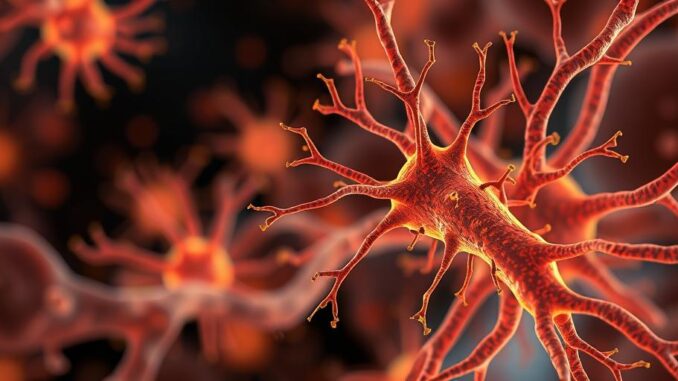
Abstract
Neuroimmunology has emerged as a crucial field, elucidating the intricate bidirectional communication between the nervous and immune systems. This cross-disciplinary field investigates the impact of immune processes on neurological function and, conversely, the influence of the nervous system on immune responses. Beyond the traditional view of the brain as an immune-privileged site, evidence now demonstrates the active role of resident immune cells, particularly microglia, and the infiltration of peripheral immune cells in modulating neuronal activity, synaptic plasticity, and overall brain homeostasis. Dysregulation of these neuroimmune interactions is increasingly implicated in a wide spectrum of neurological, psychiatric, and systemic inflammatory disorders. This report provides a comprehensive overview of the fundamental principles of neuroimmunology, including the key cellular and molecular mediators involved in neuroimmune communication. It delves into the roles of neuroinflammation, autoimmunity, and the gut-brain axis in the pathogenesis of diverse conditions such as multiple sclerosis, Alzheimer’s disease, Parkinson’s disease, autism spectrum disorder, and major depressive disorder. Furthermore, the report critically evaluates current research methodologies and highlights promising therapeutic strategies targeting neuroimmune pathways for disease modification and treatment.
Many thanks to our sponsor Esdebe who helped us prepare this research report.
1. Introduction
The convergence of neuroscience and immunology has led to the establishment of neuroimmunology, a field dedicated to understanding the complex interplay between the nervous and immune systems. Historically, the brain was considered an immune-privileged site due to the presence of the blood-brain barrier (BBB), which restricts the entry of peripheral immune cells and large molecules. However, this concept has been challenged by accumulating evidence demonstrating that the brain is not entirely isolated from the immune system and that immune cells and mediators can actively participate in maintaining brain homeostasis and influencing neuronal function [1].
The dynamic communication between the nervous and immune systems involves a diverse array of signaling molecules, including cytokines, chemokines, neurotransmitters, and neuropeptides. These molecules mediate bidirectional interactions, allowing the brain to modulate immune responses and the immune system to influence neuronal activity and behavior. Disruptions in these neuroimmune interactions are increasingly recognized as critical factors in the pathogenesis of various neurological and psychiatric disorders, including multiple sclerosis (MS), Alzheimer’s disease (AD), Parkinson’s disease (PD), autism spectrum disorder (ASD), and major depressive disorder (MDD) [2].
Furthermore, systemic inflammatory conditions, such as rheumatoid arthritis and inflammatory bowel disease, are often associated with neurological and psychiatric comorbidities, highlighting the systemic impact of inflammation on brain function. The gut-brain axis, a bidirectional communication pathway between the gastrointestinal tract and the brain, also plays a crucial role in neuroimmunology, with gut microbiota influencing immune responses and brain function through various mechanisms [3].
This report aims to provide a comprehensive overview of the fundamental principles of neuroimmunology, focusing on the cellular and molecular mechanisms underlying neuroimmune communication and the role of neuroimmune dysregulation in the pathogenesis of diverse neurological, psychiatric, and systemic inflammatory disorders. The report will also explore current research methodologies and potential therapeutic strategies targeting neuroimmune pathways for disease modification and treatment.
Many thanks to our sponsor Esdebe who helped us prepare this research report.
2. Fundamental Principles of Neuroimmunology
The core of neuroimmunology rests on several key principles that govern the interaction between the nervous and immune systems. Understanding these principles is essential for comprehending the complexities of neuroimmune regulation and its implications for health and disease.
2.1 Bidirectional Communication
The nervous and immune systems engage in a reciprocal dialogue, wherein each system can influence the function of the other. The brain, through the hypothalamic-pituitary-adrenal (HPA) axis and the autonomic nervous system (ANS), can modulate immune responses. For example, stress-induced activation of the HPA axis leads to the release of glucocorticoids, which suppress immune cell activity. The ANS, via sympathetic and parasympathetic pathways, can also influence immune cell trafficking and cytokine production [4].
Conversely, the immune system can impact brain function through various mechanisms. Peripheral immune cells, such as macrophages and T cells, can migrate into the brain under certain conditions, particularly during inflammation. These cells can release cytokines and chemokines that directly affect neuronal activity, synaptic plasticity, and neurotransmitter release. In addition, circulating antibodies can cross the BBB and bind to neuronal targets, leading to neuronal dysfunction or even cell death [5].
2.2 Immune Cells in the Brain
The brain contains a unique population of resident immune cells, primarily microglia, which are the resident macrophages of the central nervous system (CNS). Microglia play a crucial role in maintaining brain homeostasis, clearing cellular debris, and shaping synaptic connections. Under normal conditions, microglia exist in a resting or surveying state, continuously monitoring the brain microenvironment. However, upon activation by inflammatory stimuli or neuronal damage, microglia undergo morphological and functional changes, becoming reactive and releasing cytokines, chemokines, and other inflammatory mediators [6].
In addition to microglia, other immune cells, such as astrocytes and perivascular macrophages, also contribute to the neuroimmune response. Astrocytes, the most abundant glial cells in the brain, can produce cytokines and chemokines and interact with microglia to regulate inflammation. Perivascular macrophages, located in the perivascular space around blood vessels in the brain, act as the first line of defense against pathogens and can initiate inflammatory responses [7].
2.3 Neuroinflammation
Neuroinflammation is a complex process involving the activation of immune cells in the brain and the release of inflammatory mediators. While a certain degree of inflammation is necessary for tissue repair and defense against pathogens, chronic or excessive neuroinflammation can be detrimental to neuronal function and contribute to neurodegeneration. Neuroinflammation is characterized by the activation of microglia and astrocytes, the infiltration of peripheral immune cells, and the production of pro-inflammatory cytokines, such as TNF-α, IL-1β, and IL-6 [8].
These inflammatory mediators can directly damage neurons by inducing oxidative stress, excitotoxicity, and apoptosis. Furthermore, neuroinflammation can disrupt synaptic plasticity, impair neurotransmitter signaling, and compromise the integrity of the BBB. Chronic neuroinflammation is implicated in the pathogenesis of various neurological and psychiatric disorders, including AD, PD, MS, and MDD [9].
2.4 The Blood-Brain Barrier (BBB)
The BBB is a specialized structure that regulates the passage of molecules and cells between the bloodstream and the brain. It is formed by endothelial cells that line the brain capillaries, which are tightly connected by tight junctions, restricting paracellular permeability. The BBB also contains specialized transport proteins that selectively allow the passage of essential nutrients and exclude harmful substances. While the BBB provides protection for the brain, it can also be a barrier to the delivery of therapeutic agents [10].
In neuroinflammatory conditions, the BBB can become compromised, allowing the entry of peripheral immune cells and inflammatory mediators into the brain. This BBB disruption can further exacerbate neuroinflammation and contribute to neuronal damage. Several factors can compromise the BBB, including inflammation, oxidative stress, and the release of matrix metalloproteinases (MMPs), which degrade the extracellular matrix surrounding the brain capillaries [11].
Many thanks to our sponsor Esdebe who helped us prepare this research report.
3. Neuroimmunology in Neurological Disorders
The study of neuroimmunology has provided valuable insights into the pathogenesis of various neurological disorders. Dysregulation of neuroimmune interactions is increasingly recognized as a key factor in the development and progression of these conditions.
3.1 Multiple Sclerosis (MS)
MS is a chronic autoimmune disease characterized by inflammation and demyelination in the CNS. The immune system mistakenly attacks the myelin sheath, the protective covering around nerve fibers, leading to neuronal damage and impaired neurological function. T cells and B cells play a critical role in the pathogenesis of MS. Autoreactive T cells infiltrate the brain and release inflammatory cytokines, which activate microglia and macrophages, leading to myelin degradation. B cells produce antibodies that target myelin proteins, further contributing to demyelination [12].
Neuroinflammation is a prominent feature of MS, with activated microglia and astrocytes contributing to neuronal damage. The BBB is also compromised in MS, allowing the entry of peripheral immune cells and inflammatory mediators into the brain. Current treatments for MS primarily focus on suppressing the immune system to reduce inflammation and prevent further demyelination [13].
3.2 Alzheimer’s Disease (AD)
AD is a neurodegenerative disorder characterized by the progressive decline in cognitive function. The hallmark pathological features of AD are the accumulation of amyloid plaques and neurofibrillary tangles in the brain. While the exact cause of AD is still unknown, neuroinflammation is increasingly recognized as a key contributor to the disease process. Microglia, the resident immune cells of the brain, become activated in AD and release inflammatory cytokines, which can contribute to neuronal damage and synaptic dysfunction [14].
Studies have shown that genetic variations in immune-related genes, such as TREM2, are associated with an increased risk of AD. TREM2 is a receptor expressed on microglia that plays a role in phagocytosis and clearance of amyloid plaques. Dysfunctional TREM2 can impair microglia’s ability to clear amyloid plaques, leading to their accumulation in the brain. Furthermore, peripheral inflammation has been shown to exacerbate AD pathology [15].
3.3 Parkinson’s Disease (PD)
PD is a neurodegenerative disorder characterized by the loss of dopaminergic neurons in the substantia nigra, a region of the brain involved in motor control. The pathological hallmark of PD is the presence of Lewy bodies, intracellular inclusions composed of aggregated alpha-synuclein protein. Neuroinflammation is increasingly recognized as a key factor in the pathogenesis of PD. Activated microglia and astrocytes release inflammatory cytokines, which can contribute to neuronal damage and the progression of the disease [16].
Studies have shown that peripheral inflammation can exacerbate PD symptoms and accelerate disease progression. Furthermore, the gut microbiota may play a role in PD pathogenesis, with altered gut microbial composition contributing to inflammation and alpha-synuclein aggregation [17].
Many thanks to our sponsor Esdebe who helped us prepare this research report.
4. Neuroimmunology in Psychiatric Disorders
The role of neuroimmunology extends beyond neurological disorders and encompasses various psychiatric conditions. Emerging evidence suggests that immune dysregulation and neuroinflammation contribute to the pathogenesis of psychiatric disorders such as autism spectrum disorder (ASD) and major depressive disorder (MDD).
4.1 Autism Spectrum Disorder (ASD)
ASD is a neurodevelopmental disorder characterized by impaired social interaction, communication difficulties, and repetitive behaviors. While the exact cause of ASD is still unknown, genetic and environmental factors are believed to play a role. Neuroinflammation is increasingly recognized as a potential contributor to the pathogenesis of ASD. Studies have shown that children with ASD have elevated levels of inflammatory cytokines in their blood and cerebrospinal fluid [18].
Microglial activation has also been observed in the brains of individuals with ASD, suggesting that neuroinflammation may contribute to the neurodevelopmental abnormalities associated with the disorder. Furthermore, the gut microbiota may play a role in ASD pathogenesis, with altered gut microbial composition contributing to inflammation and behavioral abnormalities [19].
4.2 Major Depressive Disorder (MDD)
MDD is a mood disorder characterized by persistent feelings of sadness, loss of interest, and decreased energy. While the exact cause of MDD is still unknown, genetic, environmental, and psychological factors are believed to play a role. Neuroinflammation is increasingly recognized as a potential contributor to the pathogenesis of MDD. Studies have shown that individuals with MDD have elevated levels of inflammatory cytokines in their blood [20].
Inflammatory cytokines can affect neurotransmitter metabolism, HPA axis function, and neuroplasticity, all of which are implicated in the pathophysiology of MDD. Furthermore, antidepressant treatments have been shown to have anti-inflammatory effects, suggesting that targeting neuroimmune pathways may be a potential therapeutic strategy for MDD [21]. The impact of the gut microbiome on the brain and on immune cells has also been proposed as a factor that might affect mental disorders such as MDD, and is an area of ongoing research [22].
Many thanks to our sponsor Esdebe who helped us prepare this research report.
5. Research Methods and Techniques in Neuroimmunology
The study of neuroimmunology relies on a diverse range of research methods and techniques, including:
- Animal Models: Animal models, such as experimental autoimmune encephalomyelitis (EAE) for MS and transgenic mouse models for AD and PD, are used to study the pathogenesis of neurological and psychiatric disorders and to test potential therapeutic interventions.
- Cellular and Molecular Assays: Cellular and molecular assays, such as ELISA, flow cytometry, and immunohistochemistry, are used to measure cytokine levels, immune cell populations, and protein expression in biological samples.
- Neuroimaging Techniques: Neuroimaging techniques, such as MRI, PET, and SPECT, are used to visualize brain structure, function, and inflammation in vivo.
- Genetics and Genomics: Genetic and genomic studies are used to identify genetic variations and gene expression patterns associated with neuroimmune disorders.
- Microbiome Analysis: Analyzing the composition and function of the gut microbiome has become a critical tool in neuroimmunology research, especially in understanding the gut-brain axis and its influence on neurological and psychiatric conditions. Techniques like 16S rRNA gene sequencing and metagenomics provide insights into microbial diversity and potential pathogenic roles [23].
- Single-Cell Sequencing: Single-cell sequencing technologies, particularly transcriptomics and proteomics, are revolutionizing neuroimmunology research. These approaches allow researchers to analyze the gene expression profiles and protein compositions of individual immune and neuronal cells within the brain, offering unprecedented insights into cellular heterogeneity and dynamic responses to inflammation and disease [24].
Many thanks to our sponsor Esdebe who helped us prepare this research report.
6. Therapeutic Targets in Neuroimmunology
The growing understanding of neuroimmune mechanisms has opened up new avenues for therapeutic intervention in neurological and psychiatric disorders. Several potential therapeutic targets have been identified, including:
- Cytokine Inhibitors: Targeting inflammatory cytokines, such as TNF-α and IL-1β, with specific inhibitors can reduce neuroinflammation and protect neurons from damage. However, systemic cytokine inhibition can have broad immunosuppressive effects, increasing the risk of infections.
- Microglia Modulators: Modulating microglial activation with drugs that promote a neuroprotective phenotype can reduce neuroinflammation and promote neuronal survival. For example, TREM2 agonists may enhance microglial clearance of amyloid plaques in AD.
- BBB Modulators: Developing strategies to protect the BBB from damage and enhance drug delivery to the brain can improve therapeutic outcomes in neuroimmune disorders. For example, nanoparticles can be used to deliver drugs across the BBB specifically to target cells [25].
- Gut Microbiome Modulation: Modulating the gut microbiome with probiotics, prebiotics, or fecal microbiota transplantation (FMT) can reduce inflammation and improve brain function in neurological and psychiatric disorders. FMT involves transplanting fecal material from a healthy donor into a recipient, aiming to restore a balanced gut microbiome [26]. However, the long-term effects and safety of FMT in neurological and psychiatric disorders require further investigation. This approach offers the potential to influence the immune system and potentially impact disease progression and symptom management.
- Autophagy Modulation: Enhancing autophagy, a cellular process that removes damaged proteins and organelles, can reduce inflammation and promote neuronal survival. Autophagy is crucial for maintaining cellular homeostasis and preventing the accumulation of toxic aggregates that contribute to neurodegeneration. Drugs that stimulate autophagy, such as rapamycin analogs (rapalogs), show promise in reducing inflammation and promoting neuronal survival, however, the therapeutic potential of autophagy modulation in neuroimmunological disorders is still being investigated in clinical trials [27].
Many thanks to our sponsor Esdebe who helped us prepare this research report.
7. Challenges and Future Directions
Despite significant advances in neuroimmunology, several challenges remain. One of the major challenges is the complexity of neuroimmune interactions. The brain is a highly heterogeneous organ with diverse cell types and signaling pathways, making it difficult to dissect the specific mechanisms underlying neuroimmune dysregulation. Another challenge is the lack of reliable biomarkers for neuroimmune disorders. Biomarkers that can accurately reflect disease activity, progression, and treatment response are needed to facilitate early diagnosis and personalized treatment.
Future research in neuroimmunology should focus on:
- Identifying novel therapeutic targets: Identifying new therapeutic targets that specifically modulate neuroimmune interactions without causing systemic immunosuppression.
- Developing personalized treatment strategies: Developing personalized treatment strategies based on individual patient characteristics and disease mechanisms.
- Understanding the role of the gut microbiome: Further elucidating the role of the gut microbiome in neuroimmune disorders and developing targeted interventions to modulate the gut-brain axis.
- Translational research: Translating basic research findings into clinical applications to improve the diagnosis, treatment, and prevention of neuroimmune disorders.
- Advanced Imaging Techniques: Developing and implementing more advanced imaging techniques that can visualize neuroinflammation and immune cell activity in real-time and with higher resolution [28].
Many thanks to our sponsor Esdebe who helped us prepare this research report.
8. Conclusion
Neuroimmunology has emerged as a critical field for understanding the complex interplay between the nervous and immune systems in health and disease. Dysregulation of neuroimmune interactions is implicated in the pathogenesis of a wide range of neurological, psychiatric, and systemic inflammatory disorders. Continued research in neuroimmunology promises to uncover novel therapeutic targets and strategies for treating and preventing these debilitating conditions. By integrating knowledge from neuroscience and immunology, neuroimmunology offers the potential to revolutionize the understanding and treatment of disorders affecting the brain and the immune system.
Many thanks to our sponsor Esdebe who helped us prepare this research report.
References
[1] Ransohoff, R. M., & Engelhardt, B. (2012). The anatomical and cellular basis of immune surveillance in the central nervous system. Nature Reviews Immunology, 12(9), 623-635.
[2] Bechter, K. (2019). Neuroimmunological aspects of psychiatric disorders. Molecular Psychiatry, 24(1), 24-45.
[3] Cryan, J. F., O’Riordan, K. J., Cowan, C. S., Sandhu, K. V., Bastiaanssen, T. F., Boehme, M., … & Dinan, T. G. (2019). The microbiota-gut-brain axis. Physiological Reviews, 99(4), 1877-2013.
[4] Tracey, K. J. (2002). The inflammatory reflex. Nature, 420(6917), 853-859.
[5] Steinman, L. (2004). Immunology of neurological disorders. Nature Immunology, 5(6), 575-582.
[6] Hanisch, U. K. (2002). Microglia as a source and target of cytokines. Glia, 40(2), 140-155.
[7] Ransohoff, R. M., & Perry, V. H. (2009). Microglial physiology: unique stimuli, specialized responses. Annual Review of Immunology, 27, 119-145.
[8] Amor, S., Peferoen, L. A., Vogel, D. Y., Breur, M., van der Valk, P., Baker, D., … & Dijkstra, C. D. (2014). Inflammation in neurodegenerative diseases. Immunology, 142(2), 151-166.
[9] Glass, C. K., Saijo, K., Winner, B., Marchetto, M. C., & Gage, F. H. (2010). Mechanisms underlying inflammation in neurodegeneration. Cell, 140(6), 918-934.
[10] Daneman, R., & Prat, A. (2015). The blood-brain barrier. Cold Spring Harbor Perspectives in Biology, 7(1), a020412.
[11] Abbott, N. J., Patabendige, A. A., Dolman, D. E., Yusof, S. R., & Begley, D. J. (2010). Structure and function of the blood-brain barrier. Xenobiotica, 40(7), 498-527.
[12] Frohman, E. M., Racke, M. K., & Raine, C. S. (2006). Multiple sclerosis—the plaque and its pathogenesis. New England Journal of Medicine, 354(9), 942-955.
[13] Compston, A., & Coles, A. (2008). Multiple sclerosis. The Lancet, 372(9648), 1502-1517.
[14] McGeer, P. L., Schulzer, M., & McGeer, E. G. (2016). Inflammation in neurodegenerative diseases. Cold Spring Harbor Perspectives in Biology, 8(7), a024976.
[15] Guerreiro, R., & Hardy, J. (2013). TREM2 is mutated in nasal-frontal lobe dementia with bone cysts. Neurobiology of Aging, 34(5), 1202-1203.
[16] Tansey, M. G., Wallings, R. L., Houser, M. C., Herrick, M. K., Park, J. S., Graybiel, A. M., & Kabani, N. (2022). Inflammation and immune dysregulation in Parkinson disease. Nature Reviews Neuroscience, 23(9), 559-577.
[17] Sampson, T. R., Debelius, J. W., Thron, T., Janssen, S., Shastri, G. G., Ilhan, Z. E., … & Mazmanian, S. K. (2016). Gut microbiota regulate motor deficits and neuroinflammation in a model of Parkinson’s disease. Cell, 167(6), 1469-1480. e12.
[18] Careaga, M., Van de Sande, L., & Ashwood, P. (2017). The role of the immune system in autism spectrum disorder. Biological Psychiatry, 81(6), 487-498.
[19] Hsiao, E. Y., McBride, S. W., Hsien, S., Sharon, G., Hyde, E. R., McCue, T., … & Mazmanian, S. K. (2013). Microbiota modulate behavioral and physiological abnormalities associated with neurodevelopmental disorders. Cell, 155(7), 1451-1463.
[20] Dowlati, Y., Ravindran, A. V., Coogan, A. N., Grunau, R. E., Gilead, M., Frey, B. N., … & McIntyre, R. S. (2010). Cytokines in major depression: a systematic review and meta-analysis. Molecular Psychiatry, 15(5), 520-542.
[21] Kohler, O., Krogh, J., Mors, O., & Benros, M. E. (2016). Inflammation in depression and the potential for anti-inflammatory treatment. Current Neuropharmacology, 14(7), 732-742.
[22] Kelly, J. R., Kennedy, P. J., Cryan, J. F., Dinan, T. G., Clarke, G., & Hyland, N. P. (2015). The influence of gut microbiota on brain and behaviour: a bidirectional communications pathway. Gastroenterology, 149(7), 1739-1752.
[23] Gilbert, J. A., Blaser, M. J., Caporaso, J. G., Jansson, J. K., Lynch, S. V., & Knight, R. (2018). Current understanding of the human microbiome. Nature Medicine, 24(4), 392-400.
[24] Saliba, J., Westermann, F., Gorski, S. A., & Kim-Anh, N. T. (2014). RNA-seq technologies for transcriptomic profiling of cancer cells. Cellular and Molecular Life Sciences, 71(24), 5475-5495.
[25] Kreuter, J. (2014). Nanoparticles—a historical perspective. International Journal of Pharmaceutics, 471(1-2), 138-146.
[26] Khoruts, A., Dicksved, J., Jansson, J. K., & Sadowsky, M. J. (2010). Changes in the human gut microbiome associated with Clostridium difficile infection. Clinical Infectious Diseases, 50(2), S109-S1121.
[27] Menzies, F. M., Fleming, A., Rubinsztein, D. C. (2015). Autophagy and neurodegeneration: why it matters for neurotherapeutics. Nature Reviews Neuroscience, 16(6), 365–379.
[28] Mushtaq, F., Soon, D., Sachinowicz, L., Brück, W., & Stadelmann, C. (2021). Advanced Imaging Modalities in Multiple Sclerosis: Illuminating Neuroinflammation and Neurodegeneration. Cells, 10(9), 2457.
This report highlights the potential of modulating the gut microbiome with FMT for neurological disorders. Research into personalized FMT protocols, considering individual microbiome profiles and disease states, could optimize therapeutic efficacy and minimize risks.
Thanks for highlighting the potential of personalized FMT protocols! Considering individual microbiome profiles is definitely a crucial next step. Further research in this area will be key to unlocking more effective and safer treatments for neurological disorders. It will be interesting to see this develop.
Editor: MedTechNews.Uk
Thank you to our Sponsor Esdebe
The report mentions single-cell sequencing offering insights into cellular heterogeneity in the brain. Could this level of detail lead to the discovery of novel, cell-specific therapeutic targets within the neuroimmune system?
That’s a fantastic point! Absolutely, the detailed resolution offered by single-cell sequencing holds immense promise. By identifying unique molecular signatures within specific cell types involved in neuroimmune processes, we can potentially develop highly targeted therapies with fewer off-target effects. This precision could revolutionize treatment strategies.
Editor: MedTechNews.Uk
Thank you to our Sponsor Esdebe
So, the brain’s not an immune-privileged site after all? Sounds like the blood-brain barrier needs a serious upgrade! Maybe a bouncer with a guest list? I wonder if we can train microglia to be more discerning about who gets in.
That’s a fun analogy! The idea of training microglia as discerning ‘bouncers’ is quite interesting. Research is exploring ways to modulate microglial activity to promote neuroprotection and reduce harmful inflammation. Perhaps targeted therapies could one day help them make better decisions about what’s friend or foe!
Editor: MedTechNews.Uk
Thank you to our Sponsor Esdebe