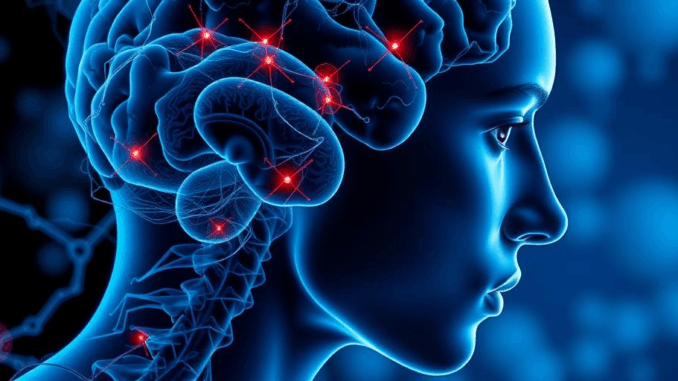
Abstract
Prion diseases, or transmissible spongiform encephalopathies (TSEs), represent a unique class of neurodegenerative disorders characterized by the misfolding and aggregation of the cellular prion protein (PrPC) into a pathogenic isoform (PrPSc). This review delves into the intricacies of prion biology, spanning the structural nuances of PrP isoforms, the mechanisms underlying prion conversion and propagation, the spectrum of prion diseases affecting humans and animals, the diagnostic challenges posed by these disorders, and the current landscape of therapeutic development. Furthermore, we explore emerging research areas, including the role of post-translational modifications, the influence of the cellular environment on prion propagation, and the potential for prion-like mechanisms in other neurodegenerative diseases. We aim to provide a comprehensive overview of the current understanding of prion biology, highlighting both established knowledge and areas ripe for future investigation.
Many thanks to our sponsor Esdebe who helped us prepare this research report.
1. Introduction
The concept of a transmissible proteinaceous infectious particle, or prion, revolutionized our understanding of infectious disease and protein folding. Stanley Prusiner’s groundbreaking work, culminating in the Nobel Prize in Physiology or Medicine in 1997, challenged the dogma that infectious agents must contain nucleic acids. Prion diseases, now understood to be caused by the misfolding of the cellular prion protein (PrPC) into a self-propagating, infectious isoform (PrPSc), encompass a range of fatal neurodegenerative disorders affecting both humans and animals. These diseases are characterized by long incubation periods, progressive neurological dysfunction, and a distinctive spongiform encephalopathy observed in the brain.
The importance of understanding prion biology extends beyond the immediate threat of prion diseases. The mechanisms of protein misfolding, aggregation, and cell-to-cell spread observed in prion diseases have profound implications for other neurodegenerative disorders, such as Alzheimer’s disease, Parkinson’s disease, and amyotrophic lateral sclerosis (ALS), where similar protein aggregation processes are implicated. This “prion-like” behavior in other proteins is an area of intense research, suggesting a unifying principle in the pathogenesis of these devastating conditions. Therefore, a comprehensive understanding of prion biology is crucial not only for developing effective treatments for prion diseases but also for gaining insights into the broader landscape of neurodegenerative disorders.
Many thanks to our sponsor Esdebe who helped us prepare this research report.
2. Structure and Function of Prion Protein Isoforms
The prion protein (PrP) is a highly conserved glycoprotein found in a variety of cell types, with the highest expression levels observed in neurons. The human PrP gene ( PRNP ) is located on chromosome 20 and encodes a protein of approximately 253 amino acids. PrP undergoes several post-translational modifications, including the addition of a glycosylphosphatidylinositol (GPI) anchor, N-linked glycosylation at two sites, and the formation of a disulfide bond. These modifications are crucial for proper folding, trafficking, and function of PrPC.
2.1. PrPC: The Cellular Isoform
PrPC is a predominantly α-helical protein, characterized by a flexible N-terminal domain and a structured C-terminal domain. The C-terminal domain contains three α-helices and a two-stranded antiparallel β-sheet. While the precise function of PrPC remains a subject of debate, several roles have been proposed, including:
- Copper binding: PrPC binds copper ions, potentially playing a role in copper homeostasis and oxidative stress protection. The N-terminal domain contains octapeptide repeats that are responsible for copper binding.
- Cell signaling: PrPC interacts with various signaling molecules, modulating neuronal excitability, cell survival, and synaptic plasticity.
- Cell adhesion: PrPC can mediate cell-cell interactions, potentially contributing to neuronal development and maintenance.
- Myelin Maintenance: Recent research has implicated PrPC in the maintenance of myelin sheaths surrounding nerve fibers.
The physiological function of PrPC is clearly complex and likely involves multiple roles depending on the cellular context. Further research is needed to fully elucidate the specific functions of PrPC and how these functions are altered in prion diseases. While PrPC knockout mice are viable and generally healthy, they exhibit subtle neurological deficits, suggesting that PrPC plays a role in long-term neuronal health.
2.2. PrPSc: The Pathogenic Isoform
PrPSc is a misfolded isoform of PrPC that is characterized by a higher β-sheet content and a propensity to aggregate into protease-resistant oligomers and amyloid fibrils. The precise structural details of PrPSc are still not fully understood due to the challenges associated with its insolubility and aggregation propensity. However, structural studies using techniques such as cryo-electron microscopy and hydrogen-deuterium exchange mass spectrometry have provided valuable insights into the architecture of PrPSc aggregates.
The conversion of PrPC to PrPSc is a self-templating process, where PrPSc acts as a seed to induce the misfolding of PrPC molecules. This conversion process is believed to involve a conformational change in PrPC, leading to the exposure of hydrophobic regions and the formation of intermolecular β-sheet interactions. These interactions drive the aggregation of PrPSc molecules into oligomers and amyloid fibrils, which are thought to be the primary infectious agents in prion diseases.
2.3. Prion Strains
A particularly perplexing aspect of prion biology is the existence of prion strains. Prion strains are defined as isolates that exhibit distinct incubation periods, lesion profiles, and clinical signs in the same host. These strain-specific properties are thought to be encoded by different conformations of PrPSc, leading to variations in the fibril structure and aggregation properties. The precise mechanism by which PrPSc conformation dictates prion strain properties is still not fully understood, but it likely involves differences in the rate of PrPC conversion, the stability of PrPSc aggregates, and the interactions of PrPSc with cellular components. This challenges the standard protein only hypothesis, suggesting that the same protein can cause multiple diseases.
Many thanks to our sponsor Esdebe who helped us prepare this research report.
3. Mechanisms of Prion Conversion and Propagation
The conversion of PrPC to PrPSc is a complex process that is influenced by several factors, including the amino acid sequence of PrP, the presence of cofactors, and the cellular environment. The prevailing model for prion conversion is the “seeded polymerization” or “template-assisted conversion” model, in which PrPSc acts as a template to induce the misfolding of PrPC. This process involves the interaction of PrPC with PrPSc, leading to a conformational change in PrPC and its incorporation into the growing PrPSc aggregate.
3.1. Cellular Location of Prion Conversion
The precise cellular location of prion conversion remains a subject of investigation. Evidence suggests that prion conversion can occur at the cell surface, in endosomes, and in lysosomes. The involvement of different cellular compartments may depend on the specific prion strain and the cell type. Lipid rafts, specialized microdomains in the plasma membrane enriched in cholesterol and sphingolipids, have been implicated in PrPC-PrPSc interactions and prion conversion. Endocytosis of PrPSc into endosomes and lysosomes may be a key step in prion propagation and degradation.
3.2. The Role of Cofactors
Mounting evidence suggests that cofactors, such as lipids, RNA, and other proteins, can play a crucial role in prion conversion and propagation. These cofactors may stabilize PrPSc aggregates, promote PrPC-PrPSc interactions, or facilitate the replication of prions. For example, certain lipids, such as phosphatidylinositol, have been shown to enhance prion formation in vitro. Similarly, RNA molecules have been found to be associated with PrPSc aggregates and may contribute to prion infectivity. The nature and role of these cofactors are still being actively investigated.
3.3. Cellular Mechanisms of Prion Propagation
Once PrPSc is formed, it can propagate through the body via several mechanisms, including:
- Cell-to-cell transmission: PrPSc can be transmitted from cell to cell through direct contact, exosomes, tunneling nanotubes, or the release of prions into the extracellular space. Exosomes, small vesicles secreted by cells, have been shown to contain PrPSc and can mediate the spread of prions to neighboring cells.
- Axonal transport: PrPSc can be transported along axons, allowing for the spread of prions from the periphery to the central nervous system. This axonal transport may involve both anterograde and retrograde transport mechanisms.
- Hematogenous spread: In some cases, PrPSc can spread through the bloodstream, allowing for the dissemination of prions to different organs and tissues. The precise mechanisms of hematogenous spread are not fully understood but may involve the association of PrPSc with blood cells or plasma proteins.
3.4 Prion Clearance Mechanisms
It is crucial to remember that prion disease arises from an imbalance between prion production and prion clearance. There are several cellular mechanisms in place to attempt to clear PrPSc. These include, autophagocytosis, proteosomal degradation and lysosomal degradation. Impairment of these systems can lead to disease susceptability.
Many thanks to our sponsor Esdebe who helped us prepare this research report.
4. Prion Diseases in Humans and Animals
Prion diseases affect a wide range of mammalian species, including humans, sheep, cattle, deer, and elk. These diseases are characterized by a long incubation period, progressive neurological dysfunction, and a distinctive spongiform encephalopathy observed in the brain. Prion diseases can arise spontaneously, through genetic mutations, or through acquired infection.
4.1. Human Prion Diseases
Human prion diseases are relatively rare, with an estimated incidence of 1-2 cases per million people per year. The most common human prion disease is Creutzfeldt-Jakob disease (CJD), which can occur sporadically (sCJD), genetically (gCJD), or through acquired infection (vCJD, iatrogenic CJD). Other human prion diseases include Gerstmann-Sträussler-Scheinker syndrome (GSS), fatal familial insomnia (FFI), and kuru.
- Sporadic Creutzfeldt-Jakob Disease (sCJD): sCJD accounts for the majority of CJD cases and typically occurs in individuals over the age of 60. The etiology of sCJD is unknown, but it is thought to arise from the spontaneous misfolding of PrPC.
- Genetic Creutzfeldt-Jakob Disease (gCJD): gCJD is caused by mutations in the PRNP gene. These mutations can alter the structure of PrP and increase its susceptibility to misfolding. Different PRNP mutations are associated with different clinical phenotypes.
- Variant Creutzfeldt-Jakob Disease (vCJD): vCJD is an acquired prion disease that is linked to the consumption of beef contaminated with bovine spongiform encephalopathy (BSE), also known as mad cow disease. vCJD typically affects younger individuals and is characterized by distinct clinical and neuropathological features compared to sCJD.
- Gerstmann-Sträussler-Scheinker Syndrome (GSS): GSS is a rare genetic prion disease that is characterized by ataxia, cognitive decline, and the presence of amyloid plaques in the brain. GSS is caused by specific mutations in the PRNP gene.
- Fatal Familial Insomnia (FFI): FFI is a rare genetic prion disease that is characterized by progressive insomnia, autonomic dysfunction, and motor abnormalities. FFI is caused by a specific mutation in the PRNP gene.
- Kuru: Kuru is an acquired prion disease that was prevalent among the Fore people of Papua New Guinea. Kuru was transmitted through ritualistic cannibalism, where the brains of deceased individuals were consumed.
4.2. Animal Prion Diseases
Animal prion diseases include scrapie in sheep and goats, bovine spongiform encephalopathy (BSE) in cattle, chronic wasting disease (CWD) in deer and elk, and transmissible mink encephalopathy (TME) in mink.
- Scrapie: Scrapie is a prion disease that affects sheep and goats. Scrapie is thought to be transmitted through contact with infected animals or contaminated environments.
- Bovine Spongiform Encephalopathy (BSE): BSE, also known as mad cow disease, is a prion disease that affects cattle. BSE is thought to have originated from the feeding of cattle with meat and bone meal contaminated with scrapie prions.
- Chronic Wasting Disease (CWD): CWD is a prion disease that affects deer and elk. CWD is highly contagious and can be transmitted through direct contact, contaminated environments, or bodily fluids.
- Transmissible Mink Encephalopathy (TME): TME is a prion disease that affects mink. TME is thought to be transmitted through the consumption of contaminated feed.
The interspecies transmission of prion diseases is a significant concern, as evidenced by the emergence of vCJD in humans following the BSE outbreak in cattle. The susceptibility of different species to prion infection depends on the degree of homology between the PrP sequences of the donor and recipient species. Species barriers can limit the transmission of prions between species, but these barriers can be overcome with prolonged exposure or through adaptation of prions to a new host.
Many thanks to our sponsor Esdebe who helped us prepare this research report.
5. Diagnosis and Challenges in Prion Disease Detection
The diagnosis of prion diseases can be challenging due to the long incubation periods, the variability in clinical presentation, and the lack of sensitive and specific diagnostic tests. A definitive diagnosis of prion disease typically requires neuropathological examination of brain tissue, but several diagnostic tests can be used to support the diagnosis in living patients.
5.1. Diagnostic Tests
- MRI: Magnetic resonance imaging (MRI) can reveal characteristic patterns of brain atrophy and hyperintensity in certain brain regions, such as the basal ganglia and thalamus, in patients with prion diseases. Diffusion-weighted imaging (DWI) is particularly sensitive for detecting early changes in the brain in sCJD.
- EEG: Electroencephalography (EEG) can reveal characteristic periodic sharp wave complexes (PSWCs) in patients with sCJD. However, PSWCs are not always present and may be absent in other prion diseases.
- CSF Analysis: Cerebrospinal fluid (CSF) analysis can detect elevated levels of certain proteins, such as 14-3-3 protein, total tau protein, and phosphorylated tau protein, in patients with prion diseases. However, these markers are not specific for prion diseases and can be elevated in other neurological conditions.
- RT-QuIC: Real-time quaking-induced conversion (RT-QuIC) is a highly sensitive and specific assay that detects PrPSc in CSF or olfactory mucosa samples. RT-QuIC is based on the principle of prion seeding, where PrPSc in the sample induces the misfolding and aggregation of recombinant PrP, which can be detected using fluorescence.
- PrPSc Detection in Brain Tissue: The gold standard for diagnosing prion disease is the detection of PrPSc in brain tissue using immunohistochemistry or Western blotting. Immunohistochemistry allows for the visualization of PrPSc deposits in the brain, while Western blotting allows for the detection of protease-resistant PrPSc fragments.
5.2. Challenges in Diagnosis
The diagnosis of prion diseases is often delayed due to the insidious onset of symptoms and the lack of awareness among clinicians. The clinical presentation of prion diseases can be variable, and the symptoms can overlap with other neurological disorders. Furthermore, the diagnostic tests for prion diseases are not always readily available or accessible.
One of the major challenges in prion disease diagnosis is the development of sensitive and specific diagnostic tests that can detect PrPSc in readily accessible tissues, such as blood or urine. The development of such tests would allow for early diagnosis and potentially enable the implementation of therapeutic interventions before irreversible brain damage occurs.
Many thanks to our sponsor Esdebe who helped us prepare this research report.
6. Therapeutic Strategies and Current Research Efforts
Currently, there are no effective treatments for prion diseases, and the prognosis is invariably fatal. However, significant research efforts are underway to develop potential therapies that can prevent prion conversion, inhibit prion propagation, or protect neurons from prion-induced damage.
6.1. Anti-Prion Compounds
Several compounds have been identified that can inhibit prion conversion or reduce PrPSc levels in vitro and in vivo. These compounds include:
- Tricyclic compounds: Quinacrine and related tricyclic compounds have been shown to inhibit prion conversion and prolong survival in animal models of prion disease. However, quinacrine has limited efficacy in human prion diseases and can cause significant side effects.
- Polyanionic compounds: Pentosan polysulfate (PPS) is a polyanionic compound that has been shown to inhibit prion propagation and prolong survival in animal models of prion disease. PPS is currently being used in compassionate use programs for patients with CJD, but the efficacy of PPS in humans is still uncertain.
- Antibodies: Antibodies that bind to PrPC or PrPSc can inhibit prion conversion and protect neurons from prion-induced damage. Several anti-PrP antibodies are currently being developed for therapeutic use in prion diseases.
- Small interfering RNAs (siRNAs): siRNAs that target the PRNP gene can reduce PrPC expression and inhibit prion propagation. siRNAs are being explored as a potential therapeutic strategy for prion diseases.
6.2. Immunotherapy
Immunotherapy approaches, such as vaccination with PrPC or PrPSc, have been explored as a potential strategy for preventing prion infection. However, the development of effective prion vaccines has been challenging due to the self-tolerance of the immune system to PrPC. Strategies to overcome self-tolerance and enhance the immune response to PrPSc are being actively investigated.
6.3. Gene Therapy
Gene therapy approaches, such as adeno-associated virus (AAV)-mediated delivery of anti-PrP antibodies or siRNAs, are being explored as a potential strategy for reducing PrPC expression and inhibiting prion propagation. Gene therapy offers the potential for long-term therapeutic benefit with a single administration.
6.4. Targeting Cellular Pathways
Research is also focused on identifying cellular pathways that are involved in prion-induced neurodegeneration and developing therapies that can target these pathways. For example, studies have shown that prion infection can activate the unfolded protein response (UPR) and induce ER stress, leading to neuronal apoptosis. Therapies that can modulate the UPR or reduce ER stress may be beneficial in prion diseases.
6.5. Challenges in Therapeutic Development
The development of effective therapies for prion diseases is challenging due to several factors, including the long incubation periods, the lack of sensitive biomarkers for early diagnosis, and the difficulty in delivering therapeutic agents to the brain. Furthermore, the prion strain and the genetic background of the host can influence the efficacy of therapeutic interventions.
Despite these challenges, significant progress is being made in the development of potential therapies for prion diseases. The identification of novel anti-prion compounds, the development of improved diagnostic tests, and the increasing understanding of the mechanisms of prion-induced neurodegeneration are paving the way for future therapeutic breakthroughs.
Many thanks to our sponsor Esdebe who helped us prepare this research report.
7. Emerging Research Areas
Several emerging research areas are expanding our understanding of prion biology and opening new avenues for therapeutic development.
7.1. Role of Post-Translational Modifications
The role of post-translational modifications, such as glycosylation and GPI anchor modification, in prion conversion and propagation is an area of intense investigation. Different glycosylation patterns of PrPC have been shown to influence its susceptibility to misfolding and its interaction with PrPSc. Similarly, variations in the GPI anchor structure can affect the trafficking and localization of PrPC and its interaction with lipid rafts.
7.2. Influence of the Cellular Environment
The influence of the cellular environment on prion propagation is another important area of research. Factors such as the presence of chaperones, the redox state of the cell, and the availability of nutrients can influence the rate of prion conversion and the formation of PrPSc aggregates. Understanding how these factors affect prion propagation can help to identify novel therapeutic targets.
7.3. Prion-Like Mechanisms in Other Neurodegenerative Diseases
The potential for prion-like mechanisms in other neurodegenerative diseases, such as Alzheimer’s disease, Parkinson’s disease, and ALS, is a rapidly growing area of research. In these diseases, misfolded proteins, such as amyloid-β, tau, α-synuclein, and TDP-43, can aggregate and spread from cell to cell, leading to progressive neurodegeneration. The mechanisms of protein misfolding, aggregation, and cell-to-cell spread in these diseases share similarities with those observed in prion diseases, suggesting that prion-like mechanisms may play a central role in the pathogenesis of these disorders.
7.4. Novel Detection Methods
New detection methods are being developed to detect prions earlier in the disease process and in more accessible bodily fluids. For example, research into improved RT-QuIC protocols, as well as amplification methods such as PMCA (Protein Misfolding Cyclic Amplification) are allowing for the detection of previously undetectable levels of PrPSc.
Many thanks to our sponsor Esdebe who helped us prepare this research report.
8. Conclusion
Prion biology represents a fascinating and complex field with profound implications for our understanding of infectious disease and neurodegeneration. The unique mechanisms of prion conversion, propagation, and neurotoxicity have challenged conventional biological paradigms and opened new avenues for research. While significant progress has been made in understanding the structure, function, and pathogenesis of prions, many questions remain unanswered.
The development of effective therapies for prion diseases remains a major challenge, but ongoing research efforts are yielding promising results. The identification of novel anti-prion compounds, the development of improved diagnostic tests, and the increasing understanding of the mechanisms of prion-induced neurodegeneration are paving the way for future therapeutic breakthroughs. Furthermore, the insights gained from prion research are providing valuable information for understanding the pathogenesis of other neurodegenerative diseases, where prion-like mechanisms may play a central role. The future of prion research is bright, and continued efforts in this field will undoubtedly lead to new discoveries that will benefit both patients with prion diseases and those with other neurodegenerative disorders.
Many thanks to our sponsor Esdebe who helped us prepare this research report.
References
- Prusiner, S. B. (1998). Prions. Proceedings of the National Academy of Sciences, 95(23), 13363-13383.
- Aguzzi, A., & Calella, A. M. (2009). Prions: protein aggregation and infectious diseases. Physiological reviews, 89(4), 1105-1152.
- Colby, D. W., & Prusiner, S. B. (2011). Prions. Cold Spring Harbor perspectives in biology, 3(1), a006833.
- Wille, H., Prusiner, S. B., & DeArmond, S. J. (2017). Prion strains. Cold Spring Harbor perspectives in biology, 9(3), a023564.
- Caughey, B., & Chesebro, B. (2001). Prion protein and the transmissible spongiform encephalopathies. Trends in Biochemical Sciences, 26(5), 321-328.
- Watts, J. C., Drisaldi, B., Stewart-Jones, G. B., Westaway, D., & Fraser, P. E. (2018). The cell biology of prion proteins. Cold Spring Harbor perspectives in biology, 10(8), a029074.
- Sim, V. L., Caughey, B. (2009). Recent advances in prion diagnostics. Clin Lab Med, 29(1), 43-61.
- Geschwind, M. D. (2015). Prion diseases. Continuum (Minneapolis, Minn.), 21(6, Neuro-infectious Disease), 1612–1638.
- Vallabh, S. M., Minikel, E. V., Schreiber, S. L., & Lander, E. S. (2020). Towards a treatment for genetic prion disease: trials and biomarkers. The Lancet Neurology, 19(6), 522-530.
- Raymond, G.J., & Caughey, B. (2018). Prion protein conversion: insight from cell-free studies. Prion, 12(2), 74-84
- Westergard, L., Christensen, H. M., & Harris, D. A. (2011). The cellular prion protein (PrP C): its physiological function and role in disease. Biochimica et Biophysica Acta (BBA)-Molecular Basis of Disease, 1812(6), 526-544.
- Mehrabian, M., Brener, A., & Regev, L. (2023). Prion Diseases: Advances in Pathogenesis, Diagnosis, and Therapy. Molecular Neurobiology, 60(11), 6355-6375
- Aguzzi, A., Baranyi, A., Nuvolone, M., & Schwietzke, D. (2013). Prion immunology. Immunological reviews, 255(1), 91-107.
- Soto, C. (2021). Prion propagation, toxicity and therapeutics in neurodegenerative diseases. Nature Reviews Neurology, 17(1), 51-66.
- Moda, F., Gambetti, P., Notari, S., Concha, M. A., Windl, O., Collins, S. J., … & Giaccone, G. (2012). Prions in the peripheral nerves of patients with Creutzfeldt–Jakob disease. New England Journal of Medicine, 366(2), 111-117.
- Makarava, N., Savtikov, D., & Baskakov, I. V. (2021). Lipid cofactors in prion conversion and replication. Cold Spring Harbor perspectives in biology, 13(3), a038869.
- Schmitz, M., Ebert, E., Stoeck, K., Karch, A., Collins, S., Calero, M., … & Zerr, I. (2016). Validation of 14‐3‐3 protein as a marker in sporadic Creutzfeldt–Jakob disease diagnostic. Brain, 139(7), 2026-2038.
- Atarashi, R., Satoh, K., Sano, K., Fuse, T., Yamaguchi, N., Ishibashi, D., … & Nishida, N. (2011). Ultrasensitive human prion detection in cerebrospinal fluid. Nature medicine, 17(2), 175-178.
- Imberdis, T., Roffé, G., & De Strooper, B. (2019). The roles of cell-to-cell transmission in neurodegenerative diseases. Nature Reviews Neuroscience, 20(2), 117-128.
- Hetz, C., Checheni, F., Ochs, K., Rose, O., Glimcher, L. H., Rogers, M., … & Maundrell, K. (2003). ER stress mediates neuronal cell death after prion infection. Journal of Cell Biology, 161(1), 41-51.
Regarding emerging research areas, what potential do improved prion detection methods, such as enhanced RT-QuIC protocols, hold for earlier diagnosis and intervention in prion diseases?
That’s a great question! Enhanced RT-QuIC and similar advancements offer the potential for much earlier diagnosis, even pre-symptomatically. This could be game-changing, allowing for preventative or disease-modifying interventions if effective treatments are developed. It would also greatly aid in risk assessment and management for at-risk individuals.
Editor: MedTechNews.Uk
Thank you to our Sponsor Esdebe
Fascinating! So, could we theoretically train dogs to sniff out misfolded proteins like they do for other diseases? A canine early warning system for prion diseases could be quite the innovation!