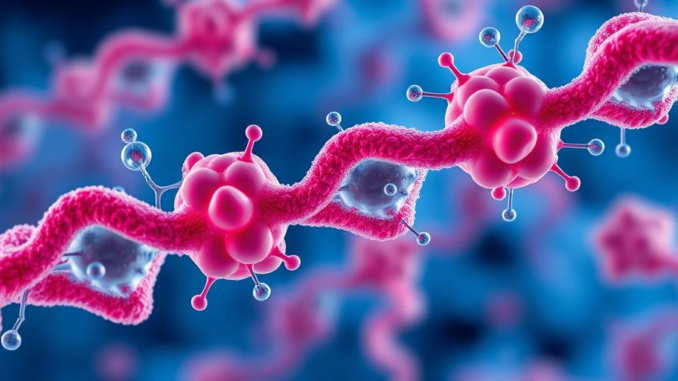
Protein Biomarkers in Cancer: From Discovery to Clinical Application
Many thanks to our sponsor Esdebe who helped us prepare this research report.
Abstract
Cancer remains a leading cause of mortality worldwide, necessitating the development of more effective diagnostic and therapeutic strategies. Protein biomarkers hold immense promise for early cancer detection, prognosis, and prediction of treatment response. This report provides a comprehensive overview of protein biomarkers in cancer, encompassing their diverse types, analytical methodologies for detection, and clinical applications. It delves into the intricacies of biomarker discovery, validation, and implementation, highlighting the challenges associated with sensitivity, specificity, variability, and the need for multiplexed approaches. Furthermore, the report explores emerging technologies and strategies aimed at overcoming these limitations, focusing on proteomics, glycomics, circulating tumor cells, extracellular vesicles, and the integration of artificial intelligence. Finally, the report critically examines the current state of protein biomarker use in cancer management and outlines future directions for advancing the field, ultimately aiming to improve patient outcomes.
Many thanks to our sponsor Esdebe who helped us prepare this research report.
1. Introduction
Cancer is a complex and heterogeneous disease characterized by uncontrolled cell growth and the potential to invade and metastasize to distant sites. Early detection and accurate diagnosis are crucial for improving patient survival rates. Traditional methods, such as imaging and histopathology, often lack the sensitivity and specificity required for early-stage detection or fail to provide comprehensive information about disease heterogeneity and progression. Therefore, the identification and validation of reliable biomarkers are essential for improving cancer management across the continuum of care. Biomarkers can be broadly defined as measurable indicators of a biological state or condition. In the context of cancer, biomarkers can include DNA, RNA, proteins, metabolites, or even whole cells. Protein biomarkers, in particular, have gained significant attention due to their direct involvement in cellular processes and their potential to reflect the dynamic changes occurring within the tumor microenvironment. This report will focus on protein biomarkers, exploring their various forms, analytical methods, challenges, and current and future clinical applications.
Many thanks to our sponsor Esdebe who helped us prepare this research report.
2. Types of Protein Biomarkers in Cancer
Protein biomarkers in cancer can be categorized based on their origin, function, and clinical utility. These include:
2.1. Circulating Tumor-Associated Antigens (TAAs): These are proteins released by tumor cells into the bloodstream or other bodily fluids. Examples include:
- Carcinoembryonic antigen (CEA): Elevated levels are associated with various cancers, including colorectal, lung, and breast cancers. While CEA is a widely used biomarker, its sensitivity and specificity are often limited, and it is also elevated in certain benign conditions. Its primary utility lies in monitoring treatment response and detecting recurrence.
- Prostate-specific antigen (PSA): PSA is primarily used for prostate cancer screening and monitoring. However, it can also be elevated in benign prostatic hyperplasia (BPH) and prostatitis, leading to overdiagnosis and overtreatment. Refinements like PSA isoforms (e.g., free PSA, proPSA) and the Prostate Health Index (PHI) have improved specificity.
- Cancer antigen 125 (CA-125): Primarily used for ovarian cancer monitoring, but also elevated in other cancers and benign conditions such as endometriosis and pregnancy. Its clinical utility is limited for early detection due to low sensitivity.
- Cancer antigen 19-9 (CA19-9): Frequently used for pancreatic cancer, but can also be elevated in other gastrointestinal cancers and benign conditions like pancreatitis and cholangitis. Its sensitivity for early pancreatic cancer detection is poor.
2.2. Enzymes: Altered expression or activity of certain enzymes can indicate cancer development or progression. Examples include:
- Lactate dehydrogenase (LDH): A general marker of tissue damage and cell turnover. Elevated levels can be observed in various cancers, but it lacks specificity.
- Prostatic acid phosphatase (PAP): Historically used for prostate cancer detection, but largely replaced by PSA due to its inferior sensitivity and specificity.
- Creatine kinase-MB (CK-MB): Primarily a marker of cardiac damage, but may be elevated in certain muscle-related cancers.
2.3. Growth Factors and Cytokines: These proteins play crucial roles in cell proliferation, angiogenesis, and immune modulation, and their dysregulation is often associated with cancer. Examples include:
- Vascular endothelial growth factor (VEGF): Promotes angiogenesis, a critical process for tumor growth and metastasis. Elevated levels are associated with various cancers.
- Epidermal growth factor receptor (EGFR): A receptor tyrosine kinase that regulates cell growth and survival. Overexpression or activating mutations are common in several cancers, making it a target for targeted therapies.
- Interleukin-6 (IL-6): A cytokine involved in inflammation and immune response. Elevated levels are associated with cancer progression and poor prognosis.
2.4. Apoptosis-Related Proteins: These proteins regulate programmed cell death, and their altered expression can contribute to cancer development. Examples include:
- Bcl-2 family proteins: A family of proteins that regulate apoptosis, including pro-apoptotic (e.g., Bax, Bak) and anti-apoptotic (e.g., Bcl-2, Bcl-xL) members. Dysregulation of these proteins can promote cancer cell survival.
- Caspases: A family of proteases that execute the apoptotic program. Altered caspase activity can disrupt apoptosis and contribute to cancer development.
2.5. Intracellular Signaling Proteins: Proteins involved in intracellular signaling pathways are frequently dysregulated in cancer. Examples include:
- PI3K/Akt/mTOR pathway proteins: This pathway regulates cell growth, survival, and metabolism. Activating mutations or overexpression of pathway components are common in many cancers.
- MAPK pathway proteins: This pathway regulates cell proliferation, differentiation, and apoptosis. Activating mutations or overexpression of pathway components are also frequently observed in cancer.
2.6. Protein Fragments and Modifications: Cancer cells often produce unique protein fragments or modifications that can serve as biomarkers. Examples include:
- Glycosylation changes: Altered glycosylation patterns are common in cancer cells and can be used as biomarkers. Glycans can be modified by adding or removing monosaccharides, changing the branching pattern, or adding different types of sugars. These changes can affect protein folding, stability, and interactions with other molecules.
- Proteolytic fragments: Cancer cells can produce unique protein fragments through altered proteolytic activity. These fragments can be detected in bodily fluids and used as biomarkers.
- Phosphorylation changes: Abnormal phosphorylation patterns are frequently observed in cancer cells due to dysregulation of kinases and phosphatases. Specific phosphorylation sites can be used as biomarkers.
2.7. Circulating Tumor Cells (CTCs): Although not strictly proteins, the analysis of proteins expressed by CTCs can provide valuable information about tumor characteristics and prognosis. CTCs are cancer cells that have detached from the primary tumor and are circulating in the bloodstream. The enumeration and characterization of CTCs provide a “liquid biopsy” that can be used to monitor treatment response, detect minimal residual disease, and predict prognosis.
2.8. Extracellular Vesicles (EVs): EVs, including exosomes and microvesicles, are membrane-bound vesicles released by cells that contain proteins, nucleic acids, and lipids. EVs derived from tumor cells can carry cancer-specific proteins and can be used as biomarkers. EVs are involved in cell-to-cell communication and can influence the tumor microenvironment, promoting cancer progression. The protein cargo of EVs can reflect the molecular profile of the originating tumor cells.
Many thanks to our sponsor Esdebe who helped us prepare this research report.
3. Analytical Methodologies for Protein Biomarker Detection
Various analytical techniques are used to detect and quantify protein biomarkers in biological samples. These methods can be broadly classified into:
3.1. Immunoassays: Immunoassays are based on the specific binding of antibodies to target proteins. Common immunoassay formats include:
- Enzyme-linked immunosorbent assay (ELISA): A widely used technique that involves immobilizing an antibody or antigen on a solid surface and detecting the binding of the target protein using an enzyme-linked antibody. ELISA is a sensitive and relatively inexpensive method, suitable for high-throughput screening.
- Western blotting: A technique used to separate proteins by size using gel electrophoresis and then detect the target protein using an antibody. Western blotting can provide information about protein size and abundance.
- Immunohistochemistry (IHC): A technique used to detect proteins in tissue samples using antibodies. IHC is a valuable tool for identifying protein expression patterns and localizing proteins within tissues.
- Flow cytometry: A technique used to analyze cells based on their size, granularity, and expression of specific proteins. Flow cytometry can be used to quantify the number of cells expressing a particular protein.
- Multiplexed immunoassays: These assays allow for the simultaneous detection and quantification of multiple proteins in a single sample. Examples include bead-based assays (e.g., Luminex) and multiplex ELISA platforms.
3.2. Mass Spectrometry (MS): MS is a powerful analytical technique that measures the mass-to-charge ratio of ions. MS-based proteomics approaches are used to identify and quantify proteins in complex biological samples.
- Bottom-up proteomics: This approach involves digesting proteins into peptides and then analyzing the peptides by MS. Bottom-up proteomics is the most widely used approach for protein identification and quantification.
- Top-down proteomics: This approach involves analyzing intact proteins by MS without prior digestion. Top-down proteomics can provide information about protein isoforms and post-translational modifications.
- Targeted proteomics: This approach involves selectively measuring the abundance of specific proteins using MS. Targeted proteomics is a highly sensitive and quantitative approach.
3.3. Affinity-Based Proteomics: This approach utilizes specific affinity reagents, such as antibodies or aptamers, to capture and enrich target proteins from complex samples, followed by detection using various methods, including mass spectrometry or fluorescence-based assays. Affinity-based proteomics offers high sensitivity and specificity for the detection of low-abundance proteins.
3.4. Aptamer-Based Assays: Aptamers are single-stranded DNA or RNA oligonucleotides that bind to specific target molecules with high affinity and specificity. Aptamer-based assays can be used to detect and quantify proteins in biological samples. Aptamers offer several advantages over antibodies, including easier production, higher stability, and lower cost.
The choice of analytical method depends on several factors, including the abundance of the target protein, the desired sensitivity and specificity, the sample type, and the availability of resources.
Many thanks to our sponsor Esdebe who helped us prepare this research report.
4. Challenges in Protein Biomarker Discovery and Validation
The development and implementation of protein biomarkers in cancer face several challenges, including:
4.1. Sensitivity and Specificity: A major challenge is achieving sufficient sensitivity and specificity to accurately detect cancer at an early stage and distinguish it from benign conditions. Many existing protein biomarkers lack the desired sensitivity and specificity, leading to false-positive and false-negative results. This is partly because many of these proteins are not exclusively expressed in cancer cells and can be elevated in other conditions as well. Furthermore, the heterogeneity of cancer can lead to variations in protein expression, making it difficult to identify biomarkers that are consistently elevated in all patients.
4.2. Protein Degradation and Stability: Proteins can degrade rapidly in biological samples, particularly in serum or plasma. This degradation can affect the accuracy of biomarker measurements. Several factors can contribute to protein degradation, including proteases, pH, and temperature. To minimize protein degradation, samples should be processed and stored properly. Furthermore, the use of protease inhibitors and other stabilizing agents can help to preserve protein integrity.
4.3. Inter-Individual Variability: Protein levels can vary significantly between individuals due to genetic factors, environmental influences, and lifestyle factors. This inter-individual variability can make it difficult to establish reliable cutoff values for biomarker measurements. To address this challenge, it is important to collect samples from a large and well-characterized cohort of patients. Furthermore, the use of personalized medicine approaches, which take into account individual patient characteristics, can help to improve the accuracy of biomarker measurements.
4.4. Lack of Standardization and Reproducibility: The lack of standardized assays and protocols can lead to inconsistencies in biomarker measurements between different laboratories. This can make it difficult to compare results from different studies and to implement biomarkers in clinical practice. To address this challenge, it is important to develop standardized assays and protocols that are validated across multiple laboratories. Furthermore, the use of certified reference materials can help to ensure the accuracy and reliability of biomarker measurements.
4.5. Complexity of the Proteome: The human proteome is incredibly complex, with thousands of different proteins and post-translational modifications. This complexity makes it challenging to identify and validate novel protein biomarkers. Furthermore, the abundance of many proteins is very low, making it difficult to detect them using conventional analytical techniques. To address this challenge, it is important to use high-sensitivity analytical techniques and to develop methods for enriching target proteins from complex samples.
4.6. Cost and Accessibility: The cost of developing and implementing protein biomarkers can be high, particularly for novel biomarkers that require extensive validation. Furthermore, many analytical techniques used for protein biomarker detection are not widely accessible, particularly in resource-limited settings. To address this challenge, it is important to develop cost-effective assays and to make analytical techniques more widely available.
Many thanks to our sponsor Esdebe who helped us prepare this research report.
5. Strategies to Overcome Challenges and Enhance Biomarker Performance
Several strategies are being developed to overcome the challenges associated with protein biomarker discovery and validation and to enhance biomarker performance:
5.1. Multiplexed Assays: Multiplexed assays allow for the simultaneous measurement of multiple proteins in a single sample. This approach can improve the sensitivity and specificity of cancer detection by combining information from multiple biomarkers. Furthermore, multiplexed assays can reduce the cost and time required for biomarker analysis.
5.2. Proteomics Technologies: Proteomics technologies, such as mass spectrometry, are being used to identify and quantify proteins in complex biological samples. These technologies can provide a comprehensive overview of the proteome and can be used to identify novel protein biomarkers. Furthermore, proteomics technologies can be used to study protein modifications, such as glycosylation and phosphorylation, which can provide valuable information about cancer development and progression.
5.3. Glycomics and Glycoproteomics: Cancer cells often exhibit altered glycosylation patterns. Glycomics and glycoproteomics approaches are being used to identify and characterize these changes and to develop novel glycan-based biomarkers. Glycosylation is a complex process, and altered glycosylation patterns can affect protein folding, stability, and interactions with other molecules.
5.4. Liquid Biopsies: Liquid biopsies, such as circulating tumor cells (CTCs) and extracellular vesicles (EVs), provide a non-invasive way to access tumor-derived material. These samples can be used to identify and quantify protein biomarkers and to monitor treatment response. Liquid biopsies offer several advantages over traditional tissue biopsies, including ease of collection and the ability to obtain serial samples over time.
5.5. Artificial Intelligence (AI) and Machine Learning (ML): AI and ML algorithms are being used to analyze large datasets of protein biomarker data and to identify patterns that can be used to improve cancer detection and prediction. AI and ML can be used to identify novel biomarker combinations, to predict treatment response, and to personalize cancer therapy.
5.6. Standardization and Validation Efforts: Initiatives are underway to standardize assays and protocols for protein biomarker detection and to validate biomarkers in large, well-characterized cohorts of patients. These efforts are essential for ensuring the accuracy and reliability of biomarker measurements and for facilitating the implementation of biomarkers in clinical practice.
5.7. Point-of-Care Testing: The development of point-of-care (POC) testing devices can enable rapid and convenient biomarker measurements at the point of care. POC testing can improve access to biomarker testing, particularly in resource-limited settings.
Many thanks to our sponsor Esdebe who helped us prepare this research report.
6. Clinical Applications of Protein Biomarkers in Cancer
Protein biomarkers have a wide range of clinical applications in cancer management, including:
6.1. Screening and Early Detection: Protein biomarkers can be used to screen individuals at high risk for cancer and to detect cancer at an early stage, when treatment is more likely to be successful. However, the limited sensitivity and specificity of many existing protein biomarkers have hampered their widespread use for screening. Novel biomarkers with improved performance are needed to improve cancer screening.
6.2. Diagnosis and Differential Diagnosis: Protein biomarkers can be used to diagnose cancer and to differentiate between different types of cancer. Biomarkers can also be used to distinguish cancer from benign conditions. Accurate diagnosis is essential for selecting the appropriate treatment strategy.
6.3. Prognosis and Risk Stratification: Protein biomarkers can be used to predict the prognosis of cancer patients and to stratify patients into different risk groups. Prognostic biomarkers can help to identify patients who are likely to benefit from more aggressive treatment and patients who may be able to avoid unnecessary treatment.
6.4. Prediction of Treatment Response: Protein biomarkers can be used to predict whether a patient is likely to respond to a particular treatment. Predictive biomarkers can help to guide treatment decisions and to avoid ineffective treatments. For example, EGFR mutations are predictive of response to EGFR tyrosine kinase inhibitors in non-small cell lung cancer.
6.5. Monitoring Treatment Response and Detecting Recurrence: Protein biomarkers can be used to monitor treatment response and to detect recurrence of cancer. Monitoring treatment response can help to identify patients who are not responding to treatment and who may need to switch to a different therapy. Detecting recurrence early can improve the chances of successful treatment.
6.6. Personalized Medicine: Protein biomarkers can be used to personalize cancer therapy by tailoring treatment decisions to the individual characteristics of each patient. Personalized medicine approaches can improve treatment outcomes and reduce side effects.
Many thanks to our sponsor Esdebe who helped us prepare this research report.
7. Future Directions and Concluding Remarks
The field of protein biomarkers in cancer is rapidly evolving. Future research should focus on the following areas:
- Discovery of novel biomarkers: There is a need for novel biomarkers with improved sensitivity and specificity for early cancer detection and prediction of treatment response.
- Development of multiplexed assays: Multiplexed assays can improve the performance of biomarker testing and reduce the cost and time required for analysis.
- Integration of multi-omics data: Integrating protein biomarker data with data from other omics platforms, such as genomics, transcriptomics, and metabolomics, can provide a more comprehensive understanding of cancer biology and improve biomarker performance.
- Validation of biomarkers in large, well-characterized cohorts: Rigorous validation of biomarkers in large, well-characterized cohorts is essential for ensuring the accuracy and reliability of biomarker measurements.
- Development of point-of-care testing devices: Point-of-care testing can improve access to biomarker testing, particularly in resource-limited settings.
- Implementation of biomarkers in clinical practice: Efforts are needed to translate validated biomarkers into clinical practice and to ensure that they are used appropriately.
Protein biomarkers hold immense promise for improving cancer management. By addressing the challenges associated with biomarker discovery, validation, and implementation, and by leveraging emerging technologies and strategies, we can accelerate the development and clinical application of protein biomarkers and ultimately improve patient outcomes.
Many thanks to our sponsor Esdebe who helped us prepare this research report.
References
- Hanash, S. M., Pitteri, S. J., & Faca, V. M. (2008). Mining the human proteome to create clinical tools for early cancer detection. Nature, 452(7187), 571-579.
- Henry, N. L., Hayes, D. F. (2012). Cancer biomarkers. Molecular Oncology, 6(2), 140–146.
- Diamandis, E. P. (2010). Cancer biomarkers: Past, present, and future. Clin Chem, 56(4), 583-587.
- Duffy, M. J. (2006). Role of tumour markers in patients with solid cancers: an overview. European Journal of Internal Medicine, 17(4), 247-252.
- Escobar, G., Mangino, J. L., Jabbour, M. N., & Cavalli, I. J. (2019). Circulating tumor cells: a clinical review. Revista da Associação Médica Brasileira, 65(1), 68-73.
- Théry, C., Witwer, K. W., Aikawa, E., Alcaraz, M. J., Anderson, J. D., Andriantsitohaina, R., … & Gho, Y. S. (2018). Minimal information for studies of extracellular vesicles 2018 (MISEV2018): a position statement of the International Society for Extracellular Vesicles and update of the MISEV2014 guidelines. Journal of extracellular vesicles, 7(1), 1535750.
- Villanueva, J., & Philipson, E. (2015). Clinical proteomics: current status and future directions. Clinical Chemistry, 61(1), 16-25.
- Levin, Y., Tal, N., & Scherz, A. (2014). Aptamers: versatile tools for diagnostics, targeted drug delivery and research. Journal of Nanobiotechnology, 12(1), 50.
- Sokoll, L. J., Chan, D. W. (2003). Proteomics in cancer diagnosis. Expert Review of Molecular Diagnostics, 3(6), 655-666.
- Rai, A. J., Gelfond, J. A., Hayek, O. R., Cote, R. J., & Hanash, S. M. (2005). Proteomic approaches to cancer biomarker discovery. Clin Chem, 51(11), 1900-1908.
- Paulovich, A. G., Billheimer, D., Hamamoto, D., Giedzinski, E., McIntosh, M., & Springler, E. (2005). Standardizing biomarkers for personalized cancer medicine. Disease Markers, 21(1), 39-46.
- Hortin, G. L., Sviridov, D., Anderson, N. L., & Greely, D. A. (2008). Standardizing mass spectrometry for clinical proteomics. Clinical Chemistry, 54(5), 789-797.
- Poste, G. (2011). Bring on the biomarkers. Nature, 469(7329), 156-157.
- Sawyers, C. L. (2004). The cancer biomarker problem. Nature, 432(7015), 368-369.
- Lisitsa, A. V., Poroikov, V. V., Popov, A. A., Archakov, A. I. (2010). Bioinformatics in biomarker discovery. Acta Naturae, 2(2), 28-39.
- Vasanthi, A., Adrianzen Herrera, D., Slieman, T., & Ali, A. (2024). Artificial intelligence-powered discovery of cancer biomarkers for personalized medicine. Frontiers in Digital Health, 6, 1356956.
- Wu, J., Song, F., Liu, X., & Li, M. (2022). A review on the application of point-of-care testing in oncology management. Frontiers in Molecular Biosciences, 9, 905337.
Extracellular vesicles carrying protein cargo sounds like tiny Trojan horses! If they’re anything like real horses, I wonder if we’ll need special biomarker “stable hands” to manage them and prevent any… ahem… unexpected deliveries in our clinical trials?
That’s a fantastic analogy! The idea of “stable hands” for extracellular vesicles is spot on. Standardized protocols are crucial for EV research to ensure consistent and reliable results, especially in clinical trials. Better characterization will help us prevent variability and enhance reproducibility, much like caring for those horses!
Editor: MedTechNews.Uk
Thank you to our Sponsor Esdebe
So, if these circulating tumor cells are detectable in liquid biopsies, why are we still poking around with needles when we could just ask for a vial? Seems a lot less medieval, no?
That’s a great point! The move toward liquid biopsies for circulating tumor cells is definitely gaining momentum, and for many good reasons. While liquid biopsies offer less invasive sampling, traditional biopsies can sometimes provide more detailed information about the tumor’s microenvironment and heterogeneity. As technology improves, perhaps the ‘needle’ will become less vital. Thanks for sparking the discussion!
Editor: MedTechNews.Uk
Thank you to our Sponsor Esdebe
The overview of glycosylation changes as cancer biomarkers is particularly insightful. Further research into specific glycan structures unique to cancer cells could greatly enhance diagnostic accuracy and therapeutic targeting.
I’m glad you found the glycosylation section insightful! Spotting unique glycan structures is definitely a promising avenue. Exploring how these structures influence cancer cell behavior and interaction with the immune system could lead to innovative therapies. Thanks for highlighting this important area!
Editor: MedTechNews.Uk
Thank you to our Sponsor Esdebe