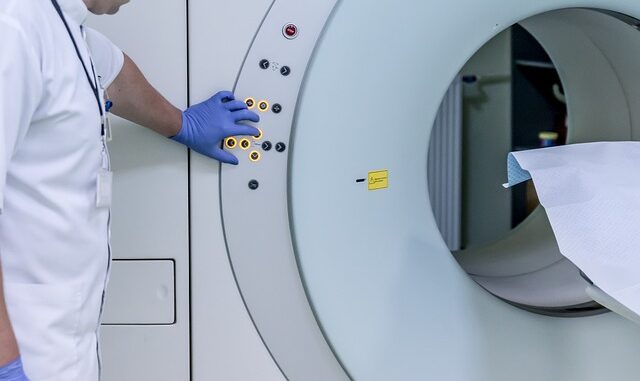
Magnetic Resonance Imaging: A Comprehensive Review of Applications, Advancements, and Future Directions
Abstract
Magnetic Resonance Imaging (MRI) has revolutionized medical diagnostics, offering unparalleled soft tissue contrast and versatility across numerous clinical applications. This review provides a comprehensive overview of MRI technology, encompassing its fundamental principles, diverse applications beyond cancer screening, key advancements in hardware and pulse sequences, the role of contrast agents, critical safety considerations, and emerging approaches to cost-effectiveness. Furthermore, we explore the transformative potential of artificial intelligence (AI) in enhancing MRI image analysis, interpretation, and overall workflow efficiency. We critically evaluate the strengths and limitations of current MRI techniques, highlighting areas ripe for future research and development to further expand the diagnostic and therapeutic impact of this powerful imaging modality. We contend that while MRI remains a relatively expensive imaging technique, the continued development of faster sequences, improved image reconstruction, and AI-assisted interpretation will further extend its scope and value to patient care, potentially leading to earlier and more accurate diagnoses, and improved treatment outcomes.
1. Introduction
Magnetic Resonance Imaging (MRI) has emerged as an indispensable tool in modern medicine, providing non-invasive, high-resolution anatomical and functional information. Unlike modalities relying on ionizing radiation (e.g., X-ray Computed Tomography (CT)), MRI utilizes strong magnetic fields and radio waves to generate detailed images of internal body structures. This characteristic makes MRI particularly advantageous for visualizing soft tissues, the central nervous system, and musculoskeletal system, where subtle contrast differences are crucial for diagnosis.
While the initial applications of MRI focused primarily on neuroimaging, the technology has rapidly expanded to encompass a broad range of clinical specialties, including cardiology, oncology, gastroenterology, and orthopedics. This expansion has been driven by continuous advancements in MRI hardware, pulse sequence design, and image reconstruction algorithms. These advancements have led to improved spatial and temporal resolution, faster scan times, and enhanced image quality.
This review aims to provide a comprehensive overview of MRI, covering its fundamental principles, clinical applications, technological advancements, safety considerations, and future directions. We will delve into the use of contrast agents and their impact on diagnostic accuracy. We will also explore the rapidly evolving role of artificial intelligence (AI) in MRI, with a particular focus on its potential to improve image analysis, interpretation, and overall clinical workflow. The ultimate goal is to present a contemporary and insightful perspective on the current state of MRI and its prospects for future development and application.
2. Fundamental Principles of MRI
At its core, MRI exploits the quantum mechanical property of nuclear spin. Atomic nuclei with an odd number of protons or neutrons possess a net angular momentum, generating a magnetic dipole moment. When placed in a strong external magnetic field (B0), these nuclei, primarily hydrogen protons (1H), align either with or against the field, creating a net magnetization vector parallel to B0. The application of a radiofrequency (RF) pulse at the Larmor frequency (the resonant frequency proportional to B0) excites these protons, causing them to tip away from the B0 axis. Upon cessation of the RF pulse, the protons relax back to their equilibrium state, emitting a signal that is detected by RF coils.
The relaxation process is characterized by two primary time constants: T1 and T2. T1 relaxation, also known as spin-lattice relaxation, describes the recovery of the longitudinal magnetization (Mz) towards its equilibrium value. T2 relaxation, or spin-spin relaxation, describes the decay of the transverse magnetization (Mxy) due to dephasing of the spins. These relaxation times are tissue-specific and form the basis for contrast in MRI images. T1-weighted images are typically obtained by using short repetition times (TR) and short echo times (TE), while T2-weighted images utilize longer TR and TE values. Intermediate values of TR and TE can also be used to generate proton density-weighted images.
Spatial encoding in MRI is achieved through the use of magnetic field gradients. These gradients linearly vary the magnetic field strength across the imaging volume, allowing for the spatial localization of the emitted RF signals. Frequency encoding is used to localize signals along one direction, while phase encoding is used to localize signals along another direction. The combination of frequency and phase encoding enables the reconstruction of a 2D or 3D image.
3. Diverse Clinical Applications of MRI
Beyond its well-established role in breast cancer screening and detection, MRI finds widespread application across a vast spectrum of clinical disciplines. Some notable examples include:
-
Neuroimaging: MRI is the gold standard for imaging the brain and spinal cord. It is used to diagnose a wide range of neurological conditions, including stroke, multiple sclerosis, Alzheimer’s disease, brain tumors, and spinal cord injuries. Functional MRI (fMRI) allows for the mapping of brain activity by detecting changes in blood flow associated with neuronal activity. Diffusion tensor imaging (DTI) provides information about the white matter tracts in the brain.
-
Cardiovascular Imaging: Cardiac MRI provides detailed anatomical and functional information about the heart. It is used to assess cardiac function, myocardial perfusion, and the presence of congenital heart defects. Magnetic resonance angiography (MRA) is used to visualize blood vessels and detect aneurysms, stenosis, and other vascular abnormalities.
-
Musculoskeletal Imaging: MRI is highly effective for visualizing the musculoskeletal system, including bones, joints, muscles, ligaments, and tendons. It is used to diagnose conditions such as arthritis, sports injuries, and tumors.
-
Abdominal and Pelvic Imaging: MRI can be used to image the liver, kidneys, pancreas, spleen, and bowel. It is used to diagnose a variety of conditions, including liver cirrhosis, kidney stones, pancreatic cancer, and inflammatory bowel disease. Pelvic MRI is used to evaluate the uterus, ovaries, and prostate.
-
Oncology: In addition to breast imaging, MRI is used to stage and monitor tumors in other parts of the body, including the brain, spine, liver, and prostate. Diffusion-weighted imaging (DWI) is a particularly useful technique for detecting and characterizing tumors based on their cellular density.
The versatility of MRI stems from its ability to generate images with excellent soft tissue contrast, without the use of ionizing radiation. Its applicability extends to a wide array of pathologies, making it a cornerstone of diagnostic medicine.
4. Advancements in MRI Technology
Continuous advancements in MRI technology have led to improved image quality, faster scan times, and expanded clinical applications. Some key advancements include:
-
Higher Field Strength Magnets: The transition from 1.5T to 3T and even 7T magnets has significantly improved signal-to-noise ratio (SNR) and spatial resolution. However, higher field strengths also present challenges such as increased susceptibility artifacts and specific absorption rate (SAR) limitations.
-
Parallel Imaging: Parallel imaging techniques, such as SENSE and GRAPPA, utilize multiple receiver coils to acquire data simultaneously, reducing scan times significantly. These techniques rely on coil sensitivity profiles to reconstruct undersampled data.
-
Compressed Sensing: Compressed sensing (CS) is a signal processing technique that allows for the acquisition of fewer data points than conventional methods, significantly reducing scan times. CS algorithms exploit the sparsity of images in certain transform domains to reconstruct images from undersampled data.
-
Advanced Pulse Sequences: Novel pulse sequences, such as diffusion tensor imaging (DTI), perfusion imaging, and magnetization transfer contrast (MTC), provide valuable information about tissue microstructure, function, and composition. These sequences are tailored to specific clinical applications and require sophisticated optimization.
-
Improved Gradient Systems: Stronger and faster gradient systems enable faster imaging speeds and improved spatial resolution. However, they also require careful management of gradient-induced eddy currents and peripheral nerve stimulation.
These technological advancements have collectively transformed MRI from a slow and technically demanding imaging modality into a fast, versatile, and clinically valuable tool.
5. Contrast Agents in MRI
Contrast agents are often used in MRI to enhance the visibility of certain tissues or structures. These agents are typically paramagnetic substances, such as gadolinium-based contrast agents (GBCAs), which shorten the T1 relaxation time of nearby water protons, leading to increased signal intensity on T1-weighted images. Liver-specific contrast agents can improve the detection and characterization of liver lesions, and blood pool contrast agents can improve visualization of blood vessels.
While GBCAs are generally considered safe, there have been concerns about the potential for gadolinium deposition in the brain and other tissues, particularly in patients with impaired renal function. This has led to increased scrutiny of GBCA usage and the development of alternative contrast agents, such as iron oxide nanoparticles. The risk of nephrogenic systemic fibrosis (NSF), a rare but serious complication associated with GBCA use in patients with severe renal impairment, has significantly decreased with the use of macrocyclic agents and careful screening of patients. Nevertheless, the potential for long-term deposition of gadolinium remains a subject of ongoing research.
The future of contrast-enhanced MRI may involve the development of more targeted and biocompatible contrast agents, as well as the exploration of alternative contrast mechanisms that do not rely on gadolinium.
6. Safety Considerations in MRI
MRI is generally considered a safe imaging modality, but several safety considerations must be addressed. The strong magnetic field can pose risks to patients with implanted metallic devices, such as pacemakers, defibrillators, and aneurysm clips. These devices may malfunction, heat up, or be dislodged by the magnetic field. Therefore, it is crucial to screen patients thoroughly for any contraindications before performing an MRI scan. Detailed information about the MR compatibility of implanted devices is essential, and appropriate precautions must be taken when scanning patients with conditional MR-compatible devices.
The RF pulses used in MRI can cause tissue heating, particularly at high field strengths. The specific absorption rate (SAR) is a measure of the RF power absorbed by the body. SAR limits are in place to prevent excessive tissue heating. Fast pulse sequences, such as echo-planar imaging (EPI), can generate significant heat deposition, so careful monitoring and adherence to SAR limits are essential.
Claustrophobia is another common concern for patients undergoing MRI. Open MRI scanners, which have a more open design, can be used to alleviate claustrophobia in some patients. Sedation may be necessary for patients who are severely claustrophobic.
Finally, acoustic noise generated during MRI can be a source of discomfort for patients. Earplugs or headphones should be provided to all patients to minimize noise exposure.
7. Cost-Effectiveness of MRI
MRI is a relatively expensive imaging modality compared to other techniques such as X-ray and ultrasound. The high cost of MRI is due to several factors, including the capital cost of the equipment, the operating costs (including power and maintenance), and the training and expertise required to operate the scanner and interpret the images. However, MRI can be cost-effective in certain clinical scenarios, particularly when it provides diagnostic information that cannot be obtained by other means. For example, MRI is often the most cost-effective imaging modality for diagnosing neurological conditions, such as multiple sclerosis and brain tumors.
Strategies to improve the cost-effectiveness of MRI include optimizing scan protocols, reducing scan times, and using MRI only when it is clinically indicated. Value-based imaging initiatives aim to ensure that MRI is used appropriately and that its benefits outweigh its costs. The use of AI to automate image analysis and interpretation can also improve efficiency and reduce the need for highly specialized radiologists, further contributing to cost savings.
8. The Role of Artificial Intelligence in MRI
The integration of artificial intelligence (AI) into MRI is rapidly transforming the field, with the potential to improve image quality, accelerate scan times, and enhance diagnostic accuracy. Deep learning algorithms, particularly convolutional neural networks (CNNs), have shown remarkable performance in various MRI applications.
-
Image Reconstruction: AI-based image reconstruction algorithms can improve image quality and reduce scan times by reconstructing images from undersampled data. These algorithms can learn to remove noise, correct for artifacts, and improve spatial resolution.
-
Image Segmentation: AI can automate the segmentation of anatomical structures and lesions in MRI images, reducing the time and effort required for manual segmentation. Accurate segmentation is crucial for quantitative analysis and treatment planning.
-
Image Classification and Diagnosis: AI algorithms can be trained to classify MRI images and diagnose various conditions, such as brain tumors, stroke, and Alzheimer’s disease. These algorithms can assist radiologists in making more accurate and timely diagnoses.
-
Workflow Optimization: AI can be used to optimize MRI workflow by automating tasks such as protocol selection, image quality assessment, and report generation. This can improve efficiency and reduce the workload on radiologists and technologists.
However, the use of AI in MRI also presents challenges. These include the need for large, high-quality datasets to train AI algorithms, the risk of bias in AI algorithms, and the need for regulatory approval of AI-based medical devices. Furthermore, the interpretability of AI algorithms is often limited, making it difficult to understand how they arrive at their conclusions. Despite these challenges, AI has the potential to revolutionize MRI and improve patient care.
9. Future Directions and Conclusion
MRI technology continues to evolve at a rapid pace, driven by advancements in hardware, pulse sequences, and software. Future research directions include:
-
Ultra-High Field MRI: The development of 11.7T and even higher field strength magnets promises to further improve SNR and spatial resolution, enabling new clinical applications.
-
Molecular MRI: Molecular MRI aims to develop contrast agents that can target specific molecular targets in vivo, providing information about disease processes at the molecular level.
-
Quantitative MRI: Quantitative MRI techniques provide objective measurements of tissue parameters, such as T1, T2, and diffusion coefficients, which can be used to diagnose and monitor disease.
-
Point-of-Care MRI: The development of portable and low-cost MRI scanners could expand access to MRI in resource-limited settings.
-
AI-Enhanced MRI: Continued advancements in AI will lead to more sophisticated image reconstruction, segmentation, and diagnostic algorithms, further improving the efficiency and accuracy of MRI.
In conclusion, MRI remains an indispensable tool in modern medicine, offering unparalleled soft tissue contrast and versatility across a wide range of clinical applications. Continuous advancements in MRI technology have led to improved image quality, faster scan times, and expanded clinical capabilities. The integration of AI into MRI is poised to further transform the field, with the potential to improve image analysis, interpretation, and overall workflow efficiency. While challenges remain, the future of MRI is bright, with the promise of even more powerful and clinically relevant applications in the years to come. We believe that the continued focus on reducing cost and increasing access to MRI will ensure its role as a central component of diagnostic imaging for decades to come.
References
[1] Westbrook C, Roth CK, Talbot J. MRI in practice. John Wiley & Sons; 2018.
[2] Haacke EM, Brown RW, Thompson MR, Venkatesan R. Magnetic resonance imaging: physical principles and sequence design. John Wiley & Sons; 1999.
[3] Bernstein MA, King KF, Zhou XJ. Handbook of MRI pulse sequences. Academic press; 2004.
[4] Kanal E, Barkovich AJ, Bell CA, Borgstede JP, Bradley WG Jr, Froelich JW, Gimbel JR, Goslin RH, Kanal MM, Lewin JS, Nyenhuis JA. ACR guidance document on MR safe practices: 2013. Journal of Magnetic Resonance Imaging. 2013 Mar;37(3):501-30.
[5] Gulani V, Caldemeyer KS, Kanal E, et al. Gadolinium deposition in the brain: summary of evidence and recommendations. The Lancet Neurology. 2017;16(11):896-907.
[6] Sodickson A, Baete SH, Jaeckel MC, et al. Broadening the applications of parallel MRI: fast imaging with semi-random Cartesian trajectories. Magnetic Resonance in Medicine. 2005;54(6):1469-79.
[7] Lustig M, Donoho DL, Pauly JM. Sparse MRI: the application of compressed sensing for rapid MR imaging. Magnetic Resonance in Medicine. 2007;58(6):1182-95.
[8] Litjens G, Kooi T, Bejnordi BE, et al. A survey on deep learning in medical image analysis. Medical Image Analysis. 2017;42:60-88.
[9] Esteva A, Kuprel B, Novoa RA, et al. Dermatologist-level classification of skin cancer with deep neural networks. Nature. 2017;542(7639):115-8.
[10] McKinney SM, Sieniek M, Godbole V, et al. International evaluation of an AI system for breast cancer screening. Nature. 2020;577(7788):86-94.
Molecular MRI targeting specific diseases? Sounds like sci-fi becoming reality! I wonder, will we soon have “smart” contrast agents that not only highlight the problem but deliver the cure too? Talk about a two-for-one special!
That’s an exciting thought! “Smart” contrast agents are definitely within the realm of possibility. Researchers are exploring targeted nanoparticles that can deliver drugs directly to diseased tissues while also providing enhanced imaging. It’s a challenging but promising area of research!
Editor: MedTechNews.Uk
Thank you to our Sponsor Esdebe
Molecular MRI, eh? Soon we’ll be getting personalized MRIs tailored to our specific DNA. Wonder if they’ll come with a family history report and ancestry breakdown too? Talk about knowing yourself inside and out!
That’s a fascinating perspective! The potential for personalized MRI based on individual genetic makeup is incredibly exciting. Imagine the possibilities for early disease detection and tailored treatment plans based on one’s unique genetic profile. Food for thought indeed!
Editor: MedTechNews.Uk
Thank you to our Sponsor Esdebe