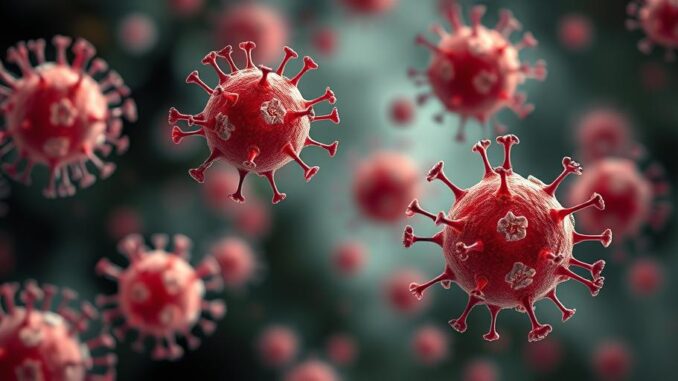
Abstract
Phagocytes, including macrophages, neutrophils, dendritic cells, and microglia, are essential components of the innate immune system, playing critical roles in tissue homeostasis, pathogen clearance, and adaptive immune responses. These cells are highly versatile, exhibiting diverse phenotypes and functional capabilities depending on their tissue environment and activation signals. This research report explores the multifaceted roles of phagocytes, highlighting their contributions to both normal physiological processes and disease pathogenesis. We delve into the origins and differentiation of various phagocyte populations, their mechanisms of action in tissue remodeling and immune surveillance, the intricate signaling pathways that regulate their activity, and their involvement in chronic inflammatory diseases, including but not limited to neurodegenerative disorders, autoimmune diseases, and metabolic syndrome. We also discuss emerging therapeutic strategies targeting phagocyte function to modulate inflammatory responses and promote tissue repair. Furthermore, we emphasize the need for a nuanced understanding of phagocyte heterogeneity and plasticity to develop targeted therapies that harness their beneficial functions while mitigating their detrimental effects in disease.
Many thanks to our sponsor Esdebe who helped us prepare this research report.
1. Introduction
Phagocytes, derived from myeloid lineage progenitors, are a heterogeneous group of cells characterized by their ability to engulf and degrade particulate matter, pathogens, and cellular debris. This process, known as phagocytosis, is fundamental to host defense and tissue homeostasis. Beyond their role as “cellular garbage collectors,” phagocytes are crucial mediators of inflammation, orchestrating both pro-inflammatory and anti-inflammatory responses to maintain tissue integrity and promote healing. The functional plasticity of phagocytes allows them to adapt to diverse microenvironments and perform a wide range of tasks, from clearing apoptotic cells to presenting antigens to T cells. This dynamic nature also makes them key players in the pathogenesis of numerous diseases, where their aberrant activation or dysfunction can contribute to chronic inflammation and tissue damage.
The classical view of phagocytes as simply scavengers of pathogens and cellular debris has evolved significantly in recent years. We now recognize their complex roles in regulating the immune response, influencing tissue remodeling, and contributing to the development and progression of chronic diseases. Understanding the intricacies of phagocyte biology, including their origins, differentiation pathways, activation mechanisms, and functional heterogeneity, is crucial for developing effective therapeutic strategies that can harness their beneficial functions while mitigating their detrimental effects.
Many thanks to our sponsor Esdebe who helped us prepare this research report.
2. Classification and Origin of Phagocytes
Phagocytes comprise a diverse group of cells that can be broadly classified into several main categories, including macrophages, neutrophils, dendritic cells (DCs), and eosinophils. While all these cells share the ability to perform phagocytosis, they differ significantly in their origin, tissue distribution, lifespan, and functional specialization.
2.1 Macrophages
Macrophages are arguably the most versatile and widely distributed phagocytes in the body. They are derived from monocytes, which originate in the bone marrow and circulate in the blood before migrating into tissues, where they differentiate into resident macrophages. Tissue-resident macrophages are strategically positioned throughout the body, including the liver (Kupffer cells), lungs (alveolar macrophages), brain (microglia), spleen (red pulp macrophages), and bone marrow (osteoclasts). They play a critical role in maintaining tissue homeostasis by clearing apoptotic cells, cellular debris, and pathogens, and by producing cytokines and growth factors that regulate tissue remodeling and repair.
Macrophages exhibit remarkable plasticity, adopting diverse phenotypes and functional profiles in response to environmental cues. The classical M1/M2 paradigm, while an oversimplification, provides a useful framework for understanding macrophage polarization. M1 macrophages, activated by pro-inflammatory stimuli such as LPS and IFN-γ, produce high levels of pro-inflammatory cytokines like TNF-α, IL-1β, and IL-12, promoting Th1-mediated immune responses and mediating anti-tumor immunity. In contrast, M2 macrophages, activated by IL-4, IL-10, and TGF-β, produce anti-inflammatory cytokines like IL-10 and TGF-β, promoting tissue repair, angiogenesis, and immunosuppression. This paradigm is increasingly seen as a spectrum of functional states, with macrophages exhibiting a range of phenotypes depending on the specific signals they receive.
The ontogeny of tissue-resident macrophages is complex and varies depending on the tissue. Some macrophages, like microglia in the brain, are derived from yolk sac progenitors during embryonic development and self-renew throughout life, largely independent of circulating monocytes. Other macrophage populations are continuously replenished by monocytes recruited from the blood. The relative contribution of these two pathways to the maintenance of tissue-resident macrophage populations is an area of ongoing research.
2.2 Neutrophils
Neutrophils are the most abundant leukocytes in the blood and are the first responders to infection and tissue injury. They are produced in large numbers in the bone marrow and rapidly migrate to sites of inflammation, where they engulf and kill bacteria, fungi, and other pathogens. Neutrophils are short-lived cells that undergo apoptosis shortly after arriving at the site of inflammation. However, their release of proteases and reactive oxygen species (ROS) can contribute to tissue damage if neutrophil activation is not tightly regulated. Neutrophils can also form neutrophil extracellular traps (NETs), which are web-like structures composed of DNA, histones, and antimicrobial proteins that trap and kill pathogens. However, excessive NET formation can contribute to inflammation and thrombosis.
2.3 Dendritic Cells
Dendritic cells (DCs) are specialized antigen-presenting cells that play a critical role in initiating adaptive immune responses. They are strategically located in tissues throughout the body, where they capture antigens and migrate to lymph nodes, where they present these antigens to T cells. DCs are highly heterogeneous, comprising several subsets with distinct functions and tissue distribution. Conventional DCs (cDCs) are the most important for initiating T cell responses, while plasmacytoid DCs (pDCs) are specialized in producing type I interferons in response to viral infection. DCs can also phagocytose pathogens and cellular debris, but their primary function is to process and present antigens to T cells.
2.4 Other Phagocytes
Other phagocytes, such as eosinophils and basophils, play specialized roles in immune responses to parasites and allergic reactions. Eosinophils release cytotoxic granules containing enzymes and proteins that kill parasites and contribute to tissue damage in allergic diseases. Basophils release histamine and other mediators that contribute to inflammation and allergic reactions. While their primary function is not phagocytosis, they can engulf particulate matter and contribute to inflammation in certain contexts.
Many thanks to our sponsor Esdebe who helped us prepare this research report.
3. Mechanisms of Phagocytosis and Intracellular Killing
Phagocytosis is a multi-step process that involves the recognition and binding of targets, engulfment, and intracellular degradation. The process is initiated by the binding of phagocyte receptors to ligands on the surface of target cells or particles. These receptors can be classified into several categories, including Fc receptors, complement receptors, scavenger receptors, and pattern recognition receptors (PRRs).
3.1 Receptor-Mediated Recognition and Binding
Fc receptors bind to the Fc region of antibodies, allowing phagocytes to engulf antibody-opsonized pathogens and cells. Complement receptors bind to complement fragments, such as C3b and iC3b, which are deposited on the surface of pathogens and cells during complement activation. Scavenger receptors bind to a variety of ligands, including modified lipoproteins, apoptotic cells, and bacterial cell wall components. PRRs, such as Toll-like receptors (TLRs) and C-type lectin receptors (CLRs), recognize pathogen-associated molecular patterns (PAMPs) and damage-associated molecular patterns (DAMPs), triggering phagocytosis and activating downstream signaling pathways that lead to the production of inflammatory cytokines.
3.2 Engulfment and Phagosome Formation
Following receptor binding, the phagocyte membrane extends around the target, forming a phagocytic cup. The actin cytoskeleton plays a critical role in driving membrane extension and engulfment. Once the target is completely engulfed, the phagocytic cup fuses to form a phagosome, a membrane-bound vesicle containing the ingested material. The phagosome then undergoes a series of maturation steps, fusing with endosomes and lysosomes to form a phagolysosome.
3.3 Intracellular Killing and Degradation
The phagolysosome contains a variety of antimicrobial enzymes and proteins, including proteases, lipases, nucleases, and reactive oxygen species (ROS), which degrade the ingested material. ROS are produced by NADPH oxidase, a multi-subunit enzyme complex that generates superoxide radicals. Other antimicrobial mechanisms include the production of nitric oxide (NO) by inducible nitric oxide synthase (iNOS) and the sequestration of iron and other essential nutrients.
Some pathogens have evolved mechanisms to evade phagocytosis or to survive within phagocytes. For example, Mycobacterium tuberculosis inhibits phagosome maturation, preventing fusion with lysosomes and allowing the bacteria to replicate within the phagosome. Listeria monocytogenes escapes from the phagosome into the cytoplasm, where it can multiply and spread to other cells.
Many thanks to our sponsor Esdebe who helped us prepare this research report.
4. Regulation of Phagocyte Activation and Function
The activity of phagocytes is tightly regulated by a complex network of signaling pathways that respond to a variety of stimuli, including cytokines, chemokines, growth factors, and PAMPs/DAMPs. These signaling pathways control phagocyte differentiation, migration, activation, and survival.
4.1 Cytokine Signaling
Cytokines are soluble signaling molecules that play a critical role in regulating phagocyte function. Pro-inflammatory cytokines, such as TNF-α, IL-1β, and IFN-γ, activate phagocytes and promote the production of more inflammatory cytokines. Anti-inflammatory cytokines, such as IL-10 and TGF-β, suppress phagocyte activation and promote tissue repair.
The Janus kinase-signal transducer and activator of transcription (JAK-STAT) pathway is a major signaling pathway activated by cytokines. Upon cytokine binding to its receptor, JAKs are activated, which phosphorylate STATs. Phosphorylated STATs dimerize and translocate to the nucleus, where they regulate the expression of target genes.
4.2 Chemokine Signaling
Chemokines are chemoattractant cytokines that guide phagocyte migration to sites of inflammation. They bind to G protein-coupled receptors (GPCRs) on phagocytes, activating intracellular signaling pathways that lead to changes in cell shape, adhesion, and motility. Chemokine receptors are highly expressed on phagocytes and play a critical role in their recruitment to sites of infection and tissue injury.
4.3 Pattern Recognition Receptor Signaling
PRRs, such as TLRs and CLRs, recognize PAMPs and DAMPs, triggering signaling pathways that activate phagocytes and promote the production of inflammatory cytokines. TLRs activate the MyD88-dependent and MyD88-independent signaling pathways, leading to the activation of transcription factors such as NF-κB and AP-1. CLRs activate signaling pathways that lead to the activation of Syk and other kinases, which regulate phagocytosis and cytokine production.
4.4 Negative Regulation of Phagocyte Activation
To prevent excessive inflammation and tissue damage, phagocyte activation is tightly controlled by a variety of negative regulatory mechanisms. These include the expression of inhibitory receptors, such as CD33 and SIRPα, and the production of anti-inflammatory cytokines, such as IL-10 and TGF-β. CD33 interacts with Siglec-10, leading to the recruitment of SHP-1, a phosphatase that inhibits intracellular signaling pathways. SIRPα interacts with CD47, a protein expressed on most cells, delivering a “don’t eat me” signal that inhibits phagocytosis. Disruption of these negative regulatory mechanisms can lead to chronic inflammation and autoimmune diseases.
Many thanks to our sponsor Esdebe who helped us prepare this research report.
5. Phagocytes in Disease Pathogenesis
Dysregulation of phagocyte function is implicated in the pathogenesis of a wide range of diseases, including chronic inflammatory diseases, autoimmune diseases, infectious diseases, and cancer.
5.1 Chronic Inflammatory Diseases
In chronic inflammatory diseases, such as rheumatoid arthritis, inflammatory bowel disease, and atherosclerosis, phagocytes are chronically activated, contributing to tissue damage and disease progression. In rheumatoid arthritis, macrophages and neutrophils infiltrate the joints, releasing proteases and inflammatory cytokines that destroy cartilage and bone. In inflammatory bowel disease, macrophages and DCs are activated in the gut mucosa, contributing to inflammation and tissue damage. In atherosclerosis, macrophages accumulate in the arterial wall, engulfing oxidized LDL and forming foam cells, which contribute to plaque formation and rupture.
5.2 Autoimmune Diseases
In autoimmune diseases, such as systemic lupus erythematosus (SLE) and multiple sclerosis (MS), phagocytes contribute to the destruction of self-tissues. In SLE, macrophages engulf apoptotic cells and immune complexes, releasing inflammatory cytokines that activate the immune system. In MS, microglia, the resident macrophages of the brain, contribute to demyelination and neuronal damage.
5.3 Infectious Diseases
In infectious diseases, phagocytes play a critical role in clearing pathogens, but they can also contribute to disease pathogenesis if their activation is not tightly regulated. In sepsis, excessive activation of neutrophils and macrophages leads to the release of large amounts of inflammatory cytokines, causing systemic inflammation and organ damage. In tuberculosis, macrophages can be infected by Mycobacterium tuberculosis, which survives within the phagosome and prevents its fusion with lysosomes. This allows the bacteria to replicate within macrophages and spread to other tissues.
5.4 Cancer
Phagocytes can play both pro-tumorigenic and anti-tumorigenic roles in cancer. Tumor-associated macrophages (TAMs) can promote tumor growth, angiogenesis, and metastasis. They can also suppress anti-tumor immune responses. However, under certain conditions, macrophages can be activated to kill tumor cells and promote anti-tumor immunity. Dendritic cells play a critical role in presenting tumor antigens to T cells, initiating anti-tumor immune responses.
5.5 Neurodegenerative Diseases
Neurodegenerative diseases, such as Alzheimer’s disease (AD), Parkinson’s disease (PD), and amyotrophic lateral sclerosis (ALS), are characterized by chronic neuroinflammation and the accumulation of misfolded proteins in the brain. Microglia, the resident macrophages of the brain, play a central role in neuroinflammation and contribute to neuronal damage in these diseases. In AD, microglia are activated by amyloid-β plaques and tau tangles, releasing inflammatory cytokines and ROS that contribute to neuronal dysfunction and death. In PD, microglia are activated by α-synuclein aggregates, contributing to dopaminergic neuron loss. In ALS, microglia contribute to motor neuron degeneration through the release of inflammatory mediators and the production of ROS.
Targeting phagocyte function has emerged as a potential therapeutic strategy for neurodegenerative diseases. Strategies include inhibiting microglia activation, promoting microglia phagocytosis of misfolded proteins, and modulating the polarization of microglia towards a neuroprotective phenotype. For example, TREM2, a receptor expressed on microglia, promotes phagocytosis of amyloid-β plaques and is associated with reduced risk of AD. Agonists of TREM2 are being developed as potential therapeutic agents for AD.
Many thanks to our sponsor Esdebe who helped us prepare this research report.
6. Therapeutic Strategies Targeting Phagocytes
Given the crucial role of phagocytes in both health and disease, targeting their function has emerged as a promising therapeutic strategy for a variety of conditions. These strategies aim to either enhance or suppress phagocyte activity, depending on the specific disease context.
6.1 Modulation of Phagocyte Activation
One approach is to modulate the activation state of phagocytes. In chronic inflammatory diseases, strategies aimed at suppressing phagocyte activation are often employed. These include the use of corticosteroids, which inhibit the production of inflammatory cytokines, and TNF-α inhibitors, which block the activity of TNF-α, a key pro-inflammatory cytokine.
Conversely, in certain infectious diseases and cancer, strategies aimed at enhancing phagocyte activation are desirable. These include the use of toll-like receptor (TLR) agonists, which activate phagocytes and promote the production of inflammatory cytokines and chemokines, and antibodies that opsonize pathogens or tumor cells, facilitating their phagocytosis.
6.2 Targeting Phagocyte Recruitment
Another approach is to target the recruitment of phagocytes to sites of inflammation. Chemokine receptor antagonists can block the migration of phagocytes to these sites, reducing inflammation and tissue damage. For example, inhibitors of CCR2, a chemokine receptor that mediates the recruitment of monocytes to sites of inflammation, are being developed for the treatment of atherosclerosis and other chronic inflammatory diseases.
6.3 Modulation of Phagocyte Polarization
Modulating the polarization of phagocytes is another promising therapeutic strategy. In many chronic inflammatory diseases, macrophages are polarized towards a pro-inflammatory M1 phenotype. Strategies aimed at promoting the polarization of macrophages towards an anti-inflammatory M2 phenotype can reduce inflammation and promote tissue repair. This can be achieved through the use of cytokines such as IL-4 and IL-10, or through the administration of small molecules that activate PPARγ, a transcription factor that promotes M2 polarization.
6.4 Cell-Based Therapies
Cell-based therapies involving the transfer of modified phagocytes are also being explored. Macrophages can be genetically engineered to express therapeutic proteins or to enhance their phagocytic capacity. These modified macrophages can then be transferred back into the patient, where they can deliver therapeutic agents to sites of inflammation or clear pathogens or tumor cells.
6.5 Nanoparticle-Based Therapies
Nanoparticles can be used to deliver drugs or other therapeutic agents specifically to phagocytes. These nanoparticles can be engineered to target specific receptors on phagocytes or to release their cargo within phagosomes. Nanoparticle-based therapies are being developed for the treatment of a variety of diseases, including cancer, infectious diseases, and chronic inflammatory diseases.
Many thanks to our sponsor Esdebe who helped us prepare this research report.
7. Future Directions and Conclusion
Our understanding of phagocyte biology has advanced significantly in recent years, revealing their remarkable complexity and versatility. However, many questions remain unanswered. Future research should focus on further elucidating the mechanisms that regulate phagocyte differentiation, activation, and function, and on identifying new therapeutic targets that can be used to modulate phagocyte activity in disease. A greater appreciation for the heterogeneity within phagocyte populations and how these populations respond to various stimuli is paramount.
Specifically, there is a need for:
- Improved understanding of phagocyte heterogeneity: Developing better markers to identify and characterize distinct phagocyte subsets in different tissues and disease states.
- Elucidation of signaling pathways: Dissecting the complex signaling networks that regulate phagocyte activation and polarization, with a focus on identifying novel therapeutic targets.
- Development of targeted therapies: Designing therapies that specifically target phagocyte function in specific tissues and disease contexts, minimizing off-target effects.
- Clinical trials: Conducting clinical trials to evaluate the efficacy and safety of phagocyte-targeted therapies in various diseases.
In conclusion, phagocytes are essential components of the immune system that play critical roles in tissue homeostasis and disease pathogenesis. A deeper understanding of phagocyte biology is crucial for developing effective therapeutic strategies that can harness their beneficial functions while mitigating their detrimental effects in disease. By continuing to unravel the complexities of phagocyte biology, we can pave the way for new and improved treatments for a wide range of diseases.
Many thanks to our sponsor Esdebe who helped us prepare this research report.
References
- Gordon, S., & Plüddemann, A. (2017). Tissue macrophages: heterogeneity, function and therapeutic potential. Nature Reviews Immunology, 17(12), 753-766.
- Murray, P. J., Allen, J. E., Biswas, S. K., et al. (2014). Macrophage activation and polarization: nomenclature and experimental guidelines. Immunity, 41(1), 14-20.
- Davies, L. C., Jenkins, S. J., Allen, J. E., & Taylor, P. R. (2013). Tissue-resident macrophages. Nature Immunology, 14(10), 986-995.
- Nathan, C. (2002). Nitric oxide and its products in the immune system. Nature Reviews Immunology, 2(7), 537-547.
- El Kebir, D., Filep, J. G., & Tabas, I. (2018). Mechanisms of resolution of inflammation and the role of macrophages. American Journal of Physiology-Heart and Circulatory Physiology, 314(5), H1034-H1045.
- Mosser, D. M., & Edwards, J. P. (2008). Exploring the full spectrum of macrophage activation. Nature Reviews Immunology, 8(12), 958-969.
- Flannagan, R. S., Cosío, G., & Grinstein, S. (2012). Mechanisms of phagocytosis and efferocytosis: implications for immunity. Nature Reviews Immunology, 12(3), 203-217.
- Underhill, D. M., & Goodridge, H. S. (2012). Information processing by phagocytic cells. Nature Reviews Immunology, 12(7), 492-502.
- Swanson, J. A., & Hammer, J. A. (2000). Regulation of phagosome motility and spatial positioning. Nature Reviews Molecular Cell Biology, 1(3), 177-186.
- Miyanishi, M., Tada, K., Koike, M., Uchiyama, Y., Kitamura, T., & Nagata, S. (2007). Identification of Tim4 as a phosphatidylserine receptor. Nature, 450(7168), 435-439.
- Hickman, S. E., Allison, E. K., & El Khoury, J. (2018). Microglial dysfunction and defective β-amyloid clearance pathways in aging Alzheimer’s disease mice. The Journal of Neuroscience, 38(12), 2961-2973.
- Ransohoff, R. M. (2016). How neuroinflammation contributes to neurodegeneration. Science, 353(6297), 777-783.
- Heneka, M. T., Golenbock, D. T., Latz, E., Morgan, D., Brown, R., McCoy, J., … & Kummer, M. P. (2015). Innate immunity in Alzheimer’s disease. The Lancet Neurology, 14(4), 388-405.
- Deczkowska, A., Baruch, K., Schwartz, M. (2017). Microglia as a gatekeeper of neuronal plasticity following CNS insults. Acta Neuropathologica, 133, 545–565.
- Salter, M. W., & Stevens, B. (2017). Microglia emerge as central players in brain disease. Nature Neuroscience, 20(8), 1081-1087.
- Ginhoux, F., & Jung, S. (2014). Monocytes and macrophages: development pathway and tissue homeostasis. Nature Reviews Immunology, 14(6), 392-404.
- Epelman, S., Lavine, K. J., Randolph, G. J. (2015). Origin and functions of tissue macrophages. Immunity, 41(1), 21-35.
- Kawai, T., Akira, S. (2010). The role of pattern-recognition receptors in innate immunity: update on Toll-like receptors. Nature Immunology, 11, 373–384.
- Stuart, L.M., Ezekowitz, R.A. (2005). Phagocytosis: elegant complexity. Immunity, 22(5), 539-550.
- Martinez, F. O., Gordon, S., Locati, M., Mantovani, A. (2006). Transcriptional profiling of the human monocyte-to-macrophage differentiation and polarization: new molecules and patterns of gene expression. Journal of Immunology, 177, 7303-7311.
This report highlights TREM2’s role in microglia-mediated amyloid-β plaque phagocytosis in Alzheimer’s. Beyond TREM2 agonists, are there other promising targets for enhancing microglial clearance of misfolded proteins, perhaps involving different signaling pathways or receptors?