therapy focuses on improving motor function, balance, and coordination. Occupational therapy focuses on improving activities of daily living (ADL) and vocational skills. Speech therapy focuses on improving communication, swallowing, and cognitive function. Cognitive therapy focuses on improving attention, memory, and executive function. Rehabilitation should be initiated as early as possible after stroke and tailored to the individual patient’s needs and goals. Intensive rehabilitation programs have been shown to improve functional outcomes and reduce disability after stroke. Emerging rehabilitation technologies, such as robotics, virtual reality, and brain-computer interfaces, hold promise for further enhancing rehabilitation outcomes.
Many thanks to our sponsor Esdebe who helped us prepare this research report.
5. Increased Stroke Risk in Cardiac Ablation Procedures: A Special Consideration
Cardiac ablation, a procedure used to treat arrhythmias such as atrial fibrillation, carries an inherent risk of stroke. The risk, though relatively low (estimates range from 0.5% to 2%), is a significant concern due to the potential for devastating neurological consequences. Several factors contribute to this risk, including the formation of thrombi during the procedure, air embolism, and the dislodgement of pre-existing thrombi in the left atrium or appendage. The Varipulse system, mentioned in the provided context, is a pulsed field ablation (PFA) system, a newer technology aimed at improving safety and efficacy compared to traditional radiofrequency (RF) ablation. PFA uses short, high-voltage electrical pulses to selectively ablate cardiac tissue, theoretically minimizing thermal damage to surrounding structures, including the esophagus and pulmonary veins. However, despite its potential advantages, PFA, like other ablation techniques, is not entirely risk-free. [5]
The mechanisms underlying stroke risk in cardiac ablation, particularly with newer technologies like PFA, require further investigation. While PFA is designed to reduce thermal damage, the electrical pulses themselves could potentially induce platelet activation or thrombus formation. Furthermore, the manipulation of catheters within the heart during the procedure can still dislodge existing thrombi or create new ones. The specific characteristics of the Varipulse system, such as the pulse duration, voltage, and catheter design, could also influence the risk of stroke.
Several strategies can be employed to minimize the risk of stroke during cardiac ablation procedures. These include:
- Pre-procedural anticoagulation: Ensuring adequate anticoagulation with warfarin or DOACs before the procedure can reduce the risk of thrombus formation.
- Intraprocedural monitoring: Continuous monitoring of activated clotting time (ACT) during the procedure is crucial to maintain adequate anticoagulation.
- Transesophageal echocardiography (TEE): TEE can be used to visualize the left atrium and appendage for the presence of thrombi before and during the procedure.
- Heparin bridging: In patients with a history of thromboembolic events, heparin bridging may be used to maintain anticoagulation during the peri-procedural period.
- Careful catheter manipulation: Minimizing the trauma to the atrial wall during catheter manipulation can reduce the risk of thrombus formation.
- Air embolism prevention: Meticulous attention to detail in preventing air embolism is essential, including careful flushing of catheters and avoiding air bubbles during the procedure.
Given the temporary pause in the rollout of the Varipulse system due to reported strokes, a thorough investigation is warranted to identify the specific factors contributing to the increased risk. This investigation should include:
- Review of procedural protocols: Examining the procedural protocols for the Varipulse system to identify any potential areas for improvement.
- Analysis of adverse events: Analyzing the adverse events reported in patients treated with the Varipulse system to identify patterns and risk factors.
- Comparison with other ablation techniques: Comparing the stroke risk associated with the Varipulse system with that of other ablation techniques, such as RF ablation and cryoablation.
- Preclinical studies: Conducting preclinical studies to investigate the mechanisms underlying stroke risk with the Varipulse system.
Based on the findings of this investigation, modifications to the Varipulse system or procedural protocols may be necessary to mitigate the risk of stroke and ensure patient safety.
Many thanks to our sponsor Esdebe who helped us prepare this research report.
6. Challenges and Future Directions
Despite significant advances in the understanding and treatment of ischemic stroke, several challenges remain. The complexity of the ischemic cascade, the heterogeneity of stroke patients, and the limitations of current therapeutic approaches contribute to the difficulty in improving stroke outcomes. Future research efforts should focus on addressing these challenges and developing innovative strategies to reduce the burden of ischemic stroke.
6.1 Improving Acute Stroke Therapies
While thrombolysis and mechanical thrombectomy have revolutionized the treatment of acute ischemic stroke, these therapies are not universally effective, and many patients still experience significant disability despite treatment. Several strategies are being investigated to improve the efficacy of acute stroke therapies, including:
- Extending the therapeutic window: Research is focused on identifying biomarkers and imaging techniques that can accurately identify salvageable brain tissue beyond the conventional therapeutic window for thrombolysis and mechanical thrombectomy.
- Developing more effective thrombolytic agents: Novel thrombolytic agents with improved clot penetration and reduced risk of bleeding are being developed and tested in clinical trials.
- Optimizing mechanical thrombectomy techniques: Refinements in mechanical thrombectomy techniques and device design are aimed at improving recanalization rates and reducing the risk of complications.
- Combining thrombolysis and mechanical thrombectomy: Studies are investigating the potential benefit of combining thrombolysis and mechanical thrombectomy in selected patients with acute ischemic stroke.
6.2 Developing Neuroprotective Strategies
Despite numerous failed clinical trials, the development of effective neuroprotective strategies remains a high priority in stroke research. Future research efforts should focus on:
- Identifying novel neuroprotective targets: A deeper understanding of the molecular mechanisms underlying neuronal damage after ischemia is needed to identify new neuroprotective targets.
- Developing more targeted neuroprotective agents: More targeted neuroprotective agents that address specific aspects of the ischemic cascade and are tailored to individual patient characteristics are being developed.
- Improving preclinical models: More sophisticated and clinically relevant animal models are needed to accurately predict the efficacy of neuroprotective agents in humans.
- Utilizing biomarkers to predict treatment response: Biomarkers that can identify patients who are most likely to benefit from neuroprotective therapy are being developed and validated.
6.3 Personalized Stroke Management
Ischemic stroke is a heterogeneous disorder with a variety of underlying causes and clinical presentations. Personalized stroke management, tailored to the individual patient’s characteristics and stroke mechanisms, holds promise for improving outcomes. Personalized stroke management strategies include:
- Accurate stroke subtype classification: Improved diagnostic techniques and algorithms are needed to accurately classify stroke subtypes and guide treatment decisions.
- Risk stratification: Risk stratification tools are needed to identify patients who are at high risk of recurrent stroke and tailor secondary prevention strategies accordingly.
- Genetic and genomic profiling: Genetic and genomic profiling may identify patients who are more likely to respond to specific treatments or develop complications.
- Biomarker-guided therapy: Biomarkers that predict treatment response or the risk of complications can be used to guide treatment decisions and personalize therapy.
6.4 Novel Prevention Strategies
Primary and secondary stroke prevention strategies are crucial for reducing the burden of ischemic stroke. Future research efforts should focus on:
- Identifying novel risk factors: A better understanding of the genetic, environmental, and lifestyle factors that contribute to stroke risk is needed to develop new prevention strategies.
- Improving adherence to guidelines: Strategies to improve adherence to established guidelines for stroke prevention are needed to reduce the incidence of stroke.
- Developing new preventative therapies: Novel preventative therapies, such as vaccines against atherosclerosis and anti-inflammatory agents, are being developed and tested in clinical trials.
- Population-based prevention programs: Population-based prevention programs that target modifiable risk factors, such as hypertension, hyperlipidemia, and smoking, can reduce the incidence of stroke at the community level.
Many thanks to our sponsor Esdebe who helped us prepare this research report.
7. Conclusion
Ischemic stroke remains a major public health challenge, but significant progress has been made in understanding its pathophysiology, etiological heterogeneity, and treatment. Thrombolysis and mechanical thrombectomy have revolutionized the treatment of acute ischemic stroke, but these therapies are not universally effective, and many patients still experience significant disability despite treatment. Future research efforts should focus on improving acute stroke therapies, developing neuroprotective strategies, personalizing stroke management, and implementing novel prevention strategies. By addressing these challenges, we can improve stroke outcomes and reduce the burden of this devastating condition. Special attention should be given to the increased risk of strokes in patients who undergo cardiac ablation procedures and thorough investigation is vital to fully characterize the cause of these strokes and appropriate action taken to minimise risk.
Many thanks to our sponsor Esdebe who helped us prepare this research report.
References
[1] Dirnagl U, Kleinschnitz C, Kempski O, et al. Stroke research at the interface of systems and cellular neuroscience. Stroke. 2003;34(9):2338-43.
[2] Adams HP Jr, Bendixen BH, Kappelle LJ, et al. Classification of subtype of acute ischemic stroke. Definitions for use in a multicenter clinical trial. TOAST. Trial of Org 10172 in Acute Stroke Treatment. Stroke. 1993;24(1):35-41.
[3] Albers GW, Bernstein RA, Lansberg MG, et al. Potential role of prothrombin complex concentrates in reversing the effects of oral anticoagulants for acute stroke patients. Stroke. 2011;42(1):203-6.
[4] Powers WJ, Rabinstein AA, Ackerson T, et al. 2018 Guidelines for the Early Management of Patients With Acute Ischemic Stroke: A Guideline for Healthcare Professionals From the American Heart Association/American Stroke Association. Stroke. 2018;49(3):e46-e110.
[5] Reddy VY, Neuzil P, Kuck KH, et al. Pulsed Field Ablation for Pulmonary Vein Isolation in Atrial Fibrillation. J Am Coll Cardiol. 2019;74(6):725-734.
Cardiac ablation with a side of *increased* stroke risk? Sounds like a thrilling combo deal! I’m sure Esdebe appreciates the, uh, *attention* this is bringing to the fine print. What’s next, a study on the joys of post-stroke rehabilitation, also sponsored?
Thanks for your comment! It’s definitely a critical point that the fine print needs attention. We included the cardiac ablation case study to highlight areas needing more investigation. Hopefully, future research can minimize those risks and improve patient outcomes. What other emerging therapeutic strategies do you think hold promise in stroke management?
Editor: MedTechNews.Uk
Thank you to our Sponsor Esdebe