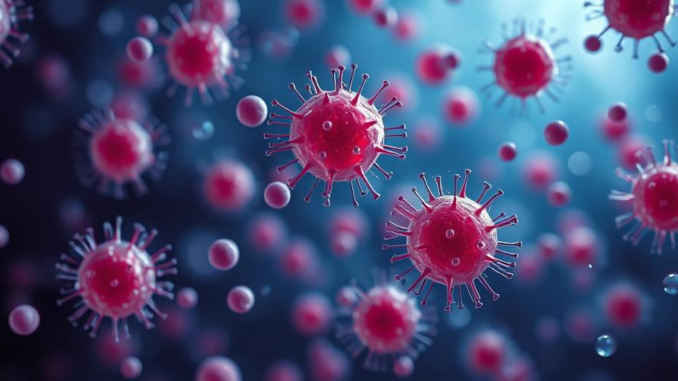
Abstract
Podocytes, terminally differentiated epithelial cells of the kidney glomerulus, are crucial for maintaining the integrity of the glomerular filtration barrier (GFB) and preventing proteinuria. Their unique structure, comprising interdigitating foot processes bridged by the slit diaphragm, ensures size- and charge-selective filtration. Podocyte dysfunction, arising from genetic mutations, hemodynamic stress (e.g., hypertension), or inflammatory mediators, is a hallmark of many glomerular diseases, ultimately leading to end-stage renal disease (ESRD). This review delves into the intricate structure and function of podocytes, exploring the molecular mechanisms underlying their susceptibility to damage. We critically examine the impact of hypertension, diabetic nephropathy, focal segmental glomerulosclerosis (FSGS), and other glomerulopathies on podocyte health, emphasizing the roles of oxidative stress, endoplasmic reticulum (ER) stress, cytoskeletal disruption, and epigenetic modifications. Furthermore, we evaluate current and emerging therapeutic strategies aimed at podocyte protection and regeneration, encompassing pharmacological interventions, gene therapy, and stem cell-based approaches. We highlight the challenges and opportunities in translating preclinical findings into effective clinical therapies for preserving podocyte integrity and preventing progressive kidney disease.
Many thanks to our sponsor Esdebe who helped us prepare this research report.
1. Introduction
The kidney, a vital organ responsible for maintaining fluid and electrolyte homeostasis and eliminating metabolic waste products, relies on the glomerulus as its primary filtration unit. Within the glomerulus, specialized cells called podocytes form the outermost layer of the glomerular filtration barrier (GFB), critically regulating the passage of molecules from the blood into the urinary space. Podocytes are terminally differentiated cells with a highly complex and specialized architecture, characterized by interdigitating foot processes connected by a specialized cell-cell junction called the slit diaphragm. This unique structure allows for selective filtration, retaining essential proteins while allowing smaller molecules, such as water, electrolytes, and metabolic waste, to pass through.
The importance of podocytes in maintaining glomerular health is underscored by the observation that podocyte dysfunction is a common feature of numerous glomerular diseases, including diabetic nephropathy, focal segmental glomerulosclerosis (FSGS), minimal change disease, and hypertensive nephropathy. Loss of podocyte integrity leads to proteinuria, a hallmark of glomerular damage, and ultimately contributes to progressive kidney disease and end-stage renal disease (ESRD). Therefore, understanding the intricate biology of podocytes, including their structure, function, and susceptibility to injury, is crucial for developing effective therapeutic strategies to protect and regenerate these essential cells.
Many thanks to our sponsor Esdebe who helped us prepare this research report.
2. Podocyte Structure and Function
2.1. Podocyte Architecture: A Tale of Interdigitation and Specialization
Podocytes exhibit a distinctive morphology characterized by a cell body (soma) that extends major processes encircling the glomerular capillaries. These major processes further branch into numerous interdigitating foot processes, creating a complex network that covers the entire glomerular basement membrane (GBM). The narrow spaces between adjacent foot processes are bridged by the slit diaphragm, a specialized cell-cell junction that functions as the final size-selective barrier in the GFB. The GBM itself, composed of collagen IV, laminin, and nidogen, also contributes to the filtration process by providing a charge-selective barrier.
The complex architecture of podocytes is maintained by a highly organized cytoskeleton, primarily composed of actin filaments. The actin cytoskeleton is dynamically regulated by various signaling pathways and is essential for podocyte shape, adhesion, and response to mechanical stress. Disruptions in the actin cytoskeleton, often induced by disease states, can lead to foot process effacement, a characteristic feature of podocyte injury, and subsequent proteinuria.
2.2. Molecular Components of the Slit Diaphragm: Gatekeepers of Filtration
The slit diaphragm is a complex protein structure that acts as a critical gatekeeper, regulating the passage of molecules across the GFB. Several key proteins are essential for the formation and function of the slit diaphragm:
- Nephrin: A transmembrane protein of the immunoglobulin superfamily, nephrin is the major structural component of the slit diaphragm. It forms homodimers between adjacent foot processes, creating a zipper-like structure that regulates filtration. Mutations in the NPHS1 gene, encoding nephrin, cause congenital nephrotic syndrome of the Finnish type, highlighting the critical role of nephrin in maintaining GFB integrity.
- Podocin: A hairpin-like protein located in the lipid rafts of the podocyte cell membrane, podocin interacts with nephrin and other slit diaphragm proteins, playing a crucial role in the assembly and stabilization of the slit diaphragm complex. Mutations in the NPHS2 gene, encoding podocin, are a common cause of autosomal recessive FSGS.
- CD2AP: A cytoplasmic adaptor protein that interacts with nephrin and podocin, CD2AP links the slit diaphragm complex to the actin cytoskeleton, providing structural support and regulating intracellular signaling pathways. Mutations in the CD2AP gene can lead to FSGS.
- TRPC6: A calcium channel that is activated by slit diaphragm proteins. Deregulation can lead to FSGS. Polymorphisms in the TRPC6 gene are associated with FSGS.
These proteins, along with other associated molecules, form a complex signaling platform that responds to mechanical stress, growth factors, and inflammatory mediators, ultimately regulating podocyte function and GFB permeability.
2.3. Podocyte Function: Beyond Filtration
While podocytes are best known for their role in glomerular filtration, they also perform other essential functions that contribute to glomerular health:
- GBM Maintenance: Podocytes synthesize and secrete components of the GBM, contributing to its structural integrity and charge selectivity. They are also involved in the turnover and remodeling of the GBM.
- Signaling and Communication: Podocytes secrete various growth factors, cytokines, and other signaling molecules that influence the behavior of other glomerular cells, including mesangial cells and endothelial cells. They also respond to signals from these cells, participating in complex cell-cell communication networks.
- Endocytosis: Podocytes possess endocytic capabilities, allowing them to remove filtered proteins and debris from the GBM, preventing clogging and maintaining GFB patency.
Many thanks to our sponsor Esdebe who helped us prepare this research report.
3. Mechanisms of Podocyte Injury
Podocyte injury is a complex process involving various mechanisms that ultimately lead to foot process effacement, detachment from the GBM, and proteinuria. Several factors contribute to podocyte damage, including:
3.1. Hemodynamic Stress: The Impact of Hypertension
Hypertension, a leading cause of chronic kidney disease, exerts significant hemodynamic stress on podocytes. Elevated glomerular capillary pressure can directly damage podocytes, leading to foot process effacement, cytoskeletal disruption, and detachment from the GBM. This mechanical stress activates various signaling pathways, including the renin-angiotensin-aldosterone system (RAAS), which further contributes to podocyte injury through increased oxidative stress and inflammation.
The RAAS, particularly angiotensin II (Ang II), plays a crucial role in mediating podocyte damage in hypertension. Ang II stimulates the production of reactive oxygen species (ROS) and inflammatory cytokines, leading to podocyte apoptosis and proteinuria. Furthermore, Ang II can directly disrupt the actin cytoskeleton, contributing to foot process effacement. The use of RAAS inhibitors, such as angiotensin-converting enzyme inhibitors (ACEIs) and angiotensin receptor blockers (ARBs), has been shown to protect podocytes and reduce proteinuria in hypertensive patients, highlighting the importance of RAAS blockade in preventing podocyte injury.
3.2. Metabolic Stress: The Role of Diabetic Nephropathy
Diabetic nephropathy (DN), a major complication of diabetes mellitus, is characterized by progressive glomerular damage, including podocyte injury. Hyperglycemia, a hallmark of diabetes, leads to increased glucose flux through various metabolic pathways, resulting in the accumulation of advanced glycation end products (AGEs) and activation of protein kinase C (PKC). These events contribute to oxidative stress, inflammation, and podocyte dysfunction.
AGEs, formed by the non-enzymatic glycation of proteins and lipids, accumulate in the GBM and podocytes, altering their structure and function. AGEs activate the receptor for AGEs (RAGE), triggering inflammatory signaling pathways that promote podocyte apoptosis and proteinuria. PKC activation also contributes to podocyte injury by disrupting the actin cytoskeleton and increasing the production of extracellular matrix proteins, leading to glomerulosclerosis.
Furthermore, hyperglycemia induces ER stress in podocytes, leading to the accumulation of unfolded or misfolded proteins in the ER. ER stress activates the unfolded protein response (UPR), a cellular stress response aimed at restoring ER homeostasis. However, prolonged or severe ER stress can overwhelm the UPR, leading to podocyte apoptosis.
3.3. Genetic Mutations: Inherited Podocytopathies
Mutations in genes encoding key podocyte proteins, such as nephrin, podocin, and CD2AP, can directly disrupt podocyte structure and function, leading to inherited podocytopathies, such as congenital nephrotic syndrome and FSGS. These mutations often impair the assembly and function of the slit diaphragm, resulting in proteinuria and progressive kidney disease.
The identification of specific gene mutations in patients with podocytopathies has provided valuable insights into the molecular mechanisms underlying podocyte function and disease pathogenesis. Genetic testing can aid in the diagnosis and management of these conditions, allowing for personalized treatment strategies.
3.4. Immunological Injury: The Impact of Glomerulonephritis
Glomerulonephritis, a group of inflammatory diseases affecting the glomeruli, can also cause podocyte injury. Immune complexes, formed by antibodies and antigens, deposit in the glomeruli, activating complement and triggering an inflammatory response. This inflammation can directly damage podocytes, leading to foot process effacement, detachment from the GBM, and proteinuria.
In some forms of glomerulonephritis, such as membranous nephropathy, antibodies target podocyte antigens, directly inducing podocyte injury. For example, antibodies against the M-type phospholipase A2 receptor (PLA2R) are a common cause of primary membranous nephropathy. Binding of anti-PLA2R antibodies to PLA2R on podocytes activates signaling pathways that lead to podocyte dysfunction and proteinuria.
3.5. Other Mechanisms: Oxidative Stress, ER Stress, and Cytoskeletal Disruption
In addition to the factors mentioned above, oxidative stress, ER stress, and cytoskeletal disruption are common pathways involved in podocyte injury, regardless of the underlying cause. Oxidative stress, characterized by an imbalance between ROS production and antioxidant defense, can damage podocyte proteins, lipids, and DNA, leading to cell dysfunction and apoptosis.
ER stress, as mentioned earlier, can also contribute to podocyte injury by activating the UPR and promoting apoptosis. Cytoskeletal disruption, often caused by mechanical stress, oxidative stress, or inflammatory mediators, impairs podocyte shape, adhesion, and function, leading to foot process effacement and proteinuria.
Epigenetic modifications, such as DNA methylation and histone acetylation, have also been implicated in podocyte injury. These modifications can alter gene expression patterns, affecting podocyte function and susceptibility to damage. For example, altered DNA methylation patterns have been observed in podocytes from patients with diabetic nephropathy, suggesting a role for epigenetic modifications in the pathogenesis of this disease.
Many thanks to our sponsor Esdebe who helped us prepare this research report.
4. Therapeutic Strategies for Podocyte Protection and Regeneration
Given the critical role of podocytes in maintaining glomerular health, therapeutic strategies aimed at podocyte protection and regeneration are essential for preventing progressive kidney disease. Several approaches are being explored, including:
4.1. Pharmacological Interventions: Targeting Specific Pathways
Various pharmacological agents have been shown to protect podocytes and reduce proteinuria by targeting specific pathways involved in podocyte injury:
- RAAS Inhibitors: ACEIs and ARBs block the RAAS, reducing Ang II levels and preventing its detrimental effects on podocytes. These agents are widely used to treat hypertension and proteinuria in patients with diabetic nephropathy and other glomerular diseases.
- Immunosuppressants: Corticosteroids, cyclosporine, and tacrolimus are immunosuppressants that suppress the immune system and reduce inflammation in glomerulonephritis. These agents can protect podocytes from immune-mediated injury and reduce proteinuria.
- Statins: Statins, commonly used to lower cholesterol levels, have also been shown to have podocyte-protective effects. Statins can reduce oxidative stress, inflammation, and ER stress in podocytes, promoting cell survival and function. Recent studies suggest that statins may stabilize the actin cytoskeleton in podocytes, counteracting the effect of foot process effacement.
- SGLT2 Inhibitors: Sodium-glucose cotransporter 2 (SGLT2) inhibitors, initially developed for the treatment of diabetes, have demonstrated renoprotective effects, including podocyte protection. They may also have anti-inflammatory effects, which can secondarily help podocytes. Some studies suggest a direct protective effect on podocytes.
- Endothelin Receptor Antagonists: Endothelin-1 (ET-1) is a potent vasoconstrictor that can contribute to podocyte injury by increasing glomerular capillary pressure and promoting oxidative stress. Endothelin receptor antagonists block the effects of ET-1, protecting podocytes and reducing proteinuria.
- Bardoxolone Methyl: Nrf2 activator, a transcription factor that regulates the expression of antioxidant genes. It has shown promising results in clinical trials for the treatment of diabetic kidney disease.
4.2. Gene Therapy: Restoring Podocyte Function
Gene therapy offers the potential to restore podocyte function by delivering therapeutic genes to damaged cells. Several gene therapy strategies are being investigated:
- Gene Replacement Therapy: Replacing mutated genes with functional copies can restore normal podocyte function in patients with inherited podocytopathies. This approach has shown promise in preclinical studies, but challenges remain in achieving efficient gene delivery to podocytes and long-term gene expression.
- Gene Editing Therapy: CRISPR-Cas9 technology allows for precise editing of the genome, offering the potential to correct mutated genes in podocytes. This approach is still in its early stages of development, but it holds great promise for treating inherited podocytopathies.
- Gene Augmentation Therapy: Introducing genes that encode for protective factors, such as antioxidants or anti-apoptotic proteins, can enhance podocyte survival and function. This approach has shown promise in preclinical studies, but further research is needed to evaluate its safety and efficacy in humans.
4.3. Stem Cell Therapy: Regenerating Damaged Podocytes
Stem cell therapy offers the potential to regenerate damaged podocytes and restore glomerular function. Several types of stem cells are being investigated for their potential to regenerate podocytes:
- Mesenchymal Stem Cells (MSCs): MSCs are multipotent stem cells that can differentiate into various cell types, including podocytes. MSCs can be derived from various sources, such as bone marrow, adipose tissue, and umbilical cord blood. MSCs can protect and repair damaged podocytes through paracrine mechanisms, secreting growth factors and cytokines that promote cell survival and angiogenesis. In vivo differentiation into podocytes is rare and questioned.
- Induced Pluripotent Stem Cells (iPSCs): iPSCs are generated by reprogramming adult somatic cells into a pluripotent state, allowing them to differentiate into any cell type in the body, including podocytes. iPSCs offer a virtually unlimited source of podocytes for cell-based therapies, but challenges remain in controlling their differentiation and preventing teratoma formation.
- Direct Reprogramming: A more recent approach involves directly converting somatic cells into podocyte-like cells, bypassing the pluripotent state. This approach offers the potential to generate podocytes more efficiently and safely than iPSC-based therapies. This approach remains technically challenging.
4.4. Exosomes: A Novel Therapeutic Approach
Exosomes, small extracellular vesicles secreted by cells, have emerged as a novel therapeutic approach for podocyte protection and regeneration. Exosomes can deliver various bioactive molecules, such as proteins, RNAs, and lipids, to recipient cells, modulating their function. Exosomes derived from healthy podocytes or MSCs can protect damaged podocytes from apoptosis, promote cell survival, and reduce proteinuria. Exosomes can also be engineered to deliver specific therapeutic molecules to podocytes, enhancing their therapeutic efficacy.
4.5 Combination Therapies: A Multifaceted Approach
Given the complex nature of podocyte injury, combination therapies that target multiple pathways may be more effective than single-agent therapies. For example, combining RAAS inhibitors with statins or SGLT2 inhibitors may provide synergistic benefits in protecting podocytes and reducing proteinuria. Future research should focus on developing and evaluating combination therapies that target multiple mechanisms of podocyte injury.
Many thanks to our sponsor Esdebe who helped us prepare this research report.
5. Challenges and Future Directions
Despite significant advances in our understanding of podocyte biology and mechanisms of podocyte injury, several challenges remain in translating preclinical findings into effective clinical therapies. These challenges include:
- Limited Podocyte Regeneration Capacity: Podocytes are terminally differentiated cells with limited regenerative capacity, making it difficult to restore podocyte number after injury. Strategies to promote podocyte regeneration, such as stem cell therapy and gene therapy, are still in their early stages of development.
- Lack of Specific Podocyte Markers: The lack of specific podocyte markers makes it difficult to track and monitor podocyte health and regeneration in vivo. The identification of novel podocyte-specific markers is crucial for developing more accurate diagnostic and therapeutic tools.
- Off-Target Effects: Gene therapy and stem cell therapy can have off-target effects, leading to unintended consequences. Careful optimization of gene delivery vectors and stem cell differentiation protocols is essential to minimize off-target effects.
- Translational Gap: Many promising preclinical findings have failed to translate into effective clinical therapies. This translational gap highlights the need for more rigorous preclinical studies and better clinical trial designs.
Future research should focus on addressing these challenges and developing more effective strategies for podocyte protection and regeneration. This includes:
- Developing Novel Podocyte-Targeted Therapies: Identifying novel targets and developing new drugs that specifically protect podocytes and promote their regeneration.
- Improving Gene Therapy and Stem Cell Therapy Strategies: Optimizing gene delivery vectors and stem cell differentiation protocols to enhance efficacy and minimize off-target effects.
- Developing Biomarkers for Podocyte Injury: Identifying and validating biomarkers that can accurately detect podocyte injury and predict disease progression.
- Conducting Rigorous Clinical Trials: Conducting well-designed clinical trials to evaluate the safety and efficacy of novel podocyte-targeted therapies.
- Utilizing Advanced Technologies: Leveraging advanced technologies, such as single-cell RNA sequencing and CRISPR-Cas9 gene editing, to gain a deeper understanding of podocyte biology and disease mechanisms.
Many thanks to our sponsor Esdebe who helped us prepare this research report.
6. Conclusion
Podocytes are critical components of the glomerular filtration barrier, and their dysfunction is a major cause of proteinuria and progressive kidney disease. Understanding the intricate structure, function, and mechanisms of injury in podocytes is essential for developing effective therapeutic strategies to protect and regenerate these essential cells. While significant progress has been made in recent years, several challenges remain in translating preclinical findings into effective clinical therapies. Future research should focus on developing novel podocyte-targeted therapies, improving gene therapy and stem cell therapy strategies, identifying biomarkers for podocyte injury, and conducting rigorous clinical trials. By addressing these challenges, we can pave the way for more effective treatments for glomerular diseases and improve the lives of patients with kidney disease.
Many thanks to our sponsor Esdebe who helped us prepare this research report.
References
[1] Kriz W, Shirato I, Nagata M, et al. Podocyte structure and function. J Am Soc Nephrol. 1996;7(12):2245-2255.
[2] Tryggvason K, Patrakka J, Wartiovaara J. Hereditary proteinuria syndromes and mechanisms of development of glomerular diseases. N Engl J Med. 2006;354(21):2295-2308.
[3] Saleem MA. Podocytes. Kidney Int. 2005;68(6):2556-2568.
[4] Pavenstädt H, Kriz W, Dunder S, et al. The podocyte slit diaphragm is a barrier for albumin filtration: a model based on ultrastructural and biochemical findings. Kidney Int. 2003;64(2):500-508.
[5] Huber TB, Benzing T. The slit diaphragm: gateway to podocyte cell biology. Curr Opin Nephrol Hypertens. 2005;14(3):211-216.
[6] Mundel P, Shankland SJ. Podocyte biology and experimentation. J Am Soc Nephrol. 2002;13(12):3005-3015.
[7] Reiser J, Polu KR, Moller CC, et al. TRPC6 is a glomerular slit diaphragm component that interacts with nephrin and podocin. J Clin Invest. 2005;115(10):2915-2925.
[8] Dai DF, Liu Y, Saleem MA, Mathieson PW. TRPC6 channels in kidney disease. Biochim Biophys Acta. 2009;1792(1):1-8.
[9] Pippin JW, Brinkkoetter PT, Nesteruk MT, et al. Podocyte detachment and nephrinuria precede albuminuria in experimental glomerular disease. J Am Soc Nephrol. 2009;20(11):2283-2295.
[10] Abbate M, Zoja C, Remuzzi G. How does proteinuria cause progressive renal damage? J Am Soc Nephrol. 2006;17(11):2974-2984.
[11] Remuzzi A, Ruggenenti P, Remuzzi G. Progression into chronic renal failure: role of intrarenal hypertension. BMJ. 1997;315(7115):1077-1081.
[12] Wolf G. New insights into the pathophysiology of diabetic nephropathy: from haemodynamics to molecular mechanisms. Eur J Clin Invest. 2004;34(12):785-796.
[13] Susztak K, Sharma K, Mazzali M, et al. Advanced glycation end products induce podocyte apoptosis through activation of the MAPK pathway. Kidney Int. 2006;69(1):125-134.
[14] Brosius FC 3rd, Tuttle KR. Advanced glycation end products and diabetic kidney disease. Semin Nephrol. 2006;26(2):126-132.
[15] Göser K, Eremina V, Baelde HJ, et al. Urokinase receptor-mediated Src activation regulates podocyte structure and function. J Am Soc Nephrol. 2008;19(1):81-93.
[16] Somlo S, Mundel P. Podocyte biology in health and disease. Curr Opin Nephrol Hypertens. 2000;9(3):229-238.
[17] Ron D, Walter P. Signal integration in the endoplasmic reticulum unfolded protein response. Nat Rev Mol Cell Biol. 2007;8(7):519-529.
[18] Huber TB, Simons M, Hartleben B, et al. Molecular basis of the functional podocyte architecture. Semin Nephrol. 2003;23(4):315-325.
[19] Canaud G, Bienaimé F, Viau A, et al. Autoimmunity to podocyte antigens in human primary membranous nephropathy. Clin Immunol. 2013;146(1):1-10.
[20] Beck LH Jr, Bonegio RG, Lambeau G, et al. M-type phospholipase A2 receptor as target antigen in idiopathic membranous nephropathy. N Engl J Med. 2009;361(1):11-21.
[21] Oxidative Stress in Kidney Disease. Oxid Med Cell Longev. 2016;2016:6975938.
[22] Cybulsky AV. Endoplasmic reticulum stress and autophagy in kidney diseases. Kidney Int. 2017;91(1):52-60.
[23] Ma FY, Zhou Y, Ito T, et al. Epigenetic modifications in podocytes in patients with diabetic nephropathy. Clin Epigenetics. 2015;7:11.
[24] Brenner BM, Cooper ME, de Zeeuw D, et al. Effects of losartan on renal and cardiovascular outcomes in patients with type 2 diabetes and nephropathy. N Engl J Med. 2001;345(12):861-869.
[25] Lewis EJ, Hunsicker LG, Bain RP, Rohde RD. The effect of angiotensin-converting enzyme inhibition on diabetic nephropathy. The Collaborative Study Group. N Engl J Med. 1993;329(20):1456-1462.
[26] Schlondorff D. Endothelins in the kidney. Kidney Int. 1996;49(1):19-29.
[27] Kohan DE, Barton M. Endothelin and endothelin antagonists in chronic kidney disease. Kidney Int. 2014;86(2):263-275.
[28] Reidy K, Sharma R, McCarthy ET, et al. Podocyte regeneration in experimental focal segmental glomerulosclerosis. Am J Pathol. 2001;158(4):1175-1181.
[29] Shankland SJ, Pippin JW, Reidy KJ. Podocyte injury and proteinuria: role of podocyte detachment. Pediatr Nephrol. 2001;16(3):278-294.
[30] Lee EY, Chung CH, Khoury H, et al. Mesenchymal stem cells restore podocyte structure and function in diabetic nephropathy. Am J Physiol Renal Physiol. 2015;309(11):F984-F993.
[31] Choi YJ, Kim HJ, Hwang SH, et al. Paracrine effects of human umbilical cord blood-derived mesenchymal stem cells on podocyte protection in diabetic nephropathy. BMC Nephrol. 2017;18(1):124.
[32] Nagata M. Podocyte injury and its consequences. Kidney Int. 2016;89(6):1221-1230.
[33] Kanwar YS, Wada J, Sun L, et al. Diabetic nephropathy: mechanisms and therapeutic potential. Clin Biochem. 2008;41(7-8):467-494.
[34] Alicka M, Grzegorzewska AE. Renoprotective effect of statins. Pol Arch Med Wewn. 2012;122(11):561-568.
[35] Wanner C, Lachin JM, Inzucchi SE, et al. Empagliflozin and Progression of Kidney Disease in Type 2 Diabetes. N Engl J Med. 2020;383(15):1436-1446.
[36] Perico N, Benigni A, Remuzzi G. New therapeutic approaches for chronic nephropathies. J Am Soc Nephrol. 2005;16 Suppl 2:S69-S79.
[37] Komers R, Plotkin JS, Thaunat O, et al. Bardoxolone methyl in type 2 diabetes and stage 4 chronic kidney disease. N Engl J Med. 2011;364(2):129-139.
[38] Fornoni A, Merscher-Gomez S, Shankland SJ. Podocyte biology and novel therapeutic targets for proteinuric kidney diseases. Semin Nephrol. 2011;31(4):356-368.
[39] Zhou L, Zhang L, Shi S, et al. Exosomes derived from human urine-derived stem cells protect against glomerular podocyte injury in diabetic nephropathy. Stem Cell Res Ther. 2020;11(1):6.
The discussion of combination therapies is particularly interesting, given the multifaceted nature of podocyte injury. Investigating synergistic effects of combined pharmacological and regenerative approaches could significantly improve treatment outcomes for glomerular diseases.
Great point! I agree that combination therapies hold immense promise. Exploring the interplay between pharmacological interventions and regenerative strategies, such as stem cell-derived exosomes, could unlock novel synergistic effects and substantially improve outcomes for those with glomerular diseases. It’s an exciting avenue for future research!
Editor: MedTechNews.Uk
Thank you to our Sponsor Esdebe
The discussion around pharmacological interventions raises interesting questions about specificity. Could more research focus on developing drugs that selectively target diseased podocytes, minimizing off-target effects in other kidney cells or systems?
That’s an excellent point regarding the specificity of pharmacological interventions! Exploring targeted drug delivery systems, like nanoparticles functionalized with podocyte-specific antibodies, could be a promising avenue to enhance specificity and minimize systemic side effects. This would allow for higher drug concentrations at the site of injury, leading to improved therapeutic outcomes.
Editor: MedTechNews.Uk
Thank you to our Sponsor Esdebe