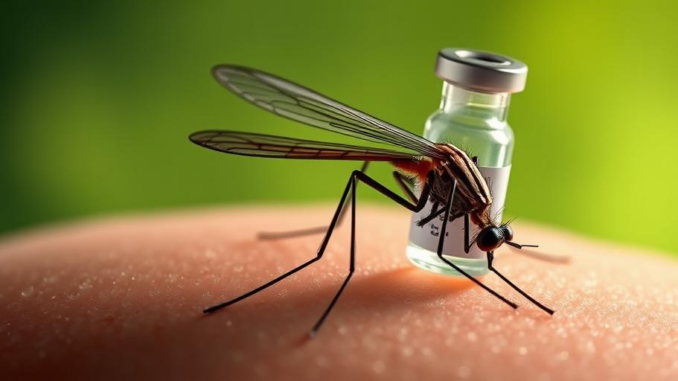
Abstract
Malaria, a mosquito-borne parasitic disease caused by Plasmodium parasites, continues to pose a significant global health burden, disproportionately affecting sub-Saharan Africa. This review provides a comprehensive overview of malaria, encompassing its epidemiology, causative agents, lifecycle, pathophysiology, prevention strategies, diagnostic methods, treatment options, the influence of climate change on transmission dynamics, and ongoing research endeavors aimed at vaccine development and eradication. Special emphasis is placed on the socioeconomic factors that exacerbate the disease burden, particularly among African children. The review critically assesses current control measures, highlights the challenges posed by drug resistance and vector insecticide resistance, and explores innovative approaches, including next-generation insecticides, gene editing technologies, and novel vaccine candidates. The urgent need for integrated strategies, coupled with sustained investment in research and development, is underscored to achieve the ambitious goal of malaria eradication.
Many thanks to our sponsor Esdebe who helped us prepare this research report.
1. Introduction
Malaria, a disease known to humanity for millennia, remains a formidable public health challenge in the 21st century. Transmitted by female Anopheles mosquitoes, the disease is caused by protozoan parasites of the genus Plasmodium. Although significant progress has been made in malaria control over the past two decades, the pace has plateaued, and in some regions, reversals have been observed. This stagnation is attributed to a confluence of factors, including the emergence and spread of drug-resistant parasites, insecticide-resistant mosquitoes, funding shortfalls, climate change, and weak healthcare systems. This review aims to provide a comprehensive and critical analysis of the key aspects of malaria, from its fundamental biology and epidemiology to the latest advances in prevention, diagnosis, treatment, and eradication strategies.
Many thanks to our sponsor Esdebe who helped us prepare this research report.
2. Epidemiology of Malaria
Malaria is endemic in over 80 countries, primarily in tropical and subtropical regions. The World Health Organization (WHO) estimates that in 2022, there were 249 million cases of malaria worldwide and 608,000 deaths. The African Region bears the brunt of the malaria burden, accounting for approximately 94% of global cases and 95% of deaths. Children under the age of five are particularly vulnerable, representing a significant proportion of malaria-related deaths. Factors influencing malaria transmission include vector abundance, human behavior, socioeconomic status, environmental conditions, and the effectiveness of control interventions.
2.1 Geographic Distribution and Risk Factors
The distribution of malaria is not uniform, even within endemic countries. Certain geographic areas, such as those with stagnant water bodies, high rainfall, and warm temperatures, are more conducive to mosquito breeding and parasite development. Human activities, such as deforestation, irrigation, and urbanization, can also alter the local ecology and increase malaria transmission risk. Socioeconomic factors, including poverty, inadequate housing, poor sanitation, and limited access to healthcare, play a crucial role in determining malaria vulnerability. Individuals living in poverty are often more exposed to mosquito bites due to substandard housing and lack of protective measures, such as insecticide-treated bed nets (ITNs). Furthermore, they may have limited access to diagnosis and treatment, leading to more severe disease outcomes.
2.2 Malaria in Sub-Saharan Africa: A Crisis of Disproportionate Impact
The disproportionate impact of malaria on sub-Saharan Africa is a stark reminder of the profound inequalities in global health. Several factors contribute to this disparity. Firstly, Anopheles gambiae, the primary malaria vector in Africa, is highly efficient at transmitting Plasmodium falciparum, the most virulent malaria parasite species. Secondly, the high prevalence of asymptomatic malaria infections in African populations, particularly in children, contributes to ongoing transmission. Thirdly, weak healthcare systems, characterized by limited resources, inadequate infrastructure, and a shortage of trained healthcare workers, hinder effective malaria control efforts. Finally, socioeconomic factors, such as poverty, lack of education, and gender inequality, exacerbate the disease burden.
Many thanks to our sponsor Esdebe who helped us prepare this research report.
3. Plasmodium Species Causing Malaria
Malaria in humans is caused by five Plasmodium species: P. falciparum, P. vivax, P. ovale, P. malariae, and P. knowlesi. Each species exhibits distinct biological characteristics, geographic distribution, and clinical manifestations.
- P. falciparum: The most virulent species, responsible for the majority of malaria-related deaths globally. Characterized by its ability to sequester in the microvasculature of vital organs, leading to severe complications such as cerebral malaria, severe anemia, and acute respiratory distress syndrome (ARDS).
- P. vivax: The most geographically widespread species, prevalent in Asia and Latin America. Can cause relapses due to the formation of dormant liver stages called hypnozoites.
- P. ovale: Similar to P. vivax in its ability to form hypnozoites, leading to relapses. Primarily found in West Africa.
- P. malariae: Causes a chronic, low-grade infection. Can persist in the human host for decades without causing symptoms.
- P. knowlesi: A zoonotic species, primarily found in Southeast Asia. Can cause severe malaria, particularly in older adults and those with underlying health conditions.
The relative importance of each Plasmodium species varies depending on the geographic region. In sub-Saharan Africa, P. falciparum is the predominant species, while P. vivax is more common in Asia and Latin America. P. knowlesi is an emerging threat in Southeast Asia, highlighting the importance of monitoring and surveillance to detect and respond to changes in the epidemiology of malaria.
Many thanks to our sponsor Esdebe who helped us prepare this research report.
4. Life Cycle of the Plasmodium Parasite
The Plasmodium parasite undergoes a complex life cycle involving both the mosquito vector and the human host. The life cycle can be broadly divided into two phases: the asexual phase in the human host and the sexual phase in the mosquito vector.
4.1 Asexual Phase in the Human Host
The asexual phase begins when an infected female Anopheles mosquito injects sporozoites into the human bloodstream during a blood meal. The sporozoites travel to the liver, where they invade hepatocytes and undergo asexual multiplication, forming schizonts. Each schizont ruptures, releasing thousands of merozoites into the bloodstream. Merozoites then invade red blood cells (erythrocytes), where they continue to multiply asexually, forming trophozoites and then schizonts. The schizonts rupture, releasing more merozoites to infect other red blood cells. This cycle of asexual multiplication in red blood cells is responsible for the clinical manifestations of malaria.
4.2 Sexual Phase in the Mosquito Vector
During the asexual phase in the human host, some merozoites differentiate into male and female gametocytes. When a female Anopheles mosquito takes a blood meal from an infected human, it ingests the gametocytes. In the mosquito’s midgut, the gametocytes undergo sexual reproduction, forming a zygote. The zygote develops into an ookinete, which penetrates the mosquito’s midgut wall and transforms into an oocyst. Within the oocyst, sporozoites develop. The oocyst eventually ruptures, releasing sporozoites that migrate to the mosquito’s salivary glands, completing the life cycle. When the infected mosquito takes another blood meal, it injects the sporozoites into a new human host, starting the cycle anew.
Many thanks to our sponsor Esdebe who helped us prepare this research report.
5. Pathophysiology of Malaria
The pathophysiology of malaria is complex and involves a combination of factors, including parasite burden, host immune response, and inflammatory mediators. The clinical manifestations of malaria range from mild, uncomplicated fever to severe, life-threatening complications.
5.1 Uncomplicated Malaria
Uncomplicated malaria is characterized by fever, chills, headache, muscle aches, and fatigue. These symptoms are often accompanied by nausea, vomiting, and diarrhea. The severity of symptoms varies depending on the Plasmodium species, the level of parasitemia, and the host’s immune status.
5.2 Severe Malaria
Severe malaria is a medical emergency characterized by one or more of the following complications:
- Cerebral Malaria: Characterized by coma, seizures, and neurological deficits. Results from the sequestration of infected red blood cells in the brain microvasculature, leading to inflammation and neuronal damage.
- Severe Anemia: Due to the destruction of infected and uninfected red blood cells by the parasite and the immune system.
- Acute Respiratory Distress Syndrome (ARDS): Due to increased pulmonary vascular permeability and fluid accumulation in the lungs.
- Renal Failure: Due to tubular necrosis and impaired kidney function.
- Metabolic Acidosis: Due to impaired tissue oxygenation and accumulation of lactic acid.
- Hypoglycemia: Due to increased glucose consumption by the parasite and impaired glucose production by the liver.
The pathogenesis of severe malaria is complex and involves the interplay of multiple factors. Sequestration of infected red blood cells in the microvasculature leads to impaired blood flow and tissue hypoxia. The release of inflammatory mediators, such as tumor necrosis factor-alpha (TNF-α) and interleukin-1 (IL-1), contributes to systemic inflammation and organ damage. The host immune response, while essential for parasite clearance, can also contribute to pathology. Excessive production of cytokines and antibodies can lead to immune-mediated injury.
Many thanks to our sponsor Esdebe who helped us prepare this research report.
6. Current Prevention Strategies
A multifaceted approach is essential for effective malaria prevention. The main strategies include vector control, chemoprophylaxis, and intermittent preventive treatment.
6.1 Vector Control
Vector control aims to reduce the density and infectivity of Anopheles mosquitoes. The primary methods include:
- Insecticide-Treated Bed Nets (ITNs): Long-lasting insecticidal nets (LLINs) are treated with pyrethroid insecticides and provide a physical barrier against mosquito bites. LLINs have been shown to be highly effective in reducing malaria transmission, particularly when used consistently and by a high proportion of the population.
- Indoor Residual Spraying (IRS): Involves spraying the inside walls of houses with insecticides. IRS can kill mosquitoes that land on the walls after feeding, reducing the likelihood of transmission. IRS is most effective when applied to a high proportion of houses in a given area.
- Larval Control: Targets mosquito larvae in their aquatic habitats. Methods include environmental management (e.g., draining stagnant water), biological control (e.g., using larvivorous fish), and the application of larvicides (e.g., Bacillus thuringiensis israelensis (Bti)).
The emergence and spread of insecticide resistance in Anopheles mosquitoes is a major challenge to vector control efforts. Alternative insecticides, such as pyrethroid-piperonyl butoxide (PBO) nets and next-generation insecticides, are being developed and deployed to combat insecticide resistance. Integrated vector management (IVM), which combines multiple control methods in a coordinated and sustainable manner, is essential for long-term malaria control.
6.2 Chemoprophylaxis
Chemoprophylaxis involves taking antimalarial drugs to prevent infection. It is recommended for travelers to malaria-endemic areas and for pregnant women in some settings. Commonly used chemoprophylactic drugs include:
- Atovaquone-proguanil (Malarone):
- Doxycycline:
- Mefloquine:
The choice of chemoprophylactic drug depends on the traveler’s destination, the local patterns of drug resistance, and the individual’s medical history. Adherence to chemoprophylaxis is crucial for its effectiveness.
6.3 Intermittent Preventive Treatment (IPT)
IPT involves administering antimalarial drugs at specific intervals to vulnerable populations, such as pregnant women (IPTp) and infants (IPTi), regardless of whether they are infected with malaria. IPTp with sulfadoxine-pyrimethamine (SP) is recommended by the WHO for pregnant women in areas with moderate to high malaria transmission. IPTi involves administering SP to infants at the time of routine vaccinations.
Many thanks to our sponsor Esdebe who helped us prepare this research report.
7. Diagnostic Methods
Accurate and timely diagnosis is essential for effective malaria management. The main diagnostic methods include:
- Microscopy: The gold standard for malaria diagnosis. Involves examining a Giemsa-stained blood smear under a microscope to identify Plasmodium parasites. Microscopy is relatively inexpensive and can detect all Plasmodium species, but it requires trained personnel and may be less sensitive at low parasite densities.
- Rapid Diagnostic Tests (RDTs): Immunochromatographic tests that detect Plasmodium antigens in a blood sample. RDTs are simple to use, require minimal training, and can provide results within minutes. However, RDTs may be less sensitive than microscopy, particularly for non-falciparum species. Quality control and proper storage are essential to ensure the accuracy of RDTs.
- Polymerase Chain Reaction (PCR): A highly sensitive and specific molecular diagnostic method that detects Plasmodium DNA in a blood sample. PCR is more expensive and requires specialized equipment and expertise, but it can detect low-density infections and identify Plasmodium species.
Point-of-care diagnostic tools are increasingly being used in malaria-endemic areas to improve access to diagnosis and treatment. These tools include mobile phone-based microscopy and RDTs with digital readers.
Many thanks to our sponsor Esdebe who helped us prepare this research report.
8. Treatment Options
The treatment of malaria depends on the Plasmodium species, the severity of the infection, and the patient’s age and health status. The mainstay of malaria treatment is artemisinin-based combination therapy (ACT).
8.1 Artemisinin-Based Combination Therapy (ACT)
ACTs combine a potent artemisinin derivative (e.g., artemether, artesunate) with a longer-acting partner drug (e.g., lumefantrine, amodiaquine, mefloquine, piperaquine). Artemisinins rapidly reduce parasite biomass, while the partner drug eliminates the remaining parasites. ACTs are highly effective in treating uncomplicated P. falciparum malaria, and their widespread use has contributed to a significant reduction in malaria morbidity and mortality.
8.2 Treatment of Severe Malaria
Severe malaria requires prompt and aggressive treatment, typically with intravenous artesunate. Quinine is an alternative if artesunate is not available. Supportive care, including fluid resuscitation, blood transfusion, and management of complications such as seizures and ARDS, is also essential.
8.3 Treatment of P. vivax and P. ovale
P. vivax and P. ovale infections require treatment with an ACT or chloroquine (in areas where chloroquine resistance is not prevalent), followed by primaquine to eliminate hypnozoites and prevent relapses. Tafenoquine is an alternative to primaquine, but it is contraindicated in individuals with glucose-6-phosphate dehydrogenase (G6PD) deficiency.
8.4 Drug Resistance
The emergence and spread of drug-resistant Plasmodium parasites is a major threat to malaria control. Resistance to chloroquine, sulfadoxine-pyrimethamine, and mefloquine has been reported in many malaria-endemic areas. Artemisinin resistance has emerged in Southeast Asia and is spreading to other regions. Strategies to combat drug resistance include the rational use of antimalarial drugs, the development of new antimalarial drugs, and the implementation of drug resistance surveillance programs.
Many thanks to our sponsor Esdebe who helped us prepare this research report.
9. Impact of Climate Change on Malaria Transmission
Climate change is expected to have a significant impact on malaria transmission, altering the geographic distribution of the disease and increasing the risk of epidemics. Warmer temperatures can accelerate parasite development in mosquitoes and increase mosquito breeding rates, leading to higher transmission intensity. Changes in rainfall patterns can create new breeding sites for mosquitoes and alter the seasonality of malaria transmission. Sea level rise can lead to the inundation of coastal areas, creating new breeding sites for mosquitoes and increasing the risk of malaria transmission in these regions. Climate change may also affect the distribution of Anopheles mosquitoes, expanding their range into previously malaria-free areas. Understanding the complex interactions between climate change and malaria transmission is crucial for developing effective adaptation strategies.
Many thanks to our sponsor Esdebe who helped us prepare this research report.
10. Ongoing Research Efforts for Vaccine Development and Eradication Strategies
Malaria eradication is a long-term goal that requires sustained investment in research and development. Ongoing research efforts are focused on developing new and improved tools for malaria prevention, diagnosis, and treatment, including vaccines, drugs, and vector control methods.
10.1 Vaccine Development
The development of an effective malaria vaccine is a major priority. Several malaria vaccine candidates are currently in clinical trials, targeting different stages of the parasite life cycle. The RTS,S/AS01 vaccine, also known as Mosquirix, is the first malaria vaccine to be recommended by the WHO for widespread use in children. The R21/Matrix-M vaccine has also demonstrated promising results in clinical trials. These vaccines provide partial protection against malaria and are expected to reduce the burden of disease in children. However, more effective vaccines are needed to achieve malaria eradication.
10.2 Eradication Strategies
Malaria eradication requires a comprehensive and integrated approach that combines multiple interventions, including vector control, chemoprophylaxis, diagnosis, and treatment. Key strategies include:
- Strengthening Healthcare Systems: Improving access to diagnosis, treatment, and prevention services, particularly in remote and underserved areas.
- Surveillance and Response: Establishing robust surveillance systems to detect and respond to malaria outbreaks promptly.
- Cross-Border Collaboration: Coordinating malaria control efforts across national borders to prevent the reintroduction of the disease.
- Community Engagement: Engaging communities in malaria control efforts to promote ownership and sustainability.
- Research and Development: Investing in research to develop new and improved tools for malaria prevention, diagnosis, and treatment.
Novel approaches, such as gene editing technologies and the use of artificial intelligence, are being explored to enhance malaria control efforts. Gene drive technology, which can spread desired traits through mosquito populations, holds promise for reducing mosquito populations and malaria transmission. Artificial intelligence can be used to analyze large datasets and identify patterns in malaria transmission, allowing for more targeted and effective interventions.
Many thanks to our sponsor Esdebe who helped us prepare this research report.
11. Conclusion
Malaria remains a significant global health challenge, disproportionately affecting sub-Saharan Africa. While progress has been made in malaria control over the past two decades, the pace has plateaued, and in some regions, reversals have been observed. The emergence and spread of drug-resistant parasites and insecticide-resistant mosquitoes, coupled with the effects of climate change and weak healthcare systems, pose major obstacles to malaria eradication. A comprehensive and integrated approach, combining vector control, chemoprophylaxis, diagnosis, treatment, and community engagement, is essential for achieving the ambitious goal of malaria eradication. Sustained investment in research and development, particularly in vaccine development and innovative vector control methods, is crucial for developing the tools needed to eliminate this devastating disease.
Many thanks to our sponsor Esdebe who helped us prepare this research report.
References
- World Health Organization. (2023). World Malaria Report 2023. Geneva: World Health Organization.
- Gething, P. W., et al. (2010). Climate change and the global malaria recession. Nature, 465(7296), 342-345.
- Greenwood, B. M. (2021). Malaria vaccines. Vaccine, 39(35), 4981-4987.
- Hemingway, J., et al. (2016). The urgent need to manage insecticide resistance in malaria vectors. Parasitology, 143(1), 3-18.
- Hoffman, S. L., et al. (2015). Malaria vaccines: challenges and opportunities. Cold Spring Harbor Perspectives in Medicine, 5(4), a017161.
- Okell, L. C., et al. (2012). The global distribution of asymptomatic Plasmodium falciparum and Plasmodium vivax infections. Malaria Journal, 11(1), 123.
- Slutsker, L., et al. (2021). Intermittent preventive treatment for malaria in infants (IPTi) with sulfadoxine-pyrimethamine. The Lancet, 397(10289), 2161-2162.
- WHO. (2021). A framework for malaria elimination. Geneva: World Health Organization.
- Ashley, E. A., et al. (2014). Spread of artemisinin resistance in Plasmodium falciparum malaria. New England Journal of Medicine, 371(5), 411-423.
- White, N. J. (2011). Artemisinin resistance. Cold Spring Harbor Perspectives in Medicine, 2(3), a007260.
- Staedke SG, Krishna S, May J, et al. Artesunate versus quinine for treatment of severe malaria in African children (AQUAMAT): an open-label, randomised trial. Lancet. 2004;363:525–531.
- Bhatt S, Weiss DJ, Cameron E, et al. The effect of malaria control on Plasmodium falciparum in Africa between 2000 and 2015. Nature. 2015;526:207–211.
- Bayoh MN, Mathias DK, Odiere MR, et al. Anopheles gambiae: historical population structure and genetic differentiation in East Africa. Malar J. 2011;10:223.
- Dondorp AM, Fairhurst RM, Slutsker L, et al. Artemisinin resistance in Plasmodium falciparum malaria. N Engl J Med. 2009;361:455–467.
- Kwiatkowski D, Manthalu E, Boele van Hensbroek M, et al. Adverse clinical outcome after prolonged fever is associated with persistent tumour necrosis factor-alpha production in children with falciparum malaria. J Infect Dis. 1990;161:1226–1231.
- Griffin JT, Hollingsworth TD, Reyburn H, et al. Gradual acquisition of effective immunity to malaria results in stabilizing transmission and is due to a non-specific process. PLoS Comput Biol. 2014;10:e1003643.
- Collins FH, Sakai RK, Vernick KD, et al. Genetic mapping of mosquito vectors. Science. 1986;234:607–610.
- Churcher TS, Lissenden N, Griffin JT, et al. The impact of persistent mosquito behavioural adaptations to ITNs on malaria control. Elife. 2016;5:e16760.
- Tarning J, Oguike MC, Maxwell CA, et al. Population pharmacokinetics of artesunate and lumefantrine and the implications for dosing in African children with uncomplicated malaria. PLoS Med. 2012;9:e1001296.
- O’Meara WP, Mangeni JN, Steketee R, Greenwood B. Changes in the burden of malaria in sub-Saharan Africa. Lancet Infect Dis. 2010;10:545–555.
Gene editing mosquitoes? Suddenly, the phrase “skeeter beater” sounds incredibly inadequate. I hope they’re also working on a mosquito GPS jammer so I can finally enjoy a summer evening in peace!
That GPS jammer idea is brilliant! While gene editing holds great potential, finding ways to deter mosquitoes from even finding us in the first place would be a game-changer for enjoying those summer evenings. It’s all about finding that balance between innovative solutions and practical relief!
Editor: MedTechNews.Uk
Thank you to our Sponsor Esdebe
The review highlights the urgent need for innovative vector control methods, including exploring gene drive technology. Further research into the ethical implications and potential ecological impacts of such technologies is crucial for responsible implementation.
Thank you for highlighting the importance of ethical considerations in the development of gene drive technology. Responsible innovation is key, and further research is vital to fully understand the potential impacts of gene editing technologies on ecosystems and human health. It’s a complex area with huge potential benefits if approached carefully.
Editor: MedTechNews.Uk
Thank you to our Sponsor Esdebe