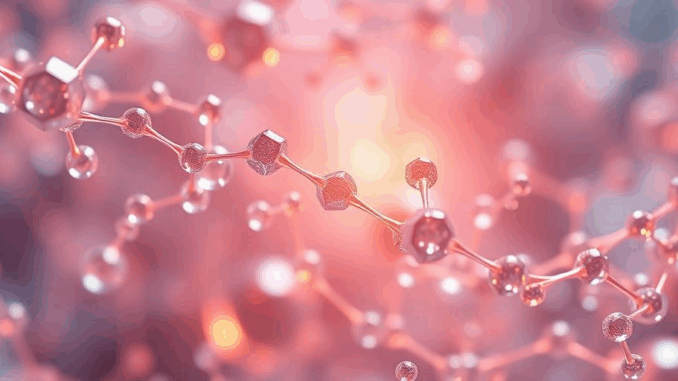
Abstract
Nanoclays, layered silicate minerals with at least one dimension in the nanometer range, have garnered significant attention across various scientific and engineering disciplines. Their unique properties, stemming from their high surface area, layered structure, and tunable surface chemistry, make them versatile building blocks for advanced materials. This report provides a comprehensive overview of nanoclays, encompassing their classification, synthesis methodologies (including advanced and emerging techniques), physicochemical properties, and potential toxicity concerns. A detailed exploration of their applications is presented, spanning from medicine and energy storage to environmental remediation and agricultural advancements. Special emphasis is placed on critically evaluating the current state of knowledge, identifying knowledge gaps, and highlighting future research directions necessary for realizing the full potential of nanoclays in diverse technological domains.
Many thanks to our sponsor Esdebe who helped us prepare this research report.
1. Introduction
Nanoclays represent a diverse family of layered mineral silicates, characterized by dimensions typically ranging from 1 to 100 nanometers in at least one direction. The term encompasses a wide variety of naturally occurring and synthetically produced materials, each possessing distinct structural and chemical compositions. The most common types of nanoclays include montmorillonite, hectorite, bentonite, kaolinite, halloysite, and imogolite, among others. These materials exhibit a layered structure, often described as 2:1 or 1:1 phyllosilicates, where tetrahedral silicate sheets are either sandwiched between or directly bonded to octahedral alumina or magnesia sheets. The stacking of these layers results in interlayer spaces (galleries) that can be modified by the intercalation of various organic and inorganic species, thus tailoring the nanoclay’s properties for specific applications.
The inherent properties of nanoclays, such as their high surface area, high aspect ratio, cation exchange capacity (CEC), and ability to form stable dispersions, make them highly attractive for a wide range of applications. Their ability to act as reinforcing agents in polymer composites, adsorbents for pollutants, carriers for drug delivery, and components in energy storage devices has fueled intense research efforts in recent years. Furthermore, the relatively low cost and abundance of some naturally occurring nanoclays contribute to their economic viability for large-scale industrial applications.
This report aims to provide a comprehensive review of nanoclays, covering their classification, synthesis, properties, toxicity, and applications. By synthesizing the existing knowledge base, highlighting current challenges, and identifying future research directions, this report seeks to provide a valuable resource for researchers, engineers, and scientists working in the field of nanotechnology and advanced materials.
Many thanks to our sponsor Esdebe who helped us prepare this research report.
2. Classification of Nanoclays
Nanoclays can be broadly classified based on their structure, composition, and origin. The primary classification criterion is their layered silicate structure, which dictates their physicochemical properties. Here’s a breakdown of the main types:
-
2:1 Phyllosilicates: These nanoclays consist of two tetrahedral silicate sheets sandwiching an octahedral alumina or magnesia sheet. This category is the most widely studied and utilized. Key examples include:
- Montmorillonite: A smectite clay characterized by a high CEC and swelling capacity due to the presence of exchangeable cations and water molecules in the interlayer space. Isomorphic substitution (e.g., Al3+ replacing Si4+ in the tetrahedral sheet or Mg2+ replacing Al3+ in the octahedral sheet) leads to a net negative charge on the layers, which is compensated by interlayer cations (typically Na+, Ca2+). Montmorillonite is widely used in nanocomposites, drug delivery, and environmental applications.
- Hectorite: Another smectite clay, similar to montmorillonite, but with magnesium as the predominant cation in the octahedral sheet and fluorine substituting for hydroxyl groups. Hectorite exhibits a higher aspect ratio than montmorillonite and is often used in rheology control and cosmetics.
- Bentonite: A naturally occurring clay primarily composed of montmorillonite, often with other minerals present. Bentonite is widely used in construction, agriculture, and as a drilling mud.
- Vermiculite: Similar in structure to smectites, but with a higher layer charge and stronger interlayer bonding. Vermiculite exhibits limited swelling in water but can be exfoliated into thin sheets. It is used in insulation, horticulture, and as a fire retardant.
-
1:1 Phyllosilicates: These nanoclays consist of one tetrahedral silicate sheet and one octahedral alumina sheet. They exhibit lower CEC and swelling capacity compared to 2:1 phyllosilicates.
- Kaolinite: The most common 1:1 clay mineral, characterized by strong hydrogen bonding between the layers, resulting in a relatively stable structure. Kaolinite is widely used in ceramics, paper coating, and pharmaceuticals.
- Halloysite: A tubular-shaped nanoclay with a similar composition to kaolinite. Halloysite nanotubes (HNTs) exhibit a high aspect ratio and can be used as carriers for drug delivery, catalysts, and reinforcing agents.
-
Fibrous Clay Minerals: These nanoclays possess a one-dimensional, fibrous morphology.
- Imogolite: A naturally occurring aluminosilicate with a tubular structure composed of a gibbsite-like sheet and a silanol surface. Imogolite exhibits unique properties, such as a high surface area and excellent adsorption capacity.
-
Synthetic Nanoclays: These nanoclays are produced through various chemical synthesis routes, allowing for precise control over their composition, morphology, and properties. Examples include synthetic hectorite (e.g., Laponite) and layered double hydroxides (LDHs), which are not technically phyllosilicates but are often classified and used similarly to nanoclays.
The classification of nanoclays is crucial for selecting the appropriate material for a specific application. The layered structure, chemical composition, and surface properties of each type of nanoclay dictate its behavior in different environments and its compatibility with other materials.
Many thanks to our sponsor Esdebe who helped us prepare this research report.
3. Synthesis Methods
The synthesis of nanoclays can be achieved through various methods, broadly categorized into natural modification, top-down approaches, and bottom-up approaches. The choice of synthesis method depends on the desired properties of the nanoclay, the scale of production, and the cost-effectiveness of the process.
-
Natural Modification: This approach involves modifying naturally occurring nanoclays to enhance their properties. The most common modification technique is ion exchange, where interlayer cations are replaced with organic cations (e.g., quaternary ammonium salts) to render the nanoclay organophilic. This modification improves the compatibility of nanoclays with organic polymers, facilitating their dispersion in nanocomposites. Acid activation, another modification technique, involves treating nanoclays with strong acids to remove impurities and increase their surface area and acidity.
-
Top-Down Approaches: These methods involve the breakdown of larger clay aggregates into nanoscale particles. Mechanical milling and exfoliation are the primary top-down techniques. Mechanical milling uses high-energy ball mills to reduce the particle size of clays. Exfoliation involves separating the individual layers of a layered clay material using chemical or physical methods. Intercalation of organic molecules or polymers into the interlayer space can facilitate exfoliation.
-
Bottom-Up Approaches: These methods involve the construction of nanoclays from molecular precursors. Hydrothermal synthesis, sol-gel synthesis, and co-precipitation are common bottom-up techniques. Hydrothermal synthesis involves reacting precursors in an aqueous solution under high temperature and pressure to form crystalline nanoclays. Sol-gel synthesis involves the hydrolysis and condensation of metal alkoxides to form a gel, which is then dried and calcined to obtain nanoclays. Co-precipitation involves the simultaneous precipitation of different metal ions from a solution to form layered structures.
Advanced Synthesis Techniques:
-
Microwave-Assisted Synthesis: This technique utilizes microwave irradiation to accelerate the reaction rate and improve the crystallinity of nanoclays. Microwave heating provides uniform and rapid heating, leading to shorter reaction times and higher yields.
-
Template-Assisted Synthesis: This method involves using a template (e.g., surfactants, polymers, or mesoporous materials) to control the morphology and size of nanoclays. The template guides the assembly of precursors into specific structures, such as nanotubes, nanowires, or nanosheets.
-
Layer-by-Layer Assembly: This technique involves the sequential deposition of charged layers onto a substrate to build up multilayered structures. This method allows for precise control over the composition and thickness of the nanoclay film.
The selection of the appropriate synthesis method is crucial for obtaining nanoclays with the desired properties. Bottom-up approaches offer greater control over the particle size, morphology, and composition, but they are often more complex and expensive than top-down approaches. Natural modification is a cost-effective option for enhancing the compatibility of naturally occurring nanoclays with other materials.
Many thanks to our sponsor Esdebe who helped us prepare this research report.
4. Physicochemical Properties
The physicochemical properties of nanoclays dictate their behavior in different environments and their suitability for various applications. Key properties include:
-
Surface Area: Nanoclays possess a high surface area due to their layered structure and small particle size. The high surface area provides a large interface for interactions with other materials, making them effective adsorbents, catalysts, and reinforcing agents.
-
Cation Exchange Capacity (CEC): The CEC is a measure of the amount of exchangeable cations that a nanoclay can hold. Nanoclays with a high CEC can effectively bind and release cations, making them useful in ion exchange applications and as carriers for nutrients and drugs.
-
Swelling Capacity: The ability of a nanoclay to swell in water or other solvents depends on the interlayer spacing and the strength of the interlayer bonding. Smectite clays, such as montmorillonite and hectorite, exhibit high swelling capacity due to the weak interlayer bonding and the presence of exchangeable cations and water molecules in the interlayer space.
-
Particle Size and Morphology: The particle size and morphology of nanoclays influence their dispersion behavior, rheological properties, and reinforcing effect in composites. Nanoclays with a small particle size and high aspect ratio tend to form stable dispersions and exhibit a higher reinforcing effect.
-
Surface Charge: The surface charge of nanoclays is determined by the isomorphic substitution in the layers and the pH of the surrounding environment. Nanoclays typically exhibit a negative surface charge, which allows them to interact with positively charged molecules and surfaces.
-
Thermal Stability: The thermal stability of nanoclays depends on their composition and structure. Nanoclays containing hydroxyl groups can undergo dehydroxylation at high temperatures, leading to a loss of structural water and a change in their properties. Organically modified nanoclays may exhibit lower thermal stability due to the decomposition of the organic modifier.
-
Rheological Properties: Nanoclays can significantly alter the rheological properties of fluids, acting as thickening agents, stabilizers, and anti-settling agents. The rheological behavior of nanoclay dispersions depends on the concentration of the nanoclay, the type of nanoclay, and the presence of other additives.
The properties of nanoclays can be tailored by modifying their chemical composition, surface chemistry, and morphology. This tunability makes them versatile building blocks for advanced materials with specific properties and functionalities.
Many thanks to our sponsor Esdebe who helped us prepare this research report.
5. Potential Toxicity and Environmental Considerations
While nanoclays offer numerous benefits, their potential toxicity and environmental impact require careful consideration. The toxicity of nanoclays depends on several factors, including their size, shape, chemical composition, surface properties, and route of exposure.
-
Inhalation Toxicity: Inhalation of nanoclay particles can lead to respiratory inflammation, fibrosis, and other lung diseases. The small size of nanoclay particles allows them to penetrate deep into the lungs, where they can interact with alveolar cells and trigger an inflammatory response. Studies have shown that some nanoclays, such as halloysite nanotubes, can induce pulmonary toxicity in animal models. However, the toxicity of nanoclays varies depending on their composition and surface properties.
-
Dermal Toxicity: Skin exposure to nanoclays can cause irritation and allergic reactions in some individuals. The high surface area of nanoclays can lead to the adsorption of skin proteins and the disruption of the skin barrier. However, the dermal toxicity of nanoclays is generally considered to be low.
-
Ingestion Toxicity: Ingestion of nanoclays is generally considered to be of low toxicity, as they are poorly absorbed from the gastrointestinal tract. However, some nanoclays can interfere with nutrient absorption or cause gastrointestinal irritation.
-
Environmental Impact: The release of nanoclays into the environment can have adverse effects on aquatic organisms and soil ecosystems. Nanoclays can adsorb pollutants and transport them through the environment, potentially contaminating water sources and soil. The long-term environmental impact of nanoclays is still under investigation.
Mitigation Strategies:
-
Encapsulation: Encapsulating nanoclays within a matrix or coating can reduce their exposure to the environment and minimize their potential toxicity.
-
Surface Modification: Modifying the surface of nanoclays with biocompatible and biodegradable materials can reduce their toxicity and improve their compatibility with biological systems.
-
Proper Handling and Disposal: Implementing proper handling and disposal procedures can minimize the release of nanoclays into the environment.
-
Risk Assessment: Conducting thorough risk assessments before using nanoclays in new applications can help identify and mitigate potential hazards.
It is crucial to conduct comprehensive toxicity studies and environmental risk assessments to ensure the safe and responsible use of nanoclays in various applications. Further research is needed to fully understand the long-term health and environmental effects of nanoclays.
Many thanks to our sponsor Esdebe who helped us prepare this research report.
6. Applications Across Diverse Fields
Nanoclays have found widespread applications across various fields, owing to their unique properties and versatility. Some key applications include:
-
Polymer Nanocomposites: Nanoclays are widely used as reinforcing agents in polymer nanocomposites. Their high surface area and aspect ratio enhance the mechanical properties, thermal stability, and barrier properties of polymers. Nanoclay-polymer nanocomposites are used in automotive parts, packaging materials, coatings, and adhesives.
-
Drug Delivery: Nanoclays can be used as carriers for drug delivery, protecting drugs from degradation and delivering them to specific target sites. Their high surface area allows for the adsorption of large amounts of drug molecules, and their layered structure allows for the controlled release of drugs. Halloysite nanotubes (HNTs) are particularly attractive for drug delivery due to their biocompatibility and ability to encapsulate drugs within their lumen.
-
Environmental Remediation: Nanoclays are effective adsorbents for pollutants, such as heavy metals, organic dyes, and pesticides. Their high surface area and CEC allow them to bind and remove pollutants from water and soil. Nanoclays can also be used as catalysts for the degradation of pollutants.
-
Energy Storage: Nanoclays are used as electrode materials and separators in batteries and supercapacitors. Their high surface area and ion conductivity enhance the performance of energy storage devices. Nanoclays can also be used to stabilize electrolytes and improve the safety of batteries.
-
Catalysis: Nanoclays can be used as supports for catalysts, providing a high surface area for catalytic reactions. Their layered structure and ability to intercalate metal ions make them ideal supports for heterogeneous catalysts. Nanoclays can also act as catalysts themselves, promoting various chemical reactions.
-
Cosmetics and Personal Care Products: Nanoclays are used as thickening agents, stabilizers, and absorbents in cosmetics and personal care products. Their ability to form stable dispersions and their non-toxic nature make them suitable for use in these products.
-
Agriculture: Nanoclays can be used to improve soil fertility, enhance plant growth, and deliver nutrients and pesticides to plants. Their ability to retain water and nutrients can improve soil water retention and reduce fertilizer runoff. Nanoclays can also be used to encapsulate pesticides and release them slowly, reducing the environmental impact of pesticides.
-
Construction Materials: Nanoclays can be added to cement and concrete to improve their mechanical properties, durability, and resistance to cracking. Their high surface area and ability to hydrate cement particles can enhance the strength and reduce the permeability of cement-based materials.
Specific Examples and Emerging Applications:
-
Wound Healing: Nanoclay-based hydrogels are being explored for wound healing applications due to their ability to promote cell proliferation, angiogenesis, and tissue regeneration. Montmorillonite, in particular, has demonstrated promising results in accelerating wound closure and reducing scar formation.
-
Sensors: Nanoclays are being utilized in the development of sensors for detecting gases, chemicals, and biomolecules. Their high surface area and ability to bind target molecules make them sensitive and selective sensing materials. For example, nanoclay-modified electrodes can be used to detect heavy metals in water.
-
Membranes: Nanoclay membranes are being developed for water purification, gas separation, and other separation applications. Their layered structure and ability to control the pore size allow for the selective transport of molecules. Nanoclay membranes can be used to remove pollutants from water or separate gases with high efficiency.
-
3D Printing: Nanoclays are being incorporated into 3D printing filaments to improve the mechanical properties and printability of printed objects. Nanoclay-reinforced filaments can be used to create strong and lightweight parts for various applications.
The applications of nanoclays are constantly expanding as researchers discover new ways to utilize their unique properties. Future research will focus on developing new nanoclay-based materials with enhanced properties and functionalities for specific applications.
Many thanks to our sponsor Esdebe who helped us prepare this research report.
7. Future Research Directions and Challenges
While nanoclays have demonstrated significant potential in various fields, several challenges and research gaps remain that need to be addressed to fully realize their potential. Some key areas for future research include:
-
Understanding the Structure-Property Relationships: A deeper understanding of the relationship between the structure, composition, and properties of nanoclays is needed to design and synthesize materials with specific functionalities. This requires advanced characterization techniques and computational modeling.
-
Developing Scalable and Cost-Effective Synthesis Methods: Developing scalable and cost-effective synthesis methods for nanoclays is crucial for their widespread industrial adoption. This includes exploring new synthesis routes and optimizing existing methods.
-
Addressing Toxicity Concerns: Comprehensive toxicity studies are needed to assess the potential health and environmental risks associated with nanoclays. This includes investigating the mechanisms of toxicity and developing strategies to mitigate these risks.
-
Improving Dispersion and Compatibility: Improving the dispersion and compatibility of nanoclays with other materials is essential for their use in nanocomposites and other applications. This requires developing new surface modification techniques and optimizing processing conditions.
-
Developing Advanced Nanoclay-Based Materials: Developing advanced nanoclay-based materials with enhanced properties and functionalities is crucial for expanding their applications. This includes exploring new combinations of nanoclays with other materials and developing new fabrication techniques.
-
Long-Term Stability and Durability: Investigating the long-term stability and durability of nanoclay-based materials under various environmental conditions is essential for their use in demanding applications. This requires accelerated aging tests and performance monitoring.
-
Regulatory Frameworks: Establishing clear regulatory frameworks for the use of nanoclays in various applications is crucial for ensuring their safe and responsible use. This requires collaboration between researchers, industry, and regulatory agencies.
Addressing these challenges and pursuing these research directions will pave the way for the development of new and innovative nanoclay-based materials with a wide range of applications. The future of nanoclays is bright, with the potential to revolutionize various industries and improve the quality of life.
Many thanks to our sponsor Esdebe who helped us prepare this research report.
8. Conclusion
Nanoclays represent a versatile class of materials with a wide range of applications across diverse fields. Their unique properties, such as high surface area, cation exchange capacity, and ability to form stable dispersions, make them attractive for use in polymer nanocomposites, drug delivery, environmental remediation, energy storage, and catalysis. While significant progress has been made in the synthesis, characterization, and application of nanoclays, several challenges remain that need to be addressed to fully realize their potential. Future research should focus on understanding the structure-property relationships, developing scalable and cost-effective synthesis methods, addressing toxicity concerns, improving dispersion and compatibility, developing advanced nanoclay-based materials, and establishing clear regulatory frameworks. By addressing these challenges, researchers and engineers can unlock the full potential of nanoclays and pave the way for their widespread use in various industries.
Many thanks to our sponsor Esdebe who helped us prepare this research report.
References
[1] Bergaya, F., Theng, B. K. G., & Lagaly, G. (Eds.). (2006). Handbook of Clay Science. Elsevier.
[2] Murray, H. H. (2006). Structure and composition of the clay minerals and their physical and chemical properties. Developments in Clay Science, 1, 7-28.
[3] Alexandre, M., & Dubois, P. (2000). Polymer-layered silicate nanocomposites: Preparation, properties and uses of a new class of materials. Materials Science and Engineering: R: Reports, 28(1-2), 1-63.
[4] Rawtani, D., Agrawal, Y. K., & Hussain, C. M. (2018). Nanomaterials: A toxicological perspective. Royal Society Open Science, 5(2), 171785.
[5] Hussain, C. M., Brustad, E. M., Hussain, I., Ahsan, A., Erali, R., Werner-Zwanziger, U., … & Kumar, S. (2017). Halloysite nanotubes for biomedical applications: State of the art and future prospects. Applied Clay Science, 146, 73-86.
[6] Carreño, N. L. V., Pereira, M. F. R., & Figueiredo, J. L. (2013). Enhanced adsorption of pharmaceuticals from water by organo-modified clays. Chemical Engineering Journal, 225, 49-56.
[7] Lv, L., He, J., Wei, M., Evans, D. G., & Duan, X. (2006). Layered double hydroxides: Synthesis, characterization, properties and applications. Chemical Engineering Journal, 140(1-3), 1-21.
[8] Ruiz-Hitzky, E., Aranda, P., & Darder, M. (2011). Functional biopolymer nanocomposites based on clay minerals. Trends in Biotechnology, 29(11), 592-602.
[9] Gaharwar, A. K., Arepalli, S., Ramirez, C. M., Nguyen, A. T., & Lee, E. J. (2014). Injectable and cytocompatible nanocomposite hydrogels for bone tissue engineering. ACS Nano, 8(1), 269-279.
[10] Darder, M., López-Blanco, M., & Ruiz-Hitzky, E. (2007). Clay mineral-drug delivery systems. Expert Opinion on Drug Delivery, 4(6), 695-713.
[11] Rana, A., Kumar, S., Thakur, V. K., & Karak, N. (2019). Nanoclay reinforced polymer nanocomposites: Preparation, properties and applications. Polymer Composites, 40(11), 4141-4163.
[12] Mohamed, R. M., Ismail, A. A., & Othman, M. R. (2015). Nanoclay reinforced polymer nanocomposites for energy storage applications: A review. Renewable and Sustainable Energy Reviews, 47, 549-567.
[13] Zhou, C. H., & Keeling, J. (2003). Clay‐supported catalysts. Applied Catalysis A: General, 243(1-2), 1-53.
The report mentions the increasing use of nanoclays in cosmetics. Given the potential for dermal toxicity, what specific modifications or coatings are proving most effective in minimizing skin irritation while maintaining the desired functional properties in cosmetic applications?
That’s a great question! The modification of nanoclays with biocompatible polymers like hyaluronic acid or phospholipids seems promising. These coatings not only reduce direct skin contact but can also enhance the delivery of active cosmetic ingredients. Further research into tailored surface modifications will be key for safe and effective applications.
Editor: MedTechNews.Uk
Thank you to our Sponsor Esdebe