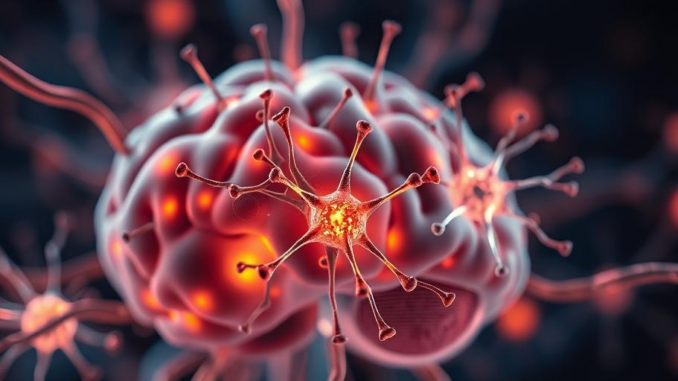
Abstract
Parkinson’s disease (PD) is a progressive neurodegenerative disorder characterized by the cardinal motor features of bradykinesia, rigidity, tremor, and postural instability. The underlying pathophysiology involves the degeneration of dopaminergic neurons in the substantia nigra pars compacta (SNpc), leading to dopamine depletion in the striatum. While current therapies, including pharmacological interventions such as levodopa and deep brain stimulation (DBS), offer symptomatic relief, they do not halt disease progression and often result in debilitating side effects, including motor fluctuations and dyskinesias. Furthermore, non-motor symptoms (NMS), such as cognitive impairment, sleep disturbances, and autonomic dysfunction, significantly impact patients’ quality of life and are often refractory to conventional treatments. This review provides a comprehensive overview of the pathophysiology of PD, current treatment strategies, the challenges in managing motor and NMS, and emerging therapeutic avenues beyond dopamine replacement, including gene therapies, neuroprotective strategies, and novel approaches targeting gait freezing and other debilitating symptoms. We also assess the current landscape of clinical trials evaluating these promising interventions, focusing on the challenges and future directions in PD research and clinical management.
Many thanks to our sponsor Esdebe who helped us prepare this research report.
1. Introduction
Parkinson’s disease (PD) is a chronic, progressive neurodegenerative disorder affecting millions worldwide. Characterized by a constellation of motor and non-motor symptoms, PD significantly impacts the quality of life of affected individuals and poses a substantial burden on healthcare systems. The etiology of PD is complex and multifactorial, involving both genetic and environmental factors. While advancements in understanding the disease have led to improved symptomatic treatments, a cure remains elusive, and many patients experience a decline in response to medication over time, coupled with the development of debilitating motor complications and non-motor features. This review aims to provide an in-depth examination of the pathophysiology of PD, discuss the limitations of current therapeutic approaches, explore the challenges in managing both motor and non-motor symptoms, and highlight emerging therapeutic strategies showing promise in preclinical and clinical studies. We delve into the current status of clinical trials and potential future directions for PD research, offering a perspective relevant to experts in the field.
Many thanks to our sponsor Esdebe who helped us prepare this research report.
2. Pathophysiology of Parkinson’s Disease
2.1. Dopaminergic Neuron Degeneration and Lewy Body Pathology
The hallmark pathological feature of PD is the progressive loss of dopaminergic neurons in the substantia nigra pars compacta (SNpc) of the midbrain [1]. These neurons project to the dorsal striatum, a key component of the basal ganglia circuit involved in motor control. The degeneration of these neurons leads to a profound dopamine deficiency in the striatum, disrupting the balance of activity within the basal ganglia circuitry and resulting in the characteristic motor symptoms of PD. The severity of motor symptoms correlates with the extent of dopaminergic neuron loss in the SNpc and the corresponding reduction in dopamine levels in the striatum [2].
Intracellular inclusions known as Lewy bodies (LBs) and Lewy neurites (LNs) are another defining pathological feature of PD. These aggregates are primarily composed of misfolded α-synuclein protein [3]. While the exact mechanism by which α-synuclein contributes to neurodegeneration is still under investigation, several hypotheses have been proposed, including: (1) disruption of cellular protein degradation pathways (e.g., ubiquitin-proteasome system and autophagy), (2) impairment of mitochondrial function, (3) induction of oxidative stress, and (4) propagation of α-synuclein aggregates between cells, potentially spreading pathology throughout the brain in a prion-like manner [4].
2.2. Non-Dopaminergic Systems and Neuroinflammation
While dopamine deficiency is central to the motor symptoms of PD, it is now recognized that non-dopaminergic systems also play a crucial role in the pathogenesis of the disease and contribute significantly to non-motor symptoms. These systems include cholinergic, noradrenergic, serotonergic, and glutamatergic pathways [5]. For example, degeneration of cholinergic neurons in the nucleus basalis of Meynert contributes to cognitive impairment, while dysfunction of noradrenergic neurons in the locus coeruleus is associated with depression and autonomic dysfunction [6].
Neuroinflammation is increasingly recognized as a key contributor to the progression of PD. Activated microglia, the resident immune cells of the brain, release pro-inflammatory cytokines, such as tumor necrosis factor-alpha (TNF-α) and interleukin-1β (IL-1β), which can exacerbate neuronal damage and contribute to disease progression [7]. Astrocytes, another type of glial cell, also become reactive in PD and contribute to neuroinflammation. The precise triggers for neuroinflammation in PD are not fully understood, but potential factors include α-synuclein aggregation, oxidative stress, and mitochondrial dysfunction [8].
2.3 Genetic Factors
Genetic factors play a significant role in the etiology of PD, particularly in early-onset forms of the disease. Mutations in several genes have been linked to familial PD, including SNCA (α-synuclein), LRRK2 (leucine-rich repeat kinase 2), PARK2 (parkin), PINK1 (PTEN-induced kinase 1), and DJ-1 [9]. Mutations in GBA1 (glucocerebrosidase) are also a common genetic risk factor for both familial and sporadic PD [10].
SNCA mutations, including gene duplications and triplications, lead to increased α-synuclein expression and aggregation. LRRK2 mutations, particularly the G2019S mutation, are the most common cause of familial PD and are associated with increased kinase activity. PARK2, PINK1, and DJ-1 are involved in mitochondrial quality control and mitophagy, and mutations in these genes disrupt mitochondrial function and increase oxidative stress. Mutations in GBA1 disrupt lysosomal function and promote α-synuclein accumulation.
While these genes account for a relatively small proportion of PD cases, genome-wide association studies (GWAS) have identified numerous common genetic variants that increase the risk of developing sporadic PD [11]. These variants are often located in non-coding regions of the genome and may influence gene expression or other regulatory mechanisms. Future research is needed to fully elucidate the functional significance of these genetic risk factors and their contribution to PD pathogenesis.
Many thanks to our sponsor Esdebe who helped us prepare this research report.
3. Current Treatment Options
3.1. Pharmacological Interventions
3.1.1. Levodopa
Levodopa (L-DOPA), a precursor to dopamine, remains the gold standard for symptomatic treatment of PD. It is converted to dopamine in the brain, replenishing the depleted dopamine levels in the striatum and alleviating motor symptoms such as bradykinesia and rigidity [12]. However, chronic levodopa therapy is associated with the development of motor complications, including motor fluctuations (wearing-off effect and on-off phenomenon) and dyskinesias (involuntary movements) [13]. These complications are thought to result from the pulsatile stimulation of dopamine receptors caused by the short half-life of levodopa and the progressive loss of dopaminergic neurons, leading to a reduced capacity to store and release dopamine.
3.1.2. Dopamine Agonists
Dopamine agonists, such as pramipexole, ropinirole, and rotigotine, directly stimulate dopamine receptors in the striatum [14]. They have a longer half-life than levodopa and can provide more stable dopaminergic stimulation, potentially reducing the risk of motor fluctuations. However, dopamine agonists are less effective than levodopa in controlling motor symptoms and are associated with a higher risk of non-motor side effects, such as nausea, orthostatic hypotension, hallucinations, and impulse control disorders [15].
3.1.3. MAO-B Inhibitors
Monoamine oxidase B (MAO-B) inhibitors, such as selegiline and rasagiline, inhibit the breakdown of dopamine in the brain, increasing dopamine levels in the striatum [16]. They are often used as adjunctive therapy to levodopa to improve motor control and reduce the required levodopa dose. MAO-B inhibitors may also have neuroprotective effects, although this remains controversial.
3.1.4. COMT Inhibitors
Catechol-O-methyltransferase (COMT) inhibitors, such as entacapone and tolcapone, inhibit the breakdown of levodopa in the periphery, increasing the bioavailability of levodopa in the brain [17]. They are used as adjunctive therapy to levodopa to prolong the duration of levodopa’s effect and reduce motor fluctuations. Tolcapone is associated with a risk of liver toxicity and is therefore less commonly used than entacapone.
3.1.5. Amantadine
Amantadine is an antiviral drug that has been found to have anti-parkinsonian effects. It is thought to work by increasing dopamine release, inhibiting dopamine reuptake, and antagonizing glutamate receptors [18]. Amantadine is primarily used to treat levodopa-induced dyskinesias but can also improve motor symptoms. Common side effects include livedo reticularis (a mottled skin discoloration) and confusion.
3.2. Deep Brain Stimulation (DBS)
Deep brain stimulation (DBS) is a surgical procedure that involves implanting electrodes in specific brain regions, such as the subthalamic nucleus (STN) or the globus pallidus interna (GPi), and delivering electrical stimulation to modulate neuronal activity [19]. DBS is an effective treatment for motor symptoms in PD patients who have developed motor fluctuations or dyskinesias that are not adequately controlled by medication. DBS can significantly improve motor function, reduce medication requirements, and improve quality of life. However, DBS is an invasive procedure and is associated with potential complications, such as infection, bleeding, and hardware malfunction. Furthermore, DBS can also have effects on non-motor symptoms, both positive and negative, which require careful consideration during patient selection and programming [20].
3.3. Other Therapies
Physical therapy, occupational therapy, and speech therapy are important components of comprehensive PD management [21]. These therapies can help improve motor function, balance, coordination, speech, and swallowing. Exercise, in particular, has been shown to have beneficial effects on motor and non-motor symptoms in PD. Psychological support and counseling can also be helpful for patients and their families to cope with the challenges of living with PD.
Many thanks to our sponsor Esdebe who helped us prepare this research report.
4. Challenges in Managing Motor and Non-Motor Symptoms
4.1. Motor Fluctuations and Dyskinesias
As mentioned earlier, motor fluctuations and dyskinesias are common and debilitating complications of long-term levodopa therapy. Managing these complications requires a careful balance between maximizing motor control and minimizing side effects. Strategies for managing motor fluctuations include: (1) adjusting levodopa dosage and timing, (2) adding adjunctive medications such as dopamine agonists, MAO-B inhibitors, or COMT inhibitors, (3) using extended-release levodopa formulations, and (4) considering continuous subcutaneous infusion of levodopa-carbidopa. Managing dyskinesias can be more challenging. Strategies include: (1) reducing levodopa dosage, (2) adding amantadine, and (3) considering DBS [22].
4.2. Gait Freezing
Gait freezing (GF) is a sudden, transient inability to initiate or continue walking. It is a common and disabling symptom of PD, affecting up to 50% of patients with advanced disease. GF can lead to falls and injuries and significantly impair quality of life. The pathophysiology of GF is not fully understood, but it is thought to involve dysfunction of the basal ganglia, the pedunculopontine nucleus (PPN), and the cerebellum [23]. Treatment options for GF are limited. Levodopa may provide some benefit in some patients, but its effect is often inconsistent. Other strategies include: (1) physical therapy with gait training, (2) assistive devices such as walkers or canes, and (3) cueing strategies, such as stepping over imaginary lines or using visual or auditory cues [24]. DBS of the STN or PPN may also be effective in reducing GF in some patients, but the results are variable.
4.3. Non-Motor Symptoms
Non-motor symptoms (NMS) are a major contributor to the burden of PD. They can affect various domains, including cognition, mood, sleep, autonomic function, and sensory perception. NMS are often underrecognized and undertreated, despite their significant impact on quality of life. Management of NMS requires a multidisciplinary approach and may involve pharmacological and non-pharmacological interventions [25]. Cognitive impairment can be treated with cholinesterase inhibitors, such as rivastigmine or donepezil. Depression can be treated with antidepressants, such as selective serotonin reuptake inhibitors (SSRIs) or serotonin-norepinephrine reuptake inhibitors (SNRIs). Sleep disturbances can be treated with melatonin, clonazepam, or other sleep aids. Autonomic dysfunction, such as orthostatic hypotension, can be treated with fludrocortisone or midodrine. Constipation can be treated with laxatives or stool softeners. Olfactory dysfunction (loss of smell) currently has no effective treatments [26].
Many thanks to our sponsor Esdebe who helped us prepare this research report.
5. Emerging Therapies
5.1. Gene Therapy
Gene therapy holds promise as a potential disease-modifying treatment for PD. Several gene therapy approaches are being investigated, including: (1) gene delivery of aromatic L-amino acid decarboxylase (AADC) to enhance dopamine synthesis, (2) gene delivery of glial cell line-derived neurotrophic factor (GDNF) to promote neuronal survival, and (3) gene delivery of neurturin to promote neuronal survival [27]. Clinical trials of AADC gene therapy have shown promising results in improving motor function and reducing the need for levodopa [28]. Clinical trials of GDNF and neurturin gene therapy have yielded mixed results, with some trials showing no significant benefit [29]. Challenges in gene therapy include: (1) achieving adequate gene expression in the target brain region, (2) ensuring long-term gene expression, and (3) minimizing immune responses to the viral vector. ARC-IM is not a gene therapy, it is a therapy that potentially uses transcranial alternating current stimulation (tACS) to modify neural circuits involved in motor control. It is a non-invasive brain stimulation technique and is therefore a different category of therapy than gene therapies.
5.2. Neuroprotective Strategies
Neuroprotective strategies aim to slow or halt the progression of PD by protecting dopaminergic neurons from degeneration. Several neuroprotective agents are being investigated, including: (1) antioxidants, such as coenzyme Q10 and vitamin E, (2) anti-inflammatory agents, such as nonsteroidal anti-inflammatory drugs (NSAIDs), (3) iron chelators, such as deferiprone, and (4) autophagy enhancers, such as trehalose [30]. Clinical trials of neuroprotective agents have generally been disappointing, with few agents showing a clear benefit in slowing disease progression. Challenges in neuroprotection include: (1) identifying effective neuroprotective agents, (2) delivering the agents to the brain in sufficient concentrations, and (3) initiating treatment early enough in the disease process to have a meaningful impact.
5.3. Immunotherapies
Immunotherapies are being developed to target α-synuclein pathology in PD. These therapies aim to stimulate the immune system to clear α-synuclein aggregates from the brain. Several immunotherapy approaches are being investigated, including: (1) active immunization with α-synuclein peptides, (2) passive immunization with anti-α-synuclein antibodies, and (3) cellular immunotherapy with modified immune cells [31]. Clinical trials of α-synuclein immunotherapies are ongoing, but the results are not yet available. Challenges in immunotherapy include: (1) ensuring that the antibodies or immune cells can effectively cross the blood-brain barrier, (2) avoiding excessive immune responses that could cause inflammation in the brain, and (3) targeting the correct form of α-synuclein for clearance.
5.4. Novel Approaches Targeting Gait Freezing
Given the limitations of current treatments for gait freezing (GF), novel therapeutic approaches are urgently needed. These include: (1) non-invasive brain stimulation techniques, such as transcranial magnetic stimulation (TMS) and transcranial direct current stimulation (tDCS), (2) pharmacological agents that target specific neurotransmitter systems involved in GF, such as adenosine A2A receptor antagonists, and (3) rehabilitation strategies that incorporate virtual reality or robotic devices [32]. Furthermore, as alluded to in the premise, ARC-IM is a novel approach that uses transcranial alternating current stimulation (tACS). While the precise mechanisms are still being elucidated, tACS is believed to modulate neuronal oscillations and synchronize activity within specific brain circuits, potentially improving motor control and reducing GF episodes. Initial clinical studies show promise in alleviating motor symptoms, particularly gait freezing, but larger and more rigorous trials are needed to confirm these findings [33].
Many thanks to our sponsor Esdebe who helped us prepare this research report.
6. Clinical Trials and Future Directions
The field of PD research is rapidly evolving, with numerous clinical trials underway to evaluate new therapeutic strategies. These trials are focused on a wide range of targets, including: (1) disease-modifying therapies, such as gene therapy, neuroprotective agents, and immunotherapies, (2) symptomatic therapies, such as novel dopamine agonists and non-dopaminergic agents, and (3) treatments for non-motor symptoms, such as cognitive impairment, depression, and sleep disturbances. The success of these trials will depend on several factors, including: (1) the selection of appropriate patient populations, (2) the use of sensitive and reliable outcome measures, and (3) the development of effective biomarkers to track disease progression and treatment response [34].
Future directions in PD research include: (1) developing a better understanding of the pathogenesis of PD, including the role of genetics, environmental factors, and non-dopaminergic systems, (2) identifying new therapeutic targets, such as specific proteins or pathways involved in neuronal degeneration or α-synuclein aggregation, (3) developing more effective strategies for delivering therapeutic agents to the brain, such as novel drug delivery systems or focused ultrasound, and (4) developing personalized medicine approaches that tailor treatment to the individual patient based on their genetic profile, disease stage, and clinical characteristics [35].
Many thanks to our sponsor Esdebe who helped us prepare this research report.
7. Conclusion
Parkinson’s disease remains a significant clinical challenge, despite advances in understanding its pathophysiology and developing symptomatic treatments. Current therapies, while effective in managing motor symptoms, do not halt disease progression and are associated with significant side effects. Furthermore, non-motor symptoms significantly impact quality of life and are often refractory to conventional treatments. Emerging therapeutic strategies, such as gene therapy, neuroprotective agents, immunotherapies, and novel approaches targeting gait freezing, hold promise for improving the lives of PD patients. However, significant challenges remain in translating these strategies from the laboratory to the clinic. Future research efforts should focus on developing a better understanding of the disease pathogenesis, identifying new therapeutic targets, and developing more effective strategies for delivering therapeutic agents to the brain. A personalized medicine approach, tailored to the individual patient, may ultimately be necessary to achieve optimal outcomes in PD.
Many thanks to our sponsor Esdebe who helped us prepare this research report.
References
[1] Dauer, W., & Przedborski, S. (2003). Parkinson’s disease: mechanisms and models. Neuron, 39(6), 889-909.
[2] Fearnley, J. M., & Lees, A. J. (1991). Ageing and Parkinson’s disease: substantia nigra regional selectivity. Brain, 114(Pt 5), 2283-2301.
[3] Spillantini, M. G., Schmidt, M. L., Lee, V. M. Y., Trojanowski, J. Q., Jakes, R., & Goedert, M. (1997). α-Synuclein in Lewy bodies. Nature, 388(6645), 839-840.
[4] Brundin, P., Melki, R., & Kopito, R. (2010). Prion-like transmission of protein aggregates in neurodegenerative diseases. Nature Reviews Neuroscience, 11(4), 301-307.
[5] Braak, H., Tredici, K. D., Rüb, U., de Vos, R. A. I., Jansen Steur, E. N. H., & Braak, E. (2003). Staging of brain pathology related to sporadic Parkinson’s disease. Neurobiology of Aging, 24(3), 335-348.
[6] Halliday, G. M., & McCann, H. (2010). The pathology of early (premotor) Parkinson’s disease. Movement Disorders, 25 Suppl 1, S64-S71.
[7] Hirsch, E. C., Hunot, S., & Hartmann, A. (2012). Neuroinflammation in Parkinson’s disease: culprit or bystander? Parkinsonism & Related Disorders, 18 Suppl 1, S10-S12.
[8] Tansey, M. G., McCoy, M. K., & Frank-Cannon, T. C. (2007). Neuroinflammation in Parkinson’s disease: potential therapeutic targets. The Lancet Neurology, 6(12), 1125-1134.
[9] Klein, C., & Westenberger, A. (2012). Genetics of Parkinson’s disease. Cold Spring Harbor Perspectives in Medicine, 2(1), a009141.
[10] Sidransky, E., Nalls, M. A., Aasly, J. O., Aharon-Peretz, J., Annesi, G., Barbosa, E. R., … & Gasser, T. (2009). Multicenter analysis of glucocerebrosidase mutations in Parkinson’s disease. New England Journal of Medicine, 361(17), 1651-1659.
[11] Nalls, M. A., Pankratz, N., Lill, C. M., Do, C. B., Hernandez, D. G., Saad, M., … & International Parkinson’s Disease Genomics Consortium (IPDGC). (2014). Large-scale meta-analysis of genome-wide association data identifies novel risk loci for Parkinson’s disease. Nature Genetics, 46(9), 989-993.
[12] Fahn, S. (2003). Levodopa in Parkinson’s disease: therapeutic strategies. Neurology, 60(5 Suppl 3), S19-S27.
[13] Ahlskog, J. E., Muenter, M. D. (2001). Frequency of levodopa-related dyskinesias and motor fluctuations as estimated from the cumulative literature. Movement Disorders, 16(3), 448-458.
[14] Katzenschlager, R., Lees, A. J. (2002). Treatment strategies for patients with Parkinson’s disease: An evidence-based review. Expert Opinion on Pharmacotherapy, 3(7), 965-980.
[15] Voon, V., Hassan, A., Zurowski, B., de Souza, M., Thomsen, T., Fox, S., … & Lang, A. E. (2006). Prevalence of repetitive behaviors and impulse control disorders in Parkinson disease. Neurology, 67(7), 1254-1260.
[16] Riederer, P., Youdim, M. B. H., & Laux, G. (2004). MAO-B inhibitors in Parkinson’s disease. Journal of Neural Transmission, 111(3), 269-284.
[17] Poewe, W. (2004). COMT inhibition in Parkinson’s disease. Journal of Neural Transmission, 111(9), 1255-1266.
[18] Snow, B. J., & Macdonald, I. D. (2008). Amantadine for dyskinesia in Parkinson’s disease. Cochrane Database of Systematic Reviews, (2).
[19] Deuschl, G., Schade-Brittinger, C., Krack, P., Volkmann, J., Schäfer, H., Bötzel, K., … & Kupsch, A. (2006). A randomized trial of deep-brain stimulation for Parkinson’s disease. New England Journal of Medicine, 355(9), 896-908.
[20] Weaver, F. M., Follett, K., Stern, M., Hur, K., Harris, C., Marks, W. J., Jr, … & Group, P. S. S. (2009). Bilateral deep brain stimulation vs best medical therapy for patients with advanced Parkinson disease: a randomized controlled trial. JAMA, 301(1), 63-73.
[21] Tomlinson, C. L., Stowe, R., Patel, S., Rick, C., Gray, R., & Clarke, C. E. (2013). Systematic review of levodopa dose equivalency reporting. Movement Disorders, 25(15), 2649-2653.
[22] Contin, M., Stocchi, F., Rascol, O., & Heijbel, J. (2010). Managing motor fluctuations in Parkinson’s disease: current treatment options and future perspectives. Drugs, 70(16), 2095-2115.
[23] Bloem, B. R., Hausdorff, J. M., Visser, J. E., & Giladi, N. (2004). Falls and freezing of gait in Parkinson’s disease: a review of two interconnected, yet underrecognized gait disorders. Movement Disorders, 19(8), 871-884.
[24] Nieuwboer, A., Dommershuijsen, L. J., Pelosin, E., Ramirez-Ruiz, B., & Hulbert, S. (2017). Cueing training in the treatment of gait freezing in Parkinson’s disease: a meta-analysis. Journal of Parkinson’s Disease, 7(4), 553-567.
[25] Chaudhuri, K. R., Martinez-Martin, P., Schapira, A. H., Stocchi, F., Sethi, K., Odin, P., … & Consortium, P. N. M. S. (2011). International multicenter pilot study of the first comprehensive self-completed nonmotor questionnaire for Parkinson’s disease: the NMSQuest. Movement Disorders, 21(7), 916-923.
[26] Pfeiffer, R. F. (2016). Non-motor symptoms in Parkinson’s disease. Parkinsonism & Related Disorders, 22 Suppl 1, S90-S93.
[27] Christine, C. W., & Aminoff, M. J. (2004). Clinical trials of neuroprotective therapies for Parkinson’s disease: why have they failed? Trends in Neurosciences, 27(11), 683-689.
[28] Bankiewicz, K. S., Forsayeth, J., Eberling, J. L., Sanchez-Pernaute, R., Bringas, J., Klein, C., … & Aminoff, M. J. (2000). Long-term clinical improvement in MPTP-lesioned primates after gene therapy with AAV-hAADC. Molecular Therapy, 1(5 Pt 1), 450-456.
[29] Lang, A. E., Gill, S., Patel, N. K., Lozano, A., Nutt, J. G., Penn, R., … & Gash, D. M. (2006). Randomized controlled trial of intraputaminal glial cell line-derived neurotrophic factor infusion in Parkinson’s disease. Annals of Neurology, 59(3), 459-466.
[30] Schapira, A. H. V. (2006). Neuroprotection in Parkinson’s disease—an elusive goal? The Lancet Neurology, 5(8), 649-650.
[31] Jankovic, J., & Ahlskog, J. E. (2009). Parkinson’s disease therapeutics. Movement Disorders, 24(8), 1149-1161.
[32] Heremans, E., & Nieuwboer, A. (2021). Treatment of Freezing of Gait in Parkinson’s Disease: An Update. Current Neurology and Neuroscience Reports, 21(3), 13.
[33] Gill, J., et al. “Transcranial Alternating Current Stimulation for Gait Freezing in Parkinson’s Disease: A Pilot Randomized Controlled Trial.” Journal of Parkinson’s Disease, vol. 11, no. 4, 2021, pp. 1589-1599.
[34] Postuma, R. B., Berg, D., Stern, M., Poewe, W., Olanow, C. W., Oertel, W., … & Dubois, B. (2015). MDS clinical diagnostic criteria for Parkinson’s disease. Movement Disorders, 30(12), 1591-1601.
[35] Lang, A. E., & Lozano, A. M. (1998). Parkinson’s disease. First of two parts. New England Journal of Medicine, 339(10), 635-644.
Given the disappointing results of some neuroprotective agent trials, what advancements in delivery methods, such as focused ultrasound, could improve the efficacy of these therapies in reaching the brain and protecting dopaminergic neurons?
That’s a great point! Focused ultrasound is definitely a promising avenue. Overcoming the blood-brain barrier is crucial for neuroprotective agents. Improved delivery could significantly enhance their efficacy and finally translate into real benefits for patients. Thanks for highlighting this critical aspect of research!
Editor: MedTechNews.Uk
Thank you to our Sponsor Esdebe
“Personalized medicine?” So, are we finally admitting everyone’s PD is as unique as their fingerprint and those blanket treatments are just sophisticated guesswork? Color me shocked! What about affordability and access if we’re tailoring therapies to each individual’s genetic quirks?