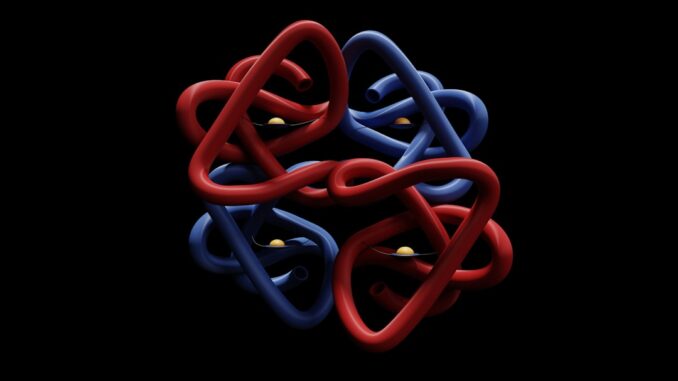
Peptides: Multifaceted Roles in Immunomodulation and Therapeutic Development
Abstract
Peptides, short chains of amino acids, represent a versatile class of biomolecules with diverse biological functions. Their roles extend far beyond simple protein building blocks, encompassing crucial functions in cell signaling, immune regulation, and host defense. This report provides a comprehensive overview of peptide structure, function, and synthesis, with a specific focus on their immunomodulatory capabilities and therapeutic potential, particularly in autoimmune diseases such as type 1 diabetes (T1D). We explore different classes of peptides, their interactions with the immune system at both innate and adaptive levels, and the latest advancements in peptide-based therapeutic strategies, including vaccine development, immunomodulatory therapies, and targeted drug delivery. Furthermore, we discuss the challenges and future directions in the field, emphasizing the need for improved peptide design, delivery systems, and clinical trial strategies to fully realize the therapeutic promise of peptides.
1. Introduction
Peptides, defined as short chains of amino acids typically ranging from 2 to 50 residues, constitute a fundamental class of biological molecules. While historically viewed primarily as intermediates in protein synthesis and degradation, peptides have emerged as critical players in a wide array of cellular processes. They function as hormones, neurotransmitters, antimicrobial agents, and immunomodulators, impacting virtually every aspect of physiology. The inherent versatility of peptides stems from their diverse structural properties and the ability to interact with a multitude of biological targets, including receptors, enzymes, and antibodies.
This report will focus on the immunomodulatory roles of peptides and their therapeutic potential in autoimmune diseases, using type 1 diabetes as a specific example. Autoimmune diseases are characterized by an aberrant immune response directed against self-antigens, leading to chronic inflammation and tissue damage. The precise mechanisms underlying autoimmunity remain complex and multifactorial, involving genetic predisposition, environmental triggers, and dysregulation of immune tolerance. Peptide-based therapies offer a promising approach to restore immune homeostasis and prevent or treat autoimmune diseases by selectively modulating immune responses without causing broad immunosuppression.
2. Peptide Structure and Synthesis
2.1 Peptide Structure
The sequence of amino acids dictates a peptide’s primary structure, and this sequence determines the peptide’s ultimate function. Each amino acid is linked to the next via a peptide bond, formed between the carboxyl group of one amino acid and the amino group of the adjacent amino acid. The resulting peptide chain possesses a defined N-terminus (amine group of the first amino acid) and a C-terminus (carboxyl group of the last amino acid). The sequence, length, and composition of amino acids dictate the specific properties of each peptide, and its structure, including hydrophobicity, charge, and ability to form secondary and tertiary structures.
Peptides can fold into diverse secondary structures such as alpha-helices, beta-sheets, and turns, which are stabilized by hydrogen bonds between the peptide backbone atoms. These secondary structures, in turn, influence the overall three-dimensional conformation of the peptide, crucial for its biological activity. The tertiary structure is stabilized by interactions between the amino acid side chains (R-groups), including hydrophobic interactions, electrostatic interactions, disulfide bonds, and hydrogen bonds. Understanding the structural properties of peptides is essential for designing effective therapeutic agents, as structure dictates the affinity and specificity of peptide binding to its target.
2.2 Peptide Synthesis
Peptides can be produced through several different methods, including:
-
Solid-Phase Peptide Synthesis (SPPS): This is the most common method for synthesizing peptides in the laboratory. SPPS involves the stepwise addition of amino acids to a resin-bound growing peptide chain. This process allows for the efficient synthesis of peptides with defined sequences and modifications. The method has been significantly advanced and automated, and allows for the inclusion of non-natural amino acids into the peptide chain.
-
Liquid-Phase Peptide Synthesis (LPPS): LPPS is a traditional method that involves synthesizing peptides in solution. While LPPS can be used for the synthesis of small peptides, it is less efficient for longer peptides due to difficulties in purification and yield reduction at each step.
-
Recombinant DNA Technology: Peptides can be produced in living organisms using recombinant DNA technology. This method involves cloning the gene encoding the desired peptide into a host organism, such as bacteria or yeast, and then inducing the organism to produce the peptide. This approach is particularly useful for producing large quantities of peptides, though may not provide the flexibility for post-translational modifications required for optimal function.
-
Enzymatic Synthesis: Enzymes, such as proteases, can be used to catalyze the formation of peptide bonds. Enzymatic synthesis offers a more environmentally friendly alternative to chemical synthesis methods, but it may be limited by substrate specificity and reversibility.
Each method offers specific advantages and disadvantages with respect to cost, scalability, and the ability to incorporate non-natural amino acids or post-translational modifications. The choice of synthesis method depends on the specific requirements of the peptide, including its length, sequence, and desired modifications.
3. Peptides and the Immune System
3.1 Peptides as Immunogens
Peptides play a critical role in shaping the immune response as antigens recognized by T cells and B cells.
-
T cell epitopes: T cells recognize peptides presented by major histocompatibility complex (MHC) molecules on the surface of antigen-presenting cells (APCs). MHC class I molecules present peptides derived from intracellular proteins to cytotoxic T lymphocytes (CTLs), while MHC class II molecules present peptides derived from extracellular proteins to helper T cells (Th cells). The interaction between the T cell receptor (TCR) and the MHC-peptide complex triggers T cell activation and downstream immune responses. Identifying immunogenic peptides, particularly those derived from pathogens or tumor-associated antigens, is crucial for developing effective vaccines and immunotherapies.
-
B cell epitopes: B cells recognize peptides and other antigens through their B cell receptor (BCR), a membrane-bound antibody. The interaction between the BCR and the antigen triggers B cell activation, proliferation, and differentiation into antibody-secreting plasma cells. Peptides can act as B cell epitopes, eliciting the production of antibodies that can neutralize pathogens, opsonize cells for phagocytosis, or activate the complement system. B cell epitopes can be linear or conformational, depending on whether they are formed by contiguous amino acid sequences or by discontinuous sequences brought together by protein folding.
3.2 Immunomodulatory Peptides
Beyond their role as antigens, peptides can also directly modulate immune cell function and cytokine production. Several classes of immunomodulatory peptides have been identified, including:
-
Cytokine-derived peptides: Certain peptides derived from cytokines themselves can modulate cytokine receptor signaling. For instance, peptides derived from IL-10 can mimic the immunosuppressive activity of IL-10, while peptides derived from IL-2 can promote T cell proliferation and activation. These peptides offer a targeted approach to modulate cytokine signaling without the potential side effects associated with systemic cytokine administration.
-
Chemokine-derived peptides: Chemokines are chemoattractant cytokines that regulate the migration of immune cells. Peptides derived from chemokines can act as agonists or antagonists of chemokine receptors, modulating immune cell trafficking and inflammation. For example, peptides derived from CCL5 (RANTES) can block the interaction of CCL5 with its receptor CCR5, inhibiting the recruitment of T cells and macrophages to inflammatory sites.
-
Antimicrobial peptides (AMPs): AMPs are a diverse group of peptides produced by a wide range of organisms, including bacteria, fungi, plants, and animals. AMPs possess broad-spectrum antimicrobial activity against bacteria, fungi, viruses, and parasites. In addition to their direct antimicrobial activity, AMPs can also modulate immune cell function, promote wound healing, and regulate inflammation. Some AMPs can act as chemoattractants for immune cells, promoting their recruitment to sites of infection or inflammation.
-
Tregitopes: Tregitopes are naturally processed and presented peptides derived from immunoglobulin G (IgG) that can induce regulatory T cells (Tregs). Tregs are a subset of T cells that suppress immune responses and maintain immune tolerance. Tregitopes can promote immune tolerance in autoimmune diseases and prevent transplant rejection. In silico screening approaches have aided the development of artificial versions with enhanced Treg activation properties.
-
Peptides Targeting Immune Checkpoints: Immune checkpoints, such as PD-1 and CTLA-4, are inhibitory receptors that regulate T cell activation and prevent excessive immune responses. Peptides targeting immune checkpoints can block the interaction of these receptors with their ligands, enhancing T cell activation and promoting anti-tumor immunity.
3.3 Peptides and Autoimmunity
In the context of autoimmune diseases, peptides can play both pathogenic and therapeutic roles. On one hand, autoreactive T cells and B cells recognize self-peptides presented by MHC molecules or displayed on cell surfaces, initiating and perpetuating autoimmune responses. On the other hand, peptides can be used to induce immune tolerance, suppress inflammation, and protect target tissues from autoimmune damage. This duality highlights the importance of carefully selecting and designing peptides for therapeutic applications in autoimmune diseases.
4. Peptide-Based Therapies for Autoimmune Diseases
Peptide-based therapies offer a promising approach to treat autoimmune diseases by selectively modulating immune responses without causing broad immunosuppression. Several peptide-based therapeutic strategies are currently under development or in clinical trials for autoimmune diseases, including type 1 diabetes.
4.1 Peptide Vaccines
Peptide vaccines aim to induce antigen-specific immune tolerance or immune activation by delivering specific peptides derived from autoantigens or pathogens. Peptide vaccines can be designed to:
-
Induce T cell tolerance: Peptide vaccines can induce tolerance by promoting the deletion or anergy of autoreactive T cells. This can be achieved by administering peptides in a tolerogenic context, such as in the absence of costimulatory signals or with the co-administration of immunosuppressive agents. For example, in type 1 diabetes, peptide vaccines based on islet autoantigens, such as insulin or glutamic acid decarboxylase (GAD), have been tested to induce tolerance to islet beta cells and prevent disease progression.
-
Activate protective T cell responses: Peptide vaccines can also be used to activate protective T cell responses against pathogens or tumor-associated antigens. This can be achieved by delivering peptides in an immunogenic context, such as with the co-administration of adjuvants that stimulate the innate immune system. For example, peptide vaccines based on viral antigens have been used to prevent or treat viral infections, while peptide vaccines based on tumor-associated antigens have been used to elicit anti-tumor immunity.
4.2 Immunomodulatory Peptides as Therapeutics
As discussed in section 3.2, peptides derived from cytokines, chemokines, antimicrobial proteins or immunoglobulins can directly modulate immune cell function and cytokine production, offering a targeted approach to treat autoimmune diseases.
- Type 1 Diabetes: Hybrid Insulin Peptides (HIPs) are modified insulin peptides that are generated during antigen processing and presentation in the thymus. HIPs have shown to be potent autoantigens in T1D and may play a role in the pathogenesis of the disease. These peptides have become therapeutic targets for T1D.
4.3 Targeted Drug Delivery
Peptides can be used as targeting ligands to deliver drugs or other therapeutic agents specifically to immune cells or target tissues. This approach can improve the efficacy and reduce the toxicity of therapeutic agents by concentrating them at the site of action. Peptides can be conjugated to drugs, nanoparticles, or liposomes, allowing for targeted delivery to specific cell types or tissues. For example, peptides that bind to specific receptors on immune cells can be used to deliver immunosuppressive drugs or anti-inflammatory agents to these cells, suppressing their activity and reducing inflammation.
5. Challenges and Future Directions
Despite the promising therapeutic potential of peptides, several challenges remain in the development of effective peptide-based therapies. These challenges include:
-
Peptide Stability and Delivery: Peptides are susceptible to degradation by proteases in vivo, limiting their bioavailability and efficacy. To overcome this challenge, several strategies are being developed to improve peptide stability, including: (1) incorporating non-natural amino acids into the peptide sequence; (2) cyclizing the peptide; (3) conjugating the peptide to polyethylene glycol (PEG) or other polymers; and (4) encapsulating the peptide in nanoparticles or liposomes. In addition, novel delivery systems, such as microneedle patches and oral delivery formulations, are being developed to improve peptide absorption and bioavailability.
-
Peptide Immunogenicity: Peptides can elicit unwanted immune responses, such as the production of anti-peptide antibodies or the activation of T cells. To minimize peptide immunogenicity, several strategies are being developed, including: (1) selecting peptides that are naturally processed and presented by MHC molecules; (2) modifying the peptide sequence to reduce its binding affinity to MHC molecules; and (3) co-administering the peptide with immunosuppressive agents.
-
Clinical Trial Design: Clinical trials of peptide-based therapies for autoimmune diseases have often yielded mixed results. This may be due to several factors, including: (1) heterogeneity in patient populations; (2) lack of validated biomarkers to monitor treatment response; and (3) inadequate dose selection. To improve the success of clinical trials, it is essential to carefully select patient populations, identify relevant biomarkers, and optimize dosing regimens.
Future research should focus on addressing these challenges and developing more effective peptide-based therapies for autoimmune diseases. Specific areas of focus should include:
-
Rational Peptide Design: Developing computational tools and high-throughput screening methods to identify and optimize peptide sequences for desired therapeutic effects. This includes designing peptides with improved stability, reduced immunogenicity, and enhanced binding affinity to target molecules.
-
Personalized Peptide Therapy: Tailoring peptide-based therapies to individual patients based on their genetic background, immune profile, and disease stage. This requires the development of biomarkers that can predict treatment response and identify patients who are most likely to benefit from peptide therapy.
-
Combination Therapies: Combining peptide-based therapies with other immunomodulatory agents or conventional therapies to achieve synergistic effects. This may involve combining peptide vaccines with checkpoint inhibitors or combining immunomodulatory peptides with anti-inflammatory drugs.
-
Advanced Delivery Systems: Exploring novel delivery systems to improve peptide bioavailability and target delivery to specific immune cells or tissues. This includes developing nanoparticles, liposomes, and cell-penetrating peptides that can effectively deliver peptides to their site of action.
6. Conclusion
Peptides represent a promising class of therapeutic agents for autoimmune diseases. Their ability to selectively modulate immune responses and target specific cells or tissues offers a significant advantage over conventional immunosuppressive therapies. While challenges remain in the development of effective peptide-based therapies, ongoing research efforts are focused on addressing these challenges and developing more potent and targeted peptide-based strategies. With continued advancements in peptide design, delivery systems, and clinical trial strategies, peptides have the potential to revolutionize the treatment of autoimmune diseases and improve the lives of millions of patients.
References
- Schwartz, R. H. T cell clonal anergy. Current Opinion in Immunology 1997, 9, 351-357.
- Stromnes, I. M.; Greenberg, P. D. Principles of adoptive T cell therapy for cancer. Immunol Rev 2014, 257, 8-26.
- Doyle, A.; Wickham, S.; Cooper, D.; Ponsonby, A. L.; Morahan, G.; Ellis, J. A. Hybrid insulin peptides are promising targets for immunotherapy of type 1 diabetes. Cell Immunol 2015, 294, 35-42.
- Bach, J. F. The effect of infections on susceptibility to autoimmune and allergic diseases. N Engl J Med 2002, 347, 911-920.
- Vincenti, F.; Bluestone, J. A.; Bourcier, T.; Durham, M. M.; Genberg, H.; Giannoukakis, N.; Gitelman, S. E.; Hafler, J. P.; Keymeulen, B.; Kolb, H. et al. Trial of Anti-CD3 Antibody, Teplizumab, in Patients at Risk for Type 1 Diabetes. N Engl J Med 2019, 381, 1628-1639.
- Ferraro, B.; Ghasemi, R.; Dhanik, A.; Amin, M. A.; Bobbala, D.; Bartolucci, A. A.; Jiao, X.; Lee, R. J.; Torchilin, V. P. Peptide-based targeted delivery. Adv Drug Deliv Rev 2020, 157, 276-307.
- Makovets, S.; Jansson, L. Strategies for improving peptide stability and delivery. Peptides 2019, 111, 3-16.
- Weber, A.; Zwick, M.; Eisenhardt, S. U.; Weber, C. Regulatory T Cell-Inducing Epitopes from IgG Constant Regions. Frontiers in Immunology 2018, 9, 2155.
- Vignali, D. A. A.; Collison, L. W.; Workman, C. J. How regulatory T cells work. Nature Reviews Immunology 2008, 8, 523-532.
- Elias, D.; Cohen, I.R. Peptide therapy for autoimmune disease. The Lancet 1994, 343, 705-706.
- Melnikov, A. A.; Grebinyk, S. V.; Kost, N. V.; Vukojevic, V.; Tkachenko, S. E.; Gerasimova, T. N.; Miroshnikov, A. I.; Kozhich, A. T. Immunomodulatory peptides: Structure, function, and clinical application. Biochemistry (Moscow) 2018, 83, 1-18.
- Bahgat, M.; Hagar, E.S.; Allam, N.G.; Ali, A.M. Antimicrobial peptides: the story so far. Arch Microbiol 2023, 205, 131.
- Khoury, S.J.; Gurewich, M.; Weiner, H.L. Oral tolerance to myelin basic protein in multiple sclerosis. Ann N Y Acad Sci 1993, 685, 343-351.
The discussion of peptide synthesis is particularly interesting. The advancements in SPPS, especially the ability to incorporate non-natural amino acids, open exciting possibilities for designing peptides with enhanced therapeutic properties and stability.
Thanks for highlighting the importance of SPPS! The ability to incorporate non-natural amino acids truly expands the possibilities for peptide design. Imagine the potential for creating novel therapeutics with increased resistance to degradation and enhanced target specificity. It’s an exciting frontier for immunomodulation!
Editor: MedTechNews.Uk
Thank you to our Sponsor Esdebe
The discussion of targeted drug delivery using peptides is compelling. This approach holds significant promise for enhancing therapeutic efficacy while minimizing off-target effects. Exploring novel peptide-drug conjugates and delivery vehicles could further improve treatment outcomes, particularly in complex autoimmune conditions.