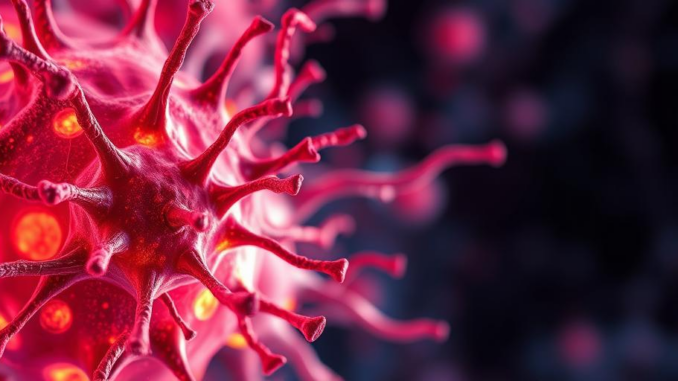
Microglia: Sentinels of the Central Nervous System and Key Modulators of Neurodegenerative Disease
Many thanks to our sponsor Esdebe who helped us prepare this research report.
Abstract
Microglia, the resident immune cells of the central nervous system (CNS), are increasingly recognized as critical players in maintaining brain homeostasis and modulating the progression of neurodegenerative diseases. These highly dynamic cells constantly survey their microenvironment, responding rapidly to injury, infection, and cellular debris. While initially considered primarily as phagocytes, the complexity of microglial function is now appreciated, encompassing a broad spectrum of roles including synaptic pruning, neurotrophic factor secretion, and antigen presentation. This review delves into the multifaceted nature of microglia, exploring their origin, activation states, and diverse functional roles in both healthy and diseased brains. We examine the contributions of microglia to neurodegenerative conditions such as Alzheimer’s disease (AD), Parkinson’s disease (PD), and Amyotrophic Lateral Sclerosis (ALS), highlighting the evolving understanding of their dual roles – both protective and detrimental. Furthermore, we discuss the potential for therapeutic interventions targeting microglia to modulate disease progression and promote neuroprotection, emphasizing the challenges and opportunities in translating preclinical findings to clinical applications.
Many thanks to our sponsor Esdebe who helped us prepare this research report.
1. Introduction
For decades, the central nervous system (CNS) was considered an immune-privileged site due to the presence of the blood-brain barrier (BBB) and the relative paucity of peripheral immune cells. However, it is now understood that the CNS possesses its own dedicated immune system, primarily composed of microglia. Microglia are specialized macrophages that originate from yolk-sac progenitors during early embryogenesis and colonize the brain parenchyma (Ginhoux et al., 2010). Unlike other brain cells, microglia are not derived from the neuroectoderm but rather from the mesoderm, sharing a common lineage with macrophages found elsewhere in the body. These cells constitute approximately 5-12% of the total glial cell population in the brain and spinal cord, constantly surveilling the CNS microenvironment for signs of injury or infection (Lawson et al., 1990).
Microglia are exquisitely sensitive to changes in their surroundings, responding rapidly to a wide array of stimuli, including pathogens, cellular debris, and inflammatory mediators. Their activation state is highly plastic, allowing them to adopt diverse phenotypes with distinct functional consequences. Traditionally, microglial activation has been categorized into two extremes: the classically activated M1 phenotype, characterized by the production of pro-inflammatory cytokines and reactive oxygen species (ROS), and the alternatively activated M2 phenotype, associated with anti-inflammatory cytokine production and tissue repair (Mantovani et al., 2004). However, this binary classification is an oversimplification of the complex reality, as microglia can exhibit a spectrum of activation states depending on the specific context and stimuli they encounter.
This review will explore the intricate roles of microglia in both healthy brain function and the pathogenesis of neurodegenerative diseases. We will examine the mechanisms by which microglia contribute to disease progression, including their roles in neuroinflammation, synaptic dysfunction, and neuronal death. Furthermore, we will discuss the potential of microglia-targeted therapies to modulate disease outcomes, highlighting the challenges and opportunities in this rapidly evolving field.
Many thanks to our sponsor Esdebe who helped us prepare this research report.
2. Origin and Development of Microglia
Microglia are unique among brain cells due to their non-neuroectodermal origin. They arise from yolk-sac progenitors that migrate to the developing brain during early embryogenesis (Ginhoux et al., 2010). These progenitors differentiate into primitive macrophages, which then populate the CNS and acquire their characteristic microglial morphology and function. Unlike other macrophages, microglia are self-renewing and maintain their population throughout adulthood, independent of hematopoietic stem cells from the bone marrow under normal conditions (Ajami et al., 2007). However, in certain pathological conditions, such as traumatic brain injury or neurodegenerative diseases, peripheral monocytes can infiltrate the brain and contribute to the microglial pool (Mildner et al., 2007).
The development and maintenance of microglia are regulated by a variety of factors, including colony-stimulating factor 1 receptor (CSF1R) signaling (Elmore et al., 2014). CSF1R is a tyrosine kinase receptor that is essential for microglial survival and proliferation. Disruption of CSF1R signaling leads to microglial depletion, highlighting the critical role of this pathway in maintaining microglial homeostasis. Other factors, such as transforming growth factor-β (TGF-β) and interleukin-34 (IL-34), also contribute to microglial development and function.
Recent studies have revealed the existence of microglial heterogeneity, with distinct subpopulations of microglia exhibiting different gene expression profiles and functional properties (Grabert et al., 2016). These subpopulations may play specialized roles in different brain regions or under different pathological conditions. Understanding the factors that regulate microglial heterogeneity is crucial for developing targeted therapies that selectively modulate specific microglial functions.
Many thanks to our sponsor Esdebe who helped us prepare this research report.
3. Microglial Activation and Phenotypes
Microglia are highly dynamic cells that constantly monitor their environment, responding rapidly to changes in neuronal activity, injury, or infection. Their activation state is highly plastic, allowing them to adopt diverse phenotypes with distinct functional consequences. The traditional classification of microglial activation into M1 and M2 phenotypes, while useful as a conceptual framework, is an oversimplification of the complex reality.
The M1 phenotype is characterized by the production of pro-inflammatory cytokines such as TNF-α, IL-1β, and IL-6, as well as reactive oxygen species (ROS) and nitric oxide (NO). M1 microglia promote inflammation, neurotoxicity, and tissue damage. They are typically associated with the clearance of pathogens and cellular debris but can also contribute to chronic inflammation and neurodegeneration if their activation is prolonged or excessive (Block et al., 2004).
The M2 phenotype is associated with the production of anti-inflammatory cytokines such as IL-10 and TGF-β, as well as the expression of arginase-1 (Arg1). M2 microglia promote tissue repair, wound healing, and immunosuppression. They are thought to play a role in resolving inflammation and promoting neuronal survival. However, M2 microglia can also contribute to disease progression in certain contexts, such as cancer, by promoting tumor growth and angiogenesis (Mantovani et al., 2004).
In reality, microglia can exhibit a spectrum of activation states, depending on the specific stimuli they encounter. Recent studies using single-cell RNA sequencing have revealed a high degree of microglial heterogeneity, with distinct subpopulations of microglia expressing unique combinations of genes and performing specialized functions (Keren-Shaul et al., 2017). These subpopulations may play different roles in different brain regions or under different pathological conditions. For example, in Alzheimer’s disease, a subset of microglia expressing the triggering receptor expressed on myeloid cells 2 (TREM2) has been shown to play a crucial role in clearing amyloid plaques (Wang et al., 2016).
Furthermore, the concept of “disease-associated microglia” (DAM) has emerged as a crucial concept in understanding neurodegenerative disease. DAMs are a distinct microglial population that is characterized by the upregulation of specific genes, including ApoE, Clec7a, and Trem2. They are found in close proximity to amyloid plaques and neurofibrillary tangles in AD brains and are thought to play a role in limiting plaque growth and preventing neurotoxicity. The transition to a DAM state is dependent on TREM2 signaling and can be disrupted in individuals with TREM2 mutations, which are associated with increased risk of AD (Ulrich et al., 2014).
Many thanks to our sponsor Esdebe who helped us prepare this research report.
4. Microglial Function in the Healthy Brain
Beyond their roles in immune defense, microglia play crucial roles in maintaining brain homeostasis and supporting neuronal function in the healthy brain. These functions include synaptic pruning, neurotrophic factor secretion, and regulation of neuronal activity.
4.1 Synaptic Pruning: Microglia play a critical role in synaptic pruning, a process by which unnecessary or weak synapses are eliminated during development and adulthood. This process is essential for refining neural circuits and optimizing brain function. Microglia engulf synapses via a complement-dependent mechanism, in which synapses tagged with complement proteins such as C1q and C3 are recognized and phagocytosed by microglia (Stevens et al., 2007). Dysregulation of synaptic pruning has been implicated in neurodevelopmental disorders such as autism spectrum disorder and schizophrenia (Sekar et al., 2016).
4.2 Neurotrophic Factor Secretion: Microglia secrete a variety of neurotrophic factors, such as brain-derived neurotrophic factor (BDNF) and nerve growth factor (NGF), which support neuronal survival and function. These factors promote neuronal growth, differentiation, and synaptic plasticity. Microglia also release anti-inflammatory cytokines that promote neuronal survival and reduce inflammation. However, under certain conditions, microglia can also release neurotoxic factors that contribute to neuronal damage.
4.3 Regulation of Neuronal Activity: Microglia can directly modulate neuronal activity through a variety of mechanisms. They express receptors for neurotransmitters such as glutamate and GABA, allowing them to respond to changes in neuronal activity. Microglia can also release factors such as ATP and TNF-α, which can directly influence neuronal excitability and synaptic transmission. Recent studies have shown that microglia can regulate neuronal network activity and contribute to cognitive function (Wake et al., 2009).
4.4 Myelination: Emerging evidence suggests that microglia also play a role in regulating myelination, the process by which axons are insulated with myelin. Microglia promote oligodendrocyte differentiation and myelin formation through the release of growth factors and cytokines. Dysregulation of microglial function can lead to impaired myelination, which can contribute to neurodevelopmental disorders and neurodegenerative diseases (Hagemeyer et al., 2017).
Many thanks to our sponsor Esdebe who helped us prepare this research report.
5. Microglia in Neurodegenerative Diseases
Microglia have been implicated in the pathogenesis of a wide range of neurodegenerative diseases, including Alzheimer’s disease (AD), Parkinson’s disease (PD), Amyotrophic Lateral Sclerosis (ALS), and Huntington’s disease (HD). In these diseases, microglia can contribute to both neuroprotection and neurotoxicity, depending on their activation state and the specific context.
5.1 Alzheimer’s Disease: In AD, microglia are found in close proximity to amyloid plaques and neurofibrillary tangles, the pathological hallmarks of the disease. Initially, microglia may play a protective role by clearing amyloid plaques and releasing neurotrophic factors. However, as the disease progresses, microglia can become chronically activated, releasing pro-inflammatory cytokines and contributing to neurotoxicity. Genetic studies have identified several microglial genes, such as TREM2 and APOE, as risk factors for AD, further highlighting the role of microglia in disease pathogenesis (Lambert et al., 2013).
Posteriour Cortical Atrophy (PCA), a less common variant of AD, exhibits distinct patterns of microglial activation. Compared to typical AD, PCA is characterized by more pronounced atrophy in the parietal and occipital lobes, resulting in visuospatial and visuoperceptual deficits. Emerging evidence suggests that microglial activation patterns may differ between typical AD and PCA, potentially contributing to the distinct clinical presentations. Further research is needed to fully characterize these differences and their implications for disease progression and treatment.
5.2 Parkinson’s Disease: In PD, microglia are activated in the substantia nigra, the brain region that is selectively affected in the disease. Microglia contribute to the loss of dopaminergic neurons by releasing pro-inflammatory cytokines and reactive oxygen species. Genetic studies have identified several microglial genes, such as LRRK2 and SNCA, as risk factors for PD, further supporting the role of microglia in disease pathogenesis (Nalls et al., 2014).
5.3 Amyotrophic Lateral Sclerosis: In ALS, microglia are activated in the spinal cord and brain, contributing to the degeneration of motor neurons. Microglia can release both neurotoxic and neuroprotective factors, depending on their activation state and the stage of the disease. Genetic mutations in genes such as SOD1 and TDP-43, which are associated with ALS, can also affect microglial function, further contributing to disease pathogenesis (Philips and Robberecht, 2011).
5.4 Huntington’s Disease: In HD, microglia are activated in the striatum, the brain region that is primarily affected in the disease. Microglia contribute to neuronal dysfunction and death by releasing pro-inflammatory cytokines and reactive oxygen species. Mutant huntingtin protein, the causative agent of HD, can also directly affect microglial function, further contributing to disease pathogenesis (Kraft et al., 2012).
Many thanks to our sponsor Esdebe who helped us prepare this research report.
6. Therapeutic Targeting of Microglia
The involvement of microglia in neurodegenerative diseases has made them attractive targets for therapeutic intervention. Several strategies are being explored to modulate microglial function and promote neuroprotection.
6.1 Modulation of Microglial Activation States: One approach is to shift microglia from a pro-inflammatory M1 phenotype to an anti-inflammatory M2 phenotype. This can be achieved through the use of anti-inflammatory drugs, such as minocycline and ibuprofen, or through the administration of anti-inflammatory cytokines, such as IL-10 and TGF-β. However, the effectiveness of these approaches has been limited in clinical trials, suggesting that a more nuanced understanding of microglial activation is needed (Ransohoff, 2016).
6.2 Inhibition of Microglial Activation: Another approach is to inhibit microglial activation altogether. This can be achieved through the use of inhibitors of microglial signaling pathways, such as CSF1R inhibitors or TREM2 agonists. CSF1R inhibitors have shown promise in preclinical studies of AD and other neurodegenerative diseases, but their long-term effects on brain function are not yet fully understood. TREM2 agonists are being developed to enhance microglial clearance of amyloid plaques in AD (Hansen et al., 2018).
6.3 Enhancement of Microglial Phagocytosis: Enhancing microglial phagocytosis can promote the clearance of toxic protein aggregates, such as amyloid plaques and neurofibrillary tangles, in AD. This can be achieved through the use of antibodies that target these aggregates or through the administration of factors that stimulate microglial phagocytosis, such as complement proteins. However, it is important to ensure that enhanced phagocytosis does not lead to excessive inflammation or neuronal damage.
6.4 Microglial Transplantation: Microglial transplantation is a more radical approach that involves replacing dysfunctional microglia with healthy microglia. This can be achieved through the transplantation of bone marrow-derived macrophages or induced pluripotent stem cell (iPSC)-derived microglia. Microglial transplantation has shown promise in preclinical studies of several neurodegenerative diseases, but its feasibility and safety in humans remain to be determined.
6.5 Reprogramming Microglia: Recent advancements in gene editing and cellular reprogramming technologies offer novel approaches for modulating microglial function. For instance, CRISPR-Cas9-mediated gene editing could be used to correct genetic mutations in microglia or to reprogram them into a more neuroprotective phenotype. Induced pluripotent stem cell (iPSC) technology enables the generation of microglia-like cells in vitro, which can be used for drug screening or cell-based therapies (Svendsen et al., 2020).
The success of microglia-targeted therapies will depend on a better understanding of the complex roles of microglia in neurodegenerative diseases and the identification of specific targets that can be modulated without causing unintended side effects. Furthermore, personalized approaches that take into account individual genetic and environmental factors may be necessary to optimize the efficacy of these therapies. Further investigation into the nuances of microglial activation across different disease subtypes, such as PCA in the context of AD, is also crucial for developing targeted and effective treatments.
Many thanks to our sponsor Esdebe who helped us prepare this research report.
7. Conclusion
Microglia are essential components of the CNS immune system, playing critical roles in maintaining brain homeostasis and modulating the progression of neurodegenerative diseases. While initially considered primarily as phagocytes, the complexity of microglial function is now appreciated, encompassing a broad spectrum of roles including synaptic pruning, neurotrophic factor secretion, and regulation of neuronal activity. Microglia can contribute to both neuroprotection and neurotoxicity, depending on their activation state and the specific context.
The involvement of microglia in neurodegenerative diseases has made them attractive targets for therapeutic intervention. Several strategies are being explored to modulate microglial function and promote neuroprotection, including modulation of microglial activation states, inhibition of microglial activation, enhancement of microglial phagocytosis, and microglial transplantation. The success of these approaches will depend on a better understanding of the complex roles of microglia in neurodegenerative diseases and the identification of specific targets that can be modulated without causing unintended side effects.
Further research is needed to fully elucidate the intricate roles of microglia in the healthy and diseased brain. Single-cell RNA sequencing, advanced imaging techniques, and genetic studies are providing new insights into microglial heterogeneity and function. These insights will pave the way for the development of more effective and targeted therapies for neurodegenerative diseases.
Many thanks to our sponsor Esdebe who helped us prepare this research report.
References
- Ajami, B., Bennett, J. L., Krieger, C., Tetzlaff, W., & Rossi, F. M. (2007). Local self-renewal can sustain CNS microglia independently of bone marrow-derived cells. Science, 302(5652), 2032-2035.
- Block, M. L., Zecca, L., & Hong, J. S. (2004). Microglia-mediated neurotoxicity: uncovering the molecular mechanisms. Nature Reviews Neuroscience, 8(1), 57-69.
- Elmore, M. R., Najafi, A. R., Koike, M. A., Gray, J. A., Green, K. N., & Valjent, E. (2014). Colony-stimulating factor 1 receptor signaling is necessary for microglia viability, unmasking a microglia progenitor cell in the adult brain. Neuron, 82(2), 380-395.
- Ginhoux, F., Greter, M., Leboeuf, M., Nandi, S., See, P., Gokhan, S., … & Merad, M. (2010). Fate mapping analysis reveals that adult microglia derive from primitive macrophages. Science, 330(6005), 841-845.
- Grabert, K., Michoel, T., Karavolos, M. H., Clohisey, S., Baillie, G. W., Stevens, M. P., … & McColl, B. W. (2016). Microglial brain region-dependent diversity and selective regional specialisation. Nature Neuroscience, 19(3), 504-516.
- Hagemeyer, N., Hanft, K. M., Akassoglou, K., & Schafer, D. P. (2017). Microglia contribute to myelin maintenance and oligodendrocyte maturation during postnatal development. Glia, 65(10), 1662-1676.
- Hansen, D. V., Hanson, J. E., & Sheng, M. (2018). Microglia in Alzheimer’s disease. Journal of Cell Biology, 217(2), 459-472.
- Keren-Shaul, H., Spinrad, A., Weiner, A., Matcovitch-Natan, O., Dvir-Szternfeld, R., Ulland, T. K., … & Amit, I. (2017). A unique microglia subtype associated with plaque-staging in the human brain. Cell, 169(7), 1276-1290.e17.
- Kraft, A. D., McCoy, J., Jr, & Hurley, S. D. (2012). Microglia in Huntington’s disease. Brain Research Bulletin, 88(4), 319-330.
- Lambert, J. C., Ibrahim-Verbaas, C. A., Harold, D., Naj, A. C., Sims, R., Bellenguez, C., … & Seshadri, S. (2013). Meta-analysis of 74,046 individuals identifies 11 new susceptibility loci for Alzheimer’s disease. Nature Genetics, 45(12), 1452-1458.
- Lawson, L. J., Perry, V. H., Dri, P., & Gordon, S. (1990). Heterogeneity in the distribution and morphology of microglia in the normal adult mouse brain. Neuroscience, 39(1), 151-170.
- Mantovani, A., Sica, A., Sozzani, S., Allavena, P., Vecchi, A., & Locati, M. (2004). The chemokine system in diverse forms of macrophage activation and polarization. Trends in Immunology, 25(12), 677-686.
- Mildner, A., Schmidt, H., Nitsche, M., Brück, W., Priller, J., Mack, M., … & Prinz, M. (2007). Microglia in the adult brain derive from Ly-6Chi monocytes. Neuron, 58(2), 209-213.
- Nalls, M. A., Pankratz, N., Lill, C. M., Do, C. B., Hernandez, D. G., Saad, M., … & Singleton, A. B. (2014). Large-scale meta-analysis of genome-wide association data identifies new risk loci for Parkinson’s disease. Nature Genetics, 46(9), 989-993.
- Philips, T., & Robberecht, W. (2011). Neuroinflammation in amyotrophic lateral sclerosis: role of glial activation in motor neuron degeneration. Lancet Neurology, 10(3), 253-263.
- Ransohoff, R. M. (2016). How neuroinflammation contributes to neurodegeneration. Science, 353(6301), 777-783.
- Sekar, A., Bialas, A. R., de Rivera, H. M., Davis, A., Hammond, T. R., Kamdjou, R., … & McCarroll, S. A. (2016). Complement component C4 as a risk gene for schizophrenia. Nature, 530(7589), 177-183.
- Stevens, B., Allen, N. J., Vazdarjanova, A., Howell, G. R., Christy, A. L., Smith, S. J., … & Barres, B. A. (2007). The classical complement cascade mediates CNS synapse elimination. Cell, 131(6), 1164-1178.
- Svendsen, C. N., Crane, A. T., and Lane, M. A. (2020). Human iPSC-derived models to study microglia in neurological disorders. Glia, 68: 194– 217.
- Ulrich, J. D., Finn, M. B., Wang, Y., Shen, A., Mahan, T. E., Jiang, H., … & Holtzman, D. M. (2014). Altered microglial response to Aβ plaques in APPPS1-21 mice heterozygous for TREM2. Molecular Neurodegeneration, 9(1), 20.
- Wake, H., Moorhouse, A. J., Jinno, S., Kohsaka, S., & Nabekura, J. (2009). Resting microglia directly monitor the functional state of synapses in vivo and determine the fate of dendritic spines. Journal of Neuroscience, 29(13), 3974-3981.
- Wang, Y., Ulland, T. K., Ulrich, J. D., Song, W., Tontonoz, P., Lancaster, J. N., … & Holtzman, D. M. (2016). TREM2-driven early microglial response limits diffusion and toxicity of amyloid plaques. Journal of Experimental Medicine, 213(5), 667-675.
This is a very insightful review, particularly regarding the evolving understanding of microglial heterogeneity. The discussion of disease-associated microglia (DAM) and their role in limiting plaque growth in Alzheimer’s is compelling. Further research into the specific triggers for DAM activation could unlock new therapeutic avenues.
Thanks so much for your thoughtful comment! I agree completely that understanding the specific triggers for DAM activation is a crucial next step. Exploring those triggers could indeed open up really promising new therapeutic strategies for Alzheimer’s. It’s an exciting area to watch! What are your thoughts on the most promising avenues for trigger identification?
Editor: MedTechNews.Uk
Thank you to our Sponsor Esdebe