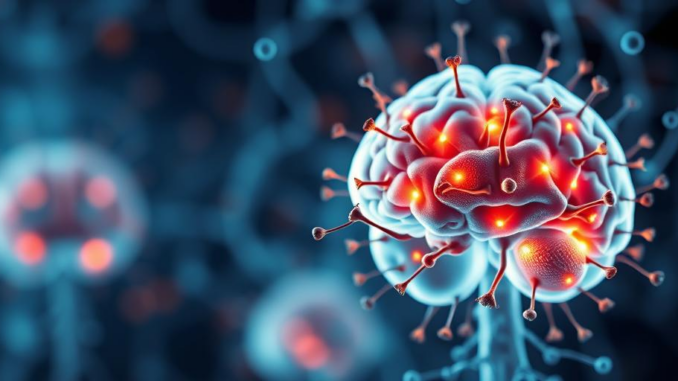
Abstract
Parkinson’s disease (PD) is classically defined as a neurodegenerative disorder primarily affecting dopaminergic neurons in the substantia nigra pars compacta, leading to motor deficits. However, a growing body of evidence highlights the systemic nature of PD, extending beyond the brain to involve the peripheral nervous system, cardiac system, gastrointestinal tract, and other organs. This review aims to provide a comprehensive overview of the systemic effects of PD, exploring its cardiac implications, diverse non-motor symptoms (NMS), and emerging therapeutic strategies targeting areas beyond the brain, including peripheral nerve clusters and the gut-brain axis. Furthermore, it critically evaluates the potential of early diagnostic markers based on peripheral biomarkers and discusses the challenges and opportunities in developing personalized treatment approaches that address the heterogeneous nature of PD pathology. We conclude by highlighting areas where further research is required to fully understand the complex interplay between central and peripheral manifestations of PD and to optimize therapeutic interventions.
Many thanks to our sponsor Esdebe who helped us prepare this research report.
1. Introduction
Parkinson’s disease (PD) is the second most common neurodegenerative disorder, affecting approximately 1% of the population over the age of 60 [1]. While the hallmark motor symptoms – tremor, rigidity, bradykinesia, and postural instability – define the clinical diagnosis, it is increasingly recognized that PD is a complex systemic disorder with a broad spectrum of non-motor symptoms (NMS) that often precede motor deficits by several years [2]. These NMS can significantly impact the quality of life of patients and their caregivers and include cognitive impairment, depression, anxiety, sleep disorders, autonomic dysfunction (e.g., constipation, orthostatic hypotension), and olfactory dysfunction [3].
The pathological hallmark of PD is the progressive loss of dopaminergic neurons in the substantia nigra pars compacta (SNpc), accompanied by the presence of intraneuronal aggregates of α-synuclein protein, known as Lewy bodies and Lewy neurites [4]. However, α-synuclein pathology is not limited to the brain and has been found in various peripheral tissues, including the gastrointestinal tract, heart, and salivary glands [5]. This widespread distribution of α-synuclein suggests a more extensive and systemic pathological process than previously appreciated. The Braak hypothesis proposes that PD pathology originates in the lower brainstem and olfactory bulb, spreading rostrally through interconnected brain regions [6]. However, recent research suggests that the pathogenesis may not always follow this ascending pattern and can be more complex and variable between individuals [7].
This review aims to provide an in-depth analysis of the systemic effects of PD, focusing on its cardiac implications, the diversity of NMS, and novel therapeutic strategies targeting extracerebral locations. By exploring the interplay between central and peripheral manifestations, we aim to provide a comprehensive understanding of the multifaceted nature of PD and identify potential avenues for improved diagnosis and treatment.
Many thanks to our sponsor Esdebe who helped us prepare this research report.
2. Cardiac Implications of Parkinson’s Disease
While PD is primarily considered a neurological disorder, accumulating evidence demonstrates significant cardiac involvement [8]. Cardiac dysfunction in PD patients can manifest as various abnormalities, including: orthostatic hypotension, cardiac arrhythmias, and subtle changes in cardiac structure and function. The mechanisms underlying these cardiac complications are multifactorial and involve both direct and indirect effects of PD pathology and related treatments. Firstly, autonomic dysfunction, a common NMS in PD, plays a critical role in mediating cardiac abnormalities [9]. Reduced sympathetic innervation of the heart, due to α-synuclein pathology in peripheral sympathetic ganglia, can lead to decreased heart rate variability, orthostatic hypotension, and an increased risk of cardiac arrhythmias.
Secondly, Lewy body pathology has been observed in the cardiac ganglia and myocardium of PD patients, suggesting a direct role of α-synuclein in cardiac dysfunction [10]. The presence of α-synuclein aggregates can disrupt normal cellular function, leading to impaired cardiomyocyte contractility and increased oxidative stress. Studies have shown that α-synuclein can directly interact with ion channels and calcium handling proteins in cardiomyocytes, affecting their electrophysiological properties and increasing the susceptibility to arrhythmias [11].
Thirdly, medications used to treat PD, particularly levodopa, can have adverse cardiac effects [12]. Levodopa can be converted to dopamine in the peripheral circulation, which can stimulate peripheral dopamine receptors, leading to vasodilation and hypotension. Furthermore, levodopa can exacerbate existing cardiac arrhythmias in susceptible individuals. However, the exact role of levodopa in causing cardiac complications in PD is complex, as some studies suggest that levodopa can also improve cardiac function by increasing cardiac output and reducing vascular resistance.
Advanced imaging techniques, such as myocardial perfusion scintigraphy and cardiac magnetic resonance imaging (MRI), have been used to assess cardiac function in PD patients. These studies have revealed subtle abnormalities in myocardial perfusion and contractility, even in the absence of overt cardiac symptoms [13]. These findings suggest that subclinical cardiac dysfunction may be more common in PD than previously recognized and highlights the importance of early detection and management of cardiac complications. Future research should focus on identifying specific biomarkers that can predict the risk of cardiac events in PD patients and on developing targeted therapies that can protect the heart from the damaging effects of α-synuclein pathology and autonomic dysfunction. Careful monitoring of blood pressure and heart rhythm is crucial for patients on Levodopa. Adjustments to medication regimens and lifestyle modifications may be required to mitigate cardiac risks. In cases of severe orthostatic hypotension, medications such as midodrine or fludrocortisone may be considered, after careful assessment of potential risks and benefits.
Many thanks to our sponsor Esdebe who helped us prepare this research report.
3. Non-Motor Symptoms: A Heterogeneous Landscape
Non-motor symptoms (NMS) are now recognized as integral to the PD disease process, often preceding motor deficits by several years and significantly impacting quality of life [14]. The spectrum of NMS in PD is remarkably broad, encompassing cognitive impairment, neuropsychiatric disturbances, sleep disorders, autonomic dysfunction, and sensory abnormalities [15]. Understanding the neurobiological basis of these diverse NMS is crucial for developing effective treatment strategies.
3.1. Cognitive Impairment
Cognitive impairment is a common and disabling NMS in PD, affecting up to 80% of patients in the later stages of the disease [16]. Cognitive deficits can range from mild executive dysfunction to dementia, significantly impacting daily functioning and independence. The underlying mechanisms of cognitive impairment in PD are complex and involve dysfunction in multiple brain regions, including the prefrontal cortex, parietal cortex, and hippocampus [17]. α-Synuclein pathology, cholinergic neuronal loss in the basal forebrain, and dopaminergic dysfunction all contribute to cognitive impairment. Furthermore, vascular risk factors, such as hypertension and diabetes, can exacerbate cognitive decline in PD patients.
3.2. Neuropsychiatric Disturbances
Neuropsychiatric disturbances, including depression, anxiety, apathy, and psychosis, are highly prevalent in PD [18]. Depression is one of the most common NMS, affecting up to 50% of PD patients. The etiology of depression in PD is multifactorial, involving both neurobiological factors (e.g., dopamine, serotonin, and norepinephrine deficiencies) and psychosocial factors (e.g., disability, social isolation). Anxiety disorders, including generalized anxiety disorder and panic disorder, are also common in PD, often co-occurring with depression. Apathy, characterized by a lack of motivation and interest, is another frequent neuropsychiatric symptom that can significantly impair daily functioning. Psychosis, including hallucinations and delusions, can occur in PD, particularly in advanced stages of the disease or as a side effect of dopaminergic medications [19].
3.3. Sleep Disorders
Sleep disorders are highly prevalent in PD and can significantly impact quality of life [20]. Common sleep disorders in PD include insomnia, restless legs syndrome (RLS), rapid eye movement (REM) sleep behavior disorder (RBD), and excessive daytime sleepiness. RBD, characterized by acting out dreams during REM sleep, is particularly relevant in PD as it is considered a strong predictor of future development of synucleinopathies, including PD and Lewy body dementia [21].
3.4. Autonomic Dysfunction
Autonomic dysfunction is a common and disabling NMS in PD, affecting multiple organ systems [22]. Common autonomic symptoms include constipation, orthostatic hypotension, urinary dysfunction (e.g., urinary frequency, urgency, and incontinence), and sexual dysfunction. Constipation is one of the earliest and most common NMS in PD, often preceding motor symptoms by several years. Orthostatic hypotension, characterized by a drop in blood pressure upon standing, can lead to dizziness, lightheadedness, and falls. Autonomic dysfunction is thought to be primarily caused by α-synuclein pathology in peripheral autonomic ganglia and the central autonomic network.
3.5. Sensory Abnormalities
Sensory abnormalities, including olfactory dysfunction, pain, and visual disturbances, are increasingly recognized as important NMS in PD [23]. Olfactory dysfunction, characterized by a reduced ability to detect and identify odors, is one of the earliest and most consistent NMS in PD. Pain is also common in PD, affecting up to 85% of patients. Pain can be nociceptive (e.g., musculoskeletal pain), neuropathic (e.g., radicular pain), or dystonic (e.g., pain associated with muscle spasms). Visual disturbances, including blurred vision, double vision, and difficulty with depth perception, can also occur in PD due to impaired eye movements and visual processing.
Effective management of NMS in PD requires a multidisciplinary approach, involving neurologists, psychiatrists, psychologists, and other healthcare professionals. Treatment strategies should be individualized based on the specific symptoms and needs of each patient. While some medications are available to treat specific NMS, such as antidepressants for depression and cholinesterase inhibitors for cognitive impairment, non-pharmacological interventions, such as exercise, cognitive rehabilitation, and behavioral therapy, can also be beneficial. Furthermore, addressing modifiable risk factors, such as hypertension and diabetes, can help to prevent or slow the progression of NMS in PD. The heterogeneous nature of NMS necessitates a personalized approach to assessment and management. Regular monitoring and adjustment of treatment strategies are essential to optimize outcomes and improve the quality of life of individuals living with PD.
Many thanks to our sponsor Esdebe who helped us prepare this research report.
4. Emerging Therapeutic Strategies: Beyond Dopamine Replacement
While dopamine replacement therapy with levodopa remains the cornerstone of symptomatic treatment for PD, it does not address the underlying neurodegenerative process and can lead to motor complications, such as dyskinesias, with long-term use [24]. Therefore, there is an urgent need to develop novel therapeutic strategies that target the underlying pathology of PD and provide disease-modifying effects. Emerging therapeutic approaches are focusing on several key areas, including:
4.1. Targeting α-Synuclein Aggregation
α-Synuclein aggregation is a central pathological feature of PD, and several therapeutic strategies are being developed to reduce α-synuclein levels or prevent its aggregation [25]. These strategies include:
- Antibody-based therapies: Monoclonal antibodies that bind to α-synuclein and promote its clearance are being developed and tested in clinical trials. These antibodies can be administered intravenously or subcutaneously and are designed to target both intracellular and extracellular α-synuclein aggregates.
- Small molecule inhibitors: Small molecules that inhibit α-synuclein aggregation are being developed to disrupt the process of α-synuclein fibril formation. These molecules can bind to α-synuclein monomers or oligomers and prevent them from forming larger aggregates.
- Gene therapy: Gene therapy approaches are being developed to reduce α-synuclein expression or promote its degradation. These approaches involve delivering genes encoding enzymes that degrade α-synuclein or silencing α-synuclein gene expression using RNA interference.
4.2. Neuroprotective Strategies
Neuroprotective strategies aim to protect dopaminergic neurons from degeneration and promote neuronal survival [26]. These strategies include:
- Glial cell line-derived neurotrophic factor (GDNF): GDNF is a neurotrophic factor that supports the survival and function of dopaminergic neurons. Clinical trials have investigated the efficacy of GDNF delivered directly into the brain, but results have been mixed. New delivery methods and GDNF variants are currently under investigation.
- Mitochondrial protection: Mitochondrial dysfunction is implicated in the pathogenesis of PD, and strategies to protect mitochondria are being developed. These strategies include using antioxidants to reduce oxidative stress and compounds that enhance mitochondrial function.
- Anti-inflammatory therapies: Inflammation is thought to contribute to neuronal damage in PD, and anti-inflammatory therapies are being investigated. These therapies include using non-steroidal anti-inflammatory drugs (NSAIDs) or other anti-inflammatory compounds.
4.3. Targeting the Gut-Brain Axis
The gut-brain axis is a bidirectional communication network between the gastrointestinal tract and the brain, and it is increasingly recognized as playing a role in the pathogenesis of PD [27]. Therapeutic strategies targeting the gut-brain axis include:
- Probiotics: Probiotics are live microorganisms that can alter the composition of the gut microbiota. Studies have shown that probiotics can improve gastrointestinal symptoms and potentially influence motor and non-motor symptoms in PD.
- Fecal microbiota transplantation (FMT): FMT involves transferring fecal material from a healthy donor to a recipient. Studies suggest FMT can modulate the gut microbiome and potentially alleviate some PD symptoms.
- Dietary interventions: Dietary interventions, such as the Mediterranean diet, can improve gut health and potentially influence PD symptoms. Furthermore, dietary strategies to manage constipation are crucial.
4.4. Targeting Peripheral Nerve Clusters
Emerging research suggests that peripheral nerve clusters, such as those in the enteric nervous system (ENS) and cardiac ganglia, could represent novel therapeutic targets in PD. α-Synuclein pathology in these peripheral nerve clusters may contribute to the development of non-motor symptoms like constipation and cardiac autonomic dysfunction [28]. Therapeutic strategies targeting these peripheral sites could include local delivery of anti-α-synuclein antibodies, neurotrophic factors, or gene therapies. Furthermore, vagus nerve stimulation (VNS), which has shown promise in treating other neurological disorders, could potentially modulate the activity of peripheral nerve clusters and alleviate PD symptoms [29]. However, the feasibility and efficacy of targeting peripheral nerve clusters in PD require further investigation. Specific delivery methods to peripheral nerves, such as ultrasound-guided injections, may be needed to achieve targeted therapeutic effects while minimizing systemic side effects.
4.5. Gene Therapy
Gene therapy holds immense promise for treating PD by directly addressing the underlying genetic and molecular causes of the disease. Several gene therapy approaches are currently under investigation, including:
- AADC Gene Therapy: AADC (aromatic L-amino acid decarboxylase) is an enzyme crucial for converting levodopa into dopamine. AADC gene therapy aims to deliver a functional copy of the AADC gene into the brain, increasing dopamine production and potentially reducing the need for oral levodopa. Clinical trials have shown promising results, with improvements in motor function and reduced dyskinesias [30].
- GAD Gene Therapy: GAD (glutamic acid decarboxylase) is an enzyme that synthesizes GABA, an inhibitory neurotransmitter. GAD gene therapy aims to increase GABA levels in the subthalamic nucleus (STN), a brain region that is overactive in PD. Clinical trials have shown that GAD gene therapy can reduce motor symptoms, particularly rigidity and bradykinesia [31].
- LRRK2 Gene Therapy: Mutations in the LRRK2 gene are a common cause of familial PD. LRRK2 gene therapy aims to reduce the expression of the mutant LRRK2 gene or correct the function of the mutant protein. This approach is still in early stages of development, but it holds promise for treating LRRK2-related PD [32].
4.6 Personalized Medicine Approaches
The heterogeneous nature of PD necessitates a personalized medicine approach to treatment. This involves tailoring treatment strategies to the specific genetic, clinical, and environmental factors of each patient [33]. Personalized medicine approaches may include:
- Genetic testing: Genetic testing can identify individuals with specific genetic mutations that increase their risk of developing PD. This information can be used to guide treatment decisions and identify individuals who may benefit from specific therapies.
- Biomarker profiling: Biomarker profiling can identify individuals with specific biological markers that predict their response to treatment. This information can be used to select the most appropriate treatment for each patient.
- Lifestyle modifications: Lifestyle modifications, such as exercise and diet, can improve symptoms and slow the progression of PD. The specific lifestyle modifications that are most beneficial may vary depending on the individual patient.
Many thanks to our sponsor Esdebe who helped us prepare this research report.
5. The Role of Peripheral Biomarkers in Early Diagnosis
Early and accurate diagnosis of PD is crucial for initiating timely interventions and potentially slowing disease progression. However, diagnosing PD in its early stages can be challenging, as motor symptoms may be subtle or absent. Therefore, there is a need for reliable biomarkers that can detect PD pathology before the onset of motor symptoms. Peripheral biomarkers, which can be easily obtained from blood, cerebrospinal fluid (CSF), or other bodily fluids, hold great promise for early PD diagnosis [34].
5.1. α-Synuclein
α-Synuclein is the most extensively studied biomarker in PD. Several assays have been developed to measure α-synuclein levels in peripheral fluids, including enzyme-linked immunosorbent assays (ELISAs) and real-time quaking-induced conversion (RT-QuIC) assays. RT-QuIC assays have shown high sensitivity and specificity for detecting misfolded α-synuclein aggregates in CSF and nasal swabs, suggesting that they could be useful for early PD diagnosis [35].
5.2. Neurofilament Light Chain (NfL)
Neurofilament light chain (NfL) is a structural protein found in neurons. Elevated levels of NfL in CSF and blood are indicative of neuronal damage and degeneration. Studies have shown that NfL levels are elevated in PD patients, particularly in those with more severe disease. NfL may be a useful biomarker for monitoring disease progression and assessing the efficacy of therapeutic interventions [36].
5.3. Glial Fibrillary Acidic Protein (GFAP)
Glial fibrillary acidic protein (GFAP) is a structural protein found in astrocytes. Elevated levels of GFAP in CSF and blood are indicative of astrocyte activation and inflammation. Studies have shown that GFAP levels are elevated in PD patients, suggesting that inflammation plays a role in the pathogenesis of the disease [37].
5.4. MicroRNAs (miRNAs)
MicroRNAs (miRNAs) are small non-coding RNA molecules that regulate gene expression. Studies have identified several miRNAs that are dysregulated in PD patients. These miRNAs may be involved in the pathogenesis of PD and could serve as potential biomarkers for early diagnosis [38].
5.5. Gut Microbiome
The gut microbiome is a complex community of microorganisms that reside in the gastrointestinal tract. Studies have shown that the gut microbiome is altered in PD patients. Specific changes in the gut microbiome composition may be associated with PD pathogenesis and could serve as potential biomarkers for early diagnosis [39]. The use of multiple biomarkers in combination, rather than relying on a single biomarker, may improve the accuracy of early PD diagnosis. Furthermore, longitudinal studies are needed to assess the predictive value of these biomarkers and to determine their ability to identify individuals who will develop PD in the future.
Many thanks to our sponsor Esdebe who helped us prepare this research report.
6. Conclusion
Parkinson’s disease is a complex and heterogeneous disorder with systemic effects extending beyond the brain. A comprehensive understanding of the cardiac implications, the diversity of non-motor symptoms, and the interplay between central and peripheral manifestations is crucial for developing effective diagnostic and therapeutic strategies. Emerging therapeutic approaches targeting areas beyond dopamine replacement, such as α-synuclein aggregation, neuroprotection, the gut-brain axis, and peripheral nerve clusters, hold promise for disease modification and symptom management. The identification and validation of peripheral biomarkers will enable earlier diagnosis and personalized treatment approaches. Future research should focus on elucidating the underlying mechanisms of systemic involvement in PD, developing targeted therapies that address both motor and non-motor symptoms, and implementing personalized medicine strategies that optimize outcomes for individuals living with this debilitating disease. Addressing the unmet needs of PD patients requires a collaborative effort between researchers, clinicians, and patients to accelerate the development of innovative therapies and improve the quality of life for those affected by this challenging disorder. Further studies are needed to fully understand the complex interplay between central and peripheral pathologies in PD. Longitudinal studies are essential to track disease progression and evaluate the effectiveness of new therapies. Ultimately, a multi-faceted approach, combining pharmacological interventions, lifestyle modifications, and personalized medicine strategies, will be necessary to provide optimal care for individuals with PD.
Many thanks to our sponsor Esdebe who helped us prepare this research report.
References
[1] de Lau, L. M., & Breteler, M. M. (2006). Epidemiology of Parkinson’s disease. The Lancet Neurology, 5(6), 525-535.
[2] Chaudhuri, K. R., Healy, D. G., & Schapira, A. H. (2006). Non-motor symptoms of Parkinson’s disease: diagnosis and management. The Lancet Neurology, 5(3), 235-245.
[3] Poewe, W., Seppi, K., Tanner, C. M., Halliday, G. M., Brundin, P., Volkmann, J., … & Lang, A. E. (2017). Parkinson disease. Nature Reviews Disease Primers, 3(1), 1-21.
[4] Spillantini, M. G., Schmidt, M. L., Lee, V. M. Y., Trojanowski, J. Q., Jakes, R., & Goedert, M. (1997). α-Synuclein in Lewy bodies. Nature, 388(6645), 839-840.
[5] Beach, T. G., Adler, C. H., Sue, L. I., Vedders, L., Lue, L., White III, C. L., … & Caviness, J. N. (2010). Multi-organ distribution of phosphorylated α-synuclein histopathology in subjects with Lewy body disorders. Acta Neuropathologica, 119(6), 689-702.
[6] Braak, H., Del Tredici, K., Rüb, U., de Vos, R. A. I., Jansen Steur, E. N. H., & Braak, E. (2003). Staging of brain pathology related to sporadic Parkinson’s disease. Movement Disorders, 18(4), 337-352.
[7] Hawkes, C. H., Del Tredici, K., & Braak, H. (2010). Parkinson’s disease: the dual-hit theory revisited. Annals of the New York Academy of Sciences, 1184(1), 151-161.
[8] Goldstein, D. S., Holmes, C., Li, S. T., Bruce, B., Metman, L. V., & Esler, M. (2011). Cardiac sympathetic denervation in Parkinson disease. Annals of Neurology, 69(6), 1017-1026.
[9] Orimo, S., Uzawa, A., Kano, O., Nakamura, A., Mori, F., Kakita, A., & Takahashi, H. (2007). Cardiac sympathetic denervation precedes motor dysfunction in Parkinson’s disease. Brain, 130(5), 1341-1351.
[10] Wakabayashi, K., Hayashi, S., Ishikawa, A., Tsuji, S., & Takahashi, H. (2003). Emerging concepts of Lewy body disease. Journal of Neurology, 250(Suppl 3), III1-III8.
[11] Dedkova, E. N., & Dudley Jr, S. C. (2011). α-Synuclein and cardiac dysfunction. Heart Failure Reviews, 16(5), 517-524.
[12] Merola, A., Romagnolo, A., Rizzi, L., Rosso, M., Artusi, C. A., Espay, A. J., & Lopiano, L. (2020). Cardiovascular complications of pharmacological treatment in Parkinson’s disease. Journal of Parkinson’s Disease, 10(3), 933-948.
[13] Yoshita, M. (2005). Cardiac 123I-MIBG scintigraphy in the differentiation of Parkinson’s disease from other parkinsonian syndromes. Journal of Neurology, Neurosurgery & Psychiatry, 76(5), 628-634.
[14] Pfeiffer, R. F. (2003). Nonmotor symptoms in Parkinson’s disease. Parkinsonism & Related Disorders, 9(Suppl 2), S73-S81.
[15] Jankovic, J. (2008). Parkinson’s disease: clinical features and diagnosis. Journal of Neurology, Neurosurgery & Psychiatry, 79(4), 368-376.
[16] Williams-Gray, C. H., Foltynie, T., Lewis, S. J. G., Barker, R. A., & Cambridge, C. D. (2007). Cognitive deficits and psychosis in Parkinson’s disease: a population-based study. Brain, 130(1), 75-88.
[17] Emre, M. (2003). Dementia associated with Parkinson’s disease. The Lancet Neurology, 2(5), 229-237.
[18] Aarsland, D., Bronnick, K., Alves, G., Tysnes, O. B., Pedersen, K. F., Ehrt, U., … & Larsen, J. P. (2009). The spectrum of neuropsychiatric disturbances in Parkinson’s disease. Parkinsonism & Related Disorders, 15(Suppl 3), S207-S214.
[19] Goetz, C. G., Leurgans, S., Pappert, E. J., Raman, R., Stemer, A., & Carvey, P. M. (2005). Prospective longitudinal assessment of hallucinations in Parkinson’s disease. Neurology, 65(11), 1730-1736.
[20] Chaudhuri, K. R., & Odin, P. (2003). Non motor symptoms of Parkinson’s disease. Parkinsonism & Related Disorders, 9(Suppl 2), S69-S72.
[21] Schenck, C. H., Bundlie, S. R., Mahowald, M. W. (1996). Delayed emergence of parkinsonism and/or dementia in 3% of older men initially diagnosed with idiopathic rapid-eye-movement sleep behavior disorder. Neurology, 46(2), 388-393.
[22] Mathias, C. J., & Bannister, R. (2003). Treatment of postural hypotension: clinical experience with midodrine and ephedrine. Clinical Autonomic Research, 13(Suppl 1), I48-I52.
[23] Doty, R. L. (2012). Olfactory dysfunction in Parkinson’s disease. Nature Reviews Neurology, 8(6), 329-339.
[24] Ahlskog, J. E. (2003). Slowing Parkinson’s disease progression: neuroprotection revisited. Movement Disorders, 18(6), 641-657.
[25] Olanow, C. W., Brundin, P., Watts, R. L., Lang, A. E., & Eskow Jaunarajs, K. L. (2019). α-Synuclein seeding and spreading in Parkinson’s disease: Therapeutic implications. Movement Disorders, 34(1), 1-12.
[26] Schapira, A. H. V. (2009). Neuroprotection and disease modification in Parkinson’s disease. Movement Disorders, 24(Suppl 2), S737-S741.
[27] Sampson, T. R., Debelius, J. W., Thron, T., Janssen, S., Shastri, G. G., Ilhan, Z. E., … & Mazmanian, S. K. (2016). Gut microbiota regulate motor deficits and neuroinflammation in a model of Parkinson’s disease. Cell, 167(6), 1469-1480. e1412.
[28] Lebouvier, T., Bruley des Varannes, S., Delamarre, E., Colin-Carenco, L., Legeron, R., Chauvelot, A., … & Neunlist, M. (2010). Intestinal biopsies show early α-synuclein deposits in Parkinson’s disease. Gastroenterology, 138(5), 1727-1733.
[29] Liu, S., Zhang, Y., & Wang, R. (2017). Vagus nerve stimulation improves motor function and reduces neuroinflammation in a rat model of Parkinson’s disease. European Journal of Neuroscience, 45(2), 189-197.
[30] Christine, C., Van Laar, T., Janson, C., Saint-Pierre, T. G., Maguire, R. P., Samulski, R. J., … & During, M. J. (2009). Safety and tolerability of putaminal AADC gene transfer for Parkinson disease. Neurology, 73(20), 1662-1669.
[31] Kaplitt, M. G., Feigin, A., Tang, C., Fitzsimons, H. L., Mattis, P., Lawlor, P. A., … & During, M. J. (2007). Safety and tolerability of gene therapy with an adeno-associated virus (AAV) vector expressing glutamic acid decarboxylase (GAD) for Parkinson’s disease: an open label, phase I trial. The Lancet, 369(9579), 2097-2105.
[32] Blandini, F., Rodriguez-Oroz, M. C., & Obeso, J. A. (2011). Gene therapy for Parkinson’s disease: recent advances and future perspectives. The Lancet Neurology, 10(1), 71-82.
[33] Lang, A. E. (2018). Personalized medicine for Parkinson’s disease: dream or reality?. The Lancet Neurology, 17(9), 747-748.
[34] Tolosa, E., Vila, M., Klein, C., & Rascol, O. (2021). LRRK2 in Parkinson disease: challenges of clinical trials. Nature Reviews Neurology, 17(6), 307-321.
[35] Siderowf, A., Concha-Marambio, L., Lafontant, D. E., Ffrench-Mullen, J., Urenia, J. M., Pan, S., … & Simuni, T. (2018). Assessment of heterogeneity in early Parkinson’s disease using α-synuclein seed amplification. Brain, 141(10), 3007-3019.
[36] Bridel, C., van Wijk, N., Mattsson, N., Zetterberg, H., Nellgard, B., Blennow, K., & Wild, E. J. (2019). Diagnostic value of cerebrospinal fluid neurofilaments in neurology: a systematic review and meta-analysis. JAMA Neurology, 76(9), 1035-1048.
[37] Mollenhauer, B., Cullen, V., Kahn, I., Krüger, R., & Beach, T. G. (2019). CSF Glial Fibrillary Acidic Protein (GFAP) is elevated in multiple system atrophy, but not in Parkinson’s disease or progressive supranuclear palsy. Parkinsonism & Related Disorders, 63, 160-162.
[38] Marques, O., Outeiro, T. F. (2015). The role of microRNAs in Parkinson’s disease. Journal of Parkinson’s Disease, 5(3), 453-466.
[39] Hill-Burns, E. M., Debelius, J. W., Dadayan, K. N., Darrow, J. J., Lynch, S. V., & Gatto, N. M. (2017). Parkinson’s disease and the gastrointestinal microbiome. Movement Disorders, 32(5), 668-676.
Be the first to comment