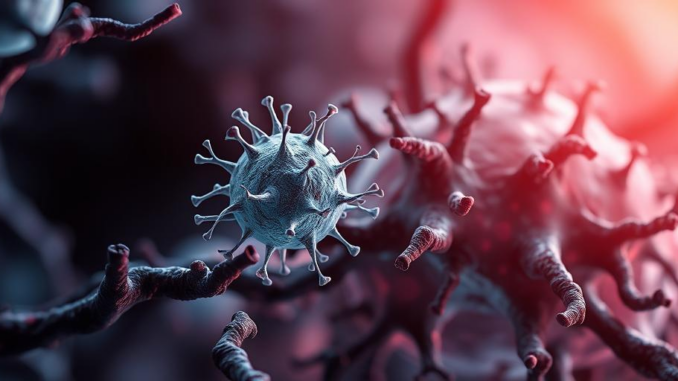
Abstract
Microglia, the resident immune cells of the central nervous system (CNS), are critical regulators of brain homeostasis. Once considered a homogenous population of macrophages, it is now recognized that microglia exhibit remarkable heterogeneity and plasticity, adapting their morphology, phenotype, and function in response to diverse stimuli and pathological conditions. This review delves into the multifaceted nature of microglia, exploring the factors that contribute to their heterogeneity, including developmental origin, regional specialization, and disease-specific cues. We examine the complex roles of microglia in neurodegenerative diseases, focusing on Alzheimer’s disease (AD), Parkinson’s disease (PD), and Amyotrophic Lateral Sclerosis (ALS), where microglia can exhibit both neuroprotective and neurotoxic functions. Furthermore, we discuss the potential of targeting microglial activity as a therapeutic strategy for neurodegenerative disorders, considering the challenges and opportunities associated with modulating their diverse and context-dependent functions. We will also review current methods of targeting microglia and consider their relative efficacies. Finally, we consider the therapeutic potential of harnessing microglial plasticity to restore homeostasis and prevent neurodegeneration.
Many thanks to our sponsor Esdebe who helped us prepare this research report.
1. Introduction
Neurodegenerative diseases, characterized by progressive neuronal loss and cognitive decline, pose a significant global health burden. While the precise etiology of these disorders remains elusive, chronic neuroinflammation is increasingly recognized as a key contributing factor. Microglia, the resident immune cells of the brain, play a central role in mediating neuroinflammation. These cells constantly survey their microenvironment, responding to injury, infection, and cellular debris. Initially, microglia may adopt a neuroprotective phenotype, clearing pathogens and promoting tissue repair. However, in the context of chronic neurodegeneration, microglia can become chronically activated, releasing pro-inflammatory mediators that exacerbate neuronal damage and contribute to disease progression. The complexity of microglial function is further compounded by their inherent heterogeneity and plasticity. Understanding these intricacies is essential for developing effective therapeutic strategies that target microglia to prevent or reverse neurodegeneration.
Microglia are the primary innate immune cells in the central nervous system (CNS), acting as sentinels constantly monitoring their environment. These cells originate from myeloid progenitors in the yolk sac during early embryonic development and colonize the brain before the formation of the blood-brain barrier. This unique origin distinguishes them from other tissue macrophages and contributes to their specific functional characteristics. Once resident in the CNS, microglia play crucial roles in brain development, homeostasis, and immune defense. They are involved in synaptic pruning, neuronal circuit refinement, and the clearance of cellular debris and pathogens.
Historically, microglia were viewed as a relatively homogenous population, responding uniformly to stimuli. However, recent advances in single-cell genomics, proteomics, and imaging techniques have revealed a remarkable degree of heterogeneity and plasticity within the microglial compartment. Microglia exhibit diverse morphologies, marker expression profiles, and functional capabilities, varying across brain regions, developmental stages, and pathological conditions. This diversity reflects their ability to adapt to different microenvironments and perform specialized tasks. The precise factors that govern microglial heterogeneity are still being investigated, but they likely include developmental programming, regional cues, epigenetic modifications, and exposure to various stimuli, such as cytokines, chemokines, and misfolded proteins.
In neurodegenerative diseases, microglia are activated in response to neuronal damage and the accumulation of pathological protein aggregates. Depending on the context, activated microglia can exert either neuroprotective or neurotoxic effects. In the early stages of disease, microglia may promote neuronal survival by clearing toxic proteins, releasing neurotrophic factors, and resolving inflammation. However, as the disease progresses, chronic activation of microglia can lead to the sustained release of pro-inflammatory cytokines, reactive oxygen species, and other neurotoxic mediators, contributing to neuronal dysfunction and death. The switch from neuroprotective to neurotoxic phenotypes is thought to be influenced by factors such as the type and concentration of activating stimuli, the duration of exposure, and the intrinsic properties of the microglia themselves.
Targeting microglia to modulate their activity represents a promising therapeutic strategy for neurodegenerative diseases. However, the complexity of microglial function poses a significant challenge. Simply suppressing microglial activation may not be beneficial, as it could impair their protective functions and compromise immune surveillance. A more nuanced approach is needed to selectively modulate microglial activity, promoting their neuroprotective functions while suppressing their neurotoxic effects. This could involve targeting specific signaling pathways, manipulating microglial polarization, or enhancing their ability to clear pathological protein aggregates. Furthermore, it is crucial to consider the heterogeneity of microglia when designing therapeutic interventions, as different microglial subpopulations may respond differently to the same treatment. Some approaches include selective removal of activated or detrimental subtypes of microglia. This could involve targeting surface markers specific to activated microglia, and using antibody-mediated phagocytosis to induce their removal.
Many thanks to our sponsor Esdebe who helped us prepare this research report.
2. Factors Influencing Microglial Heterogeneity
Microglial heterogeneity is a complex phenomenon shaped by multiple factors, including developmental origin, regional specialization, epigenetic regulation, and environmental cues. Understanding these factors is crucial for developing targeted therapeutic interventions that modulate microglial activity in a context-dependent manner.
2.1 Developmental Origin
Microglia originate from yolk sac-derived myeloid progenitors that colonize the brain early in development. Unlike other tissue macrophages, microglia do not arise from hematopoietic stem cells in the bone marrow under homeostatic conditions. This unique developmental origin contributes to their distinct transcriptional profile and functional characteristics. However, under certain pathological conditions, such as brain injury or inflammation, bone marrow-derived monocytes can infiltrate the brain and differentiate into microglia-like cells. These infiltrating cells may exhibit different phenotypes and functions compared to resident microglia, adding another layer of complexity to the microglial landscape.
2.2 Regional Specialization
Microglia exhibit regional specialization, with distinct morphologies, marker expression profiles, and functional capabilities in different brain regions. For example, microglia in the hippocampus, a region involved in learning and memory, have been shown to express higher levels of certain receptors and exhibit different responses to inflammatory stimuli compared to microglia in the cortex. These regional differences may reflect the unique functional demands of each brain region and the specific interactions between microglia and other cell types, such as neurons and astrocytes. Microglia in the white matter exhibit distinct features compared to those in the gray matter, reflecting their roles in supporting myelin integrity and axonal function. This heterogeneity is seen at both the genetic and protein expression levels, as well as functional differences such as phagocytic activity and cytokine release.
2.3 Epigenetic Regulation
Epigenetic mechanisms, such as DNA methylation and histone modification, play a crucial role in regulating microglial gene expression and function. These epigenetic modifications can be influenced by environmental factors, such as diet, stress, and exposure to toxins, leading to long-lasting changes in microglial phenotype. Epigenetic modifications can contribute to the development of distinct microglial subtypes and influence their response to inflammatory stimuli. Furthermore, epigenetic changes can be transmitted across generations, potentially contributing to the increased risk of neurodegenerative diseases in individuals with a family history of these disorders.
2.4 Environmental Cues
Microglia are highly sensitive to their microenvironment, responding to a wide range of cues, including cytokines, chemokines, neurotransmitters, and damage-associated molecular patterns (DAMPs). These cues can activate different signaling pathways within microglia, leading to changes in gene expression and function. Chronic exposure to inflammatory stimuli can drive microglia towards a pro-inflammatory phenotype, contributing to neuronal damage. Conversely, exposure to anti-inflammatory signals can promote a neuroprotective phenotype. The balance between these opposing signals determines the overall effect of microglia on neuronal health.
Many thanks to our sponsor Esdebe who helped us prepare this research report.
3. Microglial Roles in Neurodegenerative Diseases
Microglia play complex and often contradictory roles in neurodegenerative diseases. They can be both beneficial and detrimental, depending on the stage of the disease, the specific context, and the characteristics of the microglia themselves.
3.1 Alzheimer’s Disease (AD)
In AD, microglia are activated in response to the accumulation of amyloid-beta (Aβ) plaques and neurofibrillary tangles, the pathological hallmarks of the disease. Initially, microglia may promote Aβ clearance and prevent plaque formation. However, as the disease progresses, chronic activation of microglia can lead to the release of pro-inflammatory cytokines and reactive oxygen species, contributing to neuronal damage and cognitive decline. Genome-wide association studies (GWAS) have identified several AD risk genes that are specifically expressed in microglia, highlighting the importance of these cells in disease pathogenesis. These genes are involved in processes such as Aβ clearance, inflammation, and lipid metabolism. TREM2 (Triggering Receptor Expressed on Myeloid cells 2) is one such risk gene which regulates microglial activation, phagocytosis, and survival. Loss-of-function mutations in TREM2 are associated with increased risk of AD, suggesting that impaired microglial function contributes to disease development. Research has found that TREM2 is a key regulator of microglial response to amyloid plaques. In its absence, microglia are less efficient at clearing amyloid plaques. Recently, researchers have focused on targeting TREM2 and other microglial associated genes to restore microglial function and reduce the risk of AD.
3.2 Parkinson’s Disease (PD)
In PD, microglia are activated in the substantia nigra, the brain region affected by the disease, in response to the accumulation of alpha-synuclein aggregates and the loss of dopaminergic neurons. Activated microglia release pro-inflammatory cytokines and contribute to the degeneration of dopaminergic neurons. Similar to AD, GWAS studies have identified several PD risk genes that are expressed in microglia, including LRRK2 (Leucine-Rich Repeat Kinase 2) and SNCA (alpha-Synuclein). These genes are involved in processes such as inflammation, autophagy, and protein aggregation. Microglial activation has been shown to directly contribute to the degeneration of dopaminergic neurons in vitro and in vivo. Blocking microglial activation or depleting microglia can protect dopaminergic neurons and improve motor function in animal models of PD.
3.3 Amyotrophic Lateral Sclerosis (ALS)
In ALS, microglia are activated in the spinal cord and brain, contributing to the degeneration of motor neurons. The role of microglia in ALS is complex and may vary depending on the stage of the disease. In the early stages, microglia may promote motor neuron survival by releasing neurotrophic factors. However, as the disease progresses, microglia become chronically activated and release pro-inflammatory cytokines and reactive oxygen species, contributing to motor neuron death. Mutations in the SOD1 (Superoxide Dismutase 1) gene are a common cause of familial ALS. Mutant SOD1 protein can activate microglia and induce the release of neurotoxic factors. Studies have shown that suppressing microglial activation or depleting microglia can delay disease progression and extend survival in SOD1 mutant mice. Recent studies have shown that a specific subpopulation of microglia, characterized by the expression of CD14, may be particularly detrimental in ALS. Targeting this subpopulation of microglia could represent a promising therapeutic strategy.
3.4 Other Neurodegenerative Diseases
Microglial involvement has been observed in other neurodegenerative diseases such as Huntington’s disease (HD), Frontotemporal Dementia (FTD) and Multiple Sclerosis (MS). In HD, microglial activation is associated with the mutant huntingtin protein. In FTD, microglia surround tau protein aggregates and are associated with the disease progression. In MS, microglia contribute to inflammation and demyelination. The involvement of microglia in these diseases underscores the importance of further research into their role in neurodegenerative disorders.
Many thanks to our sponsor Esdebe who helped us prepare this research report.
4. Therapeutic Strategies for Modulating Microglial Activity
Targeting microglial activity represents a promising therapeutic strategy for neurodegenerative diseases. However, the complexity of microglial function requires a nuanced approach that selectively modulates their activity, promoting their neuroprotective functions while suppressing their neurotoxic effects.
4.1 Targeting Microglial Activation
One approach is to target the signaling pathways that regulate microglial activation. Several kinases, transcription factors, and inflammatory mediators have been identified as potential therapeutic targets. For example, inhibiting the NF-κB signaling pathway, a key regulator of inflammation, has been shown to reduce microglial activation and protect neurons in animal models of neurodegenerative diseases. Inhibiting inflammasome activation has also shown neuroprotective effects in animal models of neurodegenerative diseases.
4.2 Manipulating Microglial Polarization
Another approach is to manipulate microglial polarization, shifting them from a pro-inflammatory (M1) phenotype to an anti-inflammatory (M2) phenotype. This can be achieved by administering anti-inflammatory cytokines, such as IL-4 or IL-10, or by using pharmacological agents that promote M2 polarization. For example, agonists of peroxisome proliferator-activated receptor gamma (PPARγ) have been shown to promote M2 polarization and protect neurons in animal models of AD and PD. However, the M1/M2 dichotomy is an oversimplification of microglial phenotypes, and more nuanced strategies are needed to selectively modulate microglial activity.
4.3 Enhancing Microglial Clearance of Pathological Proteins
Enhancing microglial clearance of pathological proteins, such as Aβ and alpha-synuclein, represents another therapeutic strategy. This can be achieved by stimulating microglial phagocytosis or by promoting the degradation of pathological proteins within microglia. For example, antibodies that bind to Aβ can enhance microglial phagocytosis and promote Aβ clearance. Activating autophagy, a cellular process that degrades damaged proteins and organelles, has also been shown to reduce pathological protein levels and improve neuronal survival. Antibody based approaches are used frequently for this purpose.
4.4 Methods of Targeting Microglia in the Eye
Although the initial context of this paper was targeting microglia in the eye, its scope has become much broader. Nevertheless it is useful to consider some methods which may have utility in modulating microglial activity in the eye, and to compare their relative efficacies.
- Topical Eye Drops: This is the least invasive method, delivering drugs directly to the eye surface. However, penetration to the retina and deeper ocular tissues is limited due to the blood-retinal barrier. It’s most suitable for treating conditions affecting the anterior segment, like conjunctivitis or corneal inflammation. For retinal microglia, efficacy is generally low unless the drug is designed for improved penetration.
- Subconjunctival Injections: Injections under the conjunctiva allow drugs to bypass the corneal epithelium and reach the sclera and choroid. This method provides better drug delivery to the posterior segment compared to topical drops, but still faces limitations in reaching the retina due to diffusion barriers.
- Intravitreal Injections: Direct injections into the vitreous humor offer the most effective way to deliver drugs to the retina and target retinal microglia. This method allows for high drug concentrations to be achieved in the immediate vicinity of the microglia. However, it’s an invasive procedure with potential risks, such as retinal detachment, infection, and increased intraocular pressure. Drugs delivered by IVT have shorter duration and can have low bioavailability.
- Systemic Administration: Oral or intravenous drug delivery allows the drug to reach the retina via the bloodstream. This method is non-invasive but requires the drug to cross the blood-retinal barrier, which can significantly limit its efficacy. Systemic administration may also lead to unwanted side effects in other parts of the body.
- Sustained-Release Implants: These devices are surgically implanted into the eye (e.g., intravitreal implant) and slowly release the drug over a period of weeks or months. This method provides sustained drug delivery to the retina, reducing the need for frequent injections. However, implantation requires surgery, and there’s a risk of implant-related complications.
-
Gene Therapy: Viral vectors (e.g., adeno-associated virus) can be used to deliver genes encoding therapeutic proteins directly to retinal cells, including microglia. This method offers the potential for long-term gene expression and sustained therapeutic effects. However, gene therapy is still an experimental approach with potential risks, such as immune responses and off-target effects.
-
Nanoparticle-Based Drug Delivery: Nanoparticles can be engineered to encapsulate drugs and target specific cells or tissues in the eye, including microglia. These particles can be administered via different routes (e.g., intravitreal injection, systemic administration) and can be designed to cross the blood-retinal barrier more efficiently. However, further research is needed to optimize nanoparticle design and assess their safety and efficacy in vivo.
The relative efficacies of these methods depend on the specific drug, the target tissue, and the desired therapeutic effect. Intravitreal injections and sustained-release implants generally provide the most effective drug delivery to the retina, while topical eye drops and systemic administration are less effective for targeting retinal microglia. Nanoparticle-based drug delivery and gene therapy hold promise for improving drug delivery and achieving long-term therapeutic effects, but further research is needed to validate their potential.
4.5 Considerations for Clinical Translation
While preclinical studies have shown promising results in modulating microglial activity, several challenges remain before these strategies can be translated into clinical therapies. First, it is crucial to identify specific microglial subpopulations that contribute to disease pathogenesis and develop targeted therapies that selectively modulate their activity. Second, it is important to consider the potential off-target effects of microglial-targeted therapies, as microglia play important roles in brain homeostasis and immune defense. Third, it is necessary to develop biomarkers that can track microglial activity and monitor the response to therapy. Fourth, it is important to consider the timing of intervention, as microglial activation may have different effects at different stages of the disease. Finally, clinical trials should be designed to account for the heterogeneity of neurodegenerative diseases and the variability in microglial responses among individuals.
Many thanks to our sponsor Esdebe who helped us prepare this research report.
5. Harnessing Microglial Plasticity for Neuroprotection
Rather than simply suppressing microglial activation, an alternative approach is to harness their inherent plasticity and promote a neuroprotective phenotype. This could involve stimulating the expression of neurotrophic factors, enhancing the clearance of pathological proteins, or resolving inflammation. One promising strategy is to use immunomodulatory agents, such as antibodies or cytokines, to reprogram microglia towards a neuroprotective state. For example, antibodies that block the interaction between microglia and pro-inflammatory cytokines can reduce inflammation and promote neuronal survival. Another strategy is to use epigenetic modifiers to alter microglial gene expression and promote a neuroprotective phenotype. However, further research is needed to identify specific epigenetic targets and develop safe and effective epigenetic therapies.
Many thanks to our sponsor Esdebe who helped us prepare this research report.
6. Future Directions
The field of microglial research is rapidly evolving, with new discoveries constantly reshaping our understanding of their role in neurodegenerative diseases. Future research should focus on: (1) Characterizing the diversity of microglial phenotypes and their functional roles in different brain regions and disease stages; (2) Identifying the molecular mechanisms that regulate microglial plasticity and their response to environmental cues; (3) Developing novel therapeutic strategies that selectively modulate microglial activity, promoting their neuroprotective functions while suppressing their neurotoxic effects; (4) Developing biomarkers that can track microglial activity and predict the response to therapy; (5) Conducting clinical trials to evaluate the efficacy and safety of microglial-targeted therapies in patients with neurodegenerative diseases.
The development of high-throughput screening platforms and advanced imaging techniques will accelerate the discovery of new microglial targets and therapeutic agents. Furthermore, the integration of multi-omics data, including genomics, proteomics, and metabolomics, will provide a more comprehensive understanding of microglial biology and their interactions with other brain cells. Finally, collaboration between basic scientists, clinicians, and industry partners is essential for translating research findings into effective therapies for neurodegenerative diseases.
Many thanks to our sponsor Esdebe who helped us prepare this research report.
7. Conclusion
Microglia are critical regulators of brain homeostasis and play complex roles in neurodegenerative diseases. Their inherent heterogeneity and plasticity offer both challenges and opportunities for therapeutic intervention. A nuanced approach that selectively modulates microglial activity, promoting their neuroprotective functions while suppressing their neurotoxic effects, is essential for developing effective therapies for neurodegenerative diseases. Harnessing microglial plasticity and promoting a neuroprotective phenotype represents a promising strategy for preventing or reversing neurodegeneration. Further research is needed to fully understand the complexity of microglial function and to develop safe and effective microglial-targeted therapies for patients with neurodegenerative diseases.
Many thanks to our sponsor Esdebe who helped us prepare this research report.
References
- Aloisi, F. (2001). Immune function of microglia. Glia, 36(2), 165-179.
- Becher, B., Spahn, L., & Miller, S. D. (2017). How CNS-resident myeloid cells orchestrate autoimmune demyelination. Nature Reviews Immunology, 17(8), 502-515.
- Colonna, M., & Butovsky, O. (2017). Microglia function in the central nervous system during health and neurodegeneration. Annual Review of Immunology, 35, 441-468.
- Deczkowska, A., Bar-Or, A., Vilhardt, F., Weiner, H. L., & Schwartz, M. (2018). The role of microglia in brain aging. Aging cell, 17(1), e12732.
- El Khoury, J., & Luster, A. D. (2008). Mechanisms of microglia accumulation in Alzheimer’s disease: role of chemokines and receptors. Frontiers in Bioscience, 13, 6596-6607.
- Ginhoux, F., Greter, M., Leboeuf, M., Nandi, S., See, P., Gokhan, S., … & Merad, M. (2010). Microglia originate from primitive macrophages during development. Immunity, 34(2), 265-278.
- Heneka, M. T., Golenbock, D. T., Latz, E., Morgan, D., Brown, R., McCoy, J. S., … & Kummer, M. P. (2015). The inflammasome in Alzheimer’s disease. Nature, 526(7574), 529-535.
- Keren-Shaul, H., Spinrad, A., Weiner, A., Matcovitch-Natan, O., Dvir-Szternfeld, R., Ulland, T. K., … & Amit, I. (2017). A unique microglia type associated with restricting development of Alzheimer’s disease. Cell, 169(7), 1276-1290. e17.
- Mosher, K. I., Wyss-Coray, T. (2014). Microglial dysfunction in brain aging and Alzheimer’s disease. Biochemical Pharmacology, 88(4), 594-604.
- Prinz, M., Priller, J., Sisodia, S. S., & Ransohoff, R. M. (2011). Microglia regulation in brain aging and neurodegeneration. Nature Neuroscience, 14(3), 277-285.
- Ransohoff, R. M. (2016). How neuroinflammation contributes to neurodegeneration. Science, 353(6297), 777-783.
- Salter, M. W., & Stevens, B. (2017). Microglia prune synapses in the developing nervous system. Neuron, 97(5), 1023-1025.
- Sarlus, R. M., & Heneka, M. T. (2017). Microglia in Alzheimer’s disease. Journal of Clinical Investigation, 127(9), 3240-3249.
- Tremblay, M. E., Stevens, B., Sierra, A., Wake, H., Bessis, A., & Nimmerjahn, A. (2011). The role of microglia in the healthy brain. Journal of Neuroscience, 31(45), 16064-16069.
- Ulland, T. K., Song, W. M., Huang, S. C., Ulrich, J. D., Sergushina, O. A., Onstead, L., … & Colonna, M. (2017). TREM2 functions at the interface of innate immunity and neurodegeneration. Neuron, 94(3), 457-473. e10.
The discussion around microglial polarization (M1/M2 phenotypes) is intriguing. Could further research explore methods to precisely control the degree of polarization, rather than a binary switch? Perhaps a spectrum of phenotypes would allow for more tailored therapeutic interventions.
That’s a great point! Moving beyond the M1/M2 binary is definitely crucial. Exploring the nuances of microglial phenotypes, a spectrum of activation states, could unlock more targeted and effective therapies for neurodegenerative diseases. Understanding the signals that fine-tune microglial responses is an exciting avenue for future research.
Editor: MedTechNews.Uk
Thank you to our Sponsor Esdebe
Wow, that’s a deep dive! Reading about microglia’s Jekyll and Hyde act makes me wonder if we should be offering them tiny lab coats and giving them performance reviews. “Microglia, your neurotoxic tendencies are impacting team morale. Please attend sensitivity training.”