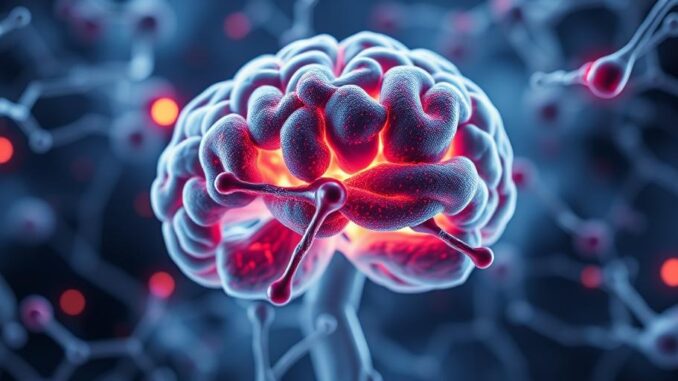
Abstract
Neurodegenerative diseases, including Alzheimer’s disease (AD), Parkinson’s disease (PD), Huntington’s disease (HD), and amyotrophic lateral sclerosis (ALS), are characterized by the progressive loss of neuronal function and the accumulation of misfolded protein aggregates. While amyloid-β (Aβ) and tau protein have been central to AD research, and α-synuclein to PD, a broader perspective on protein homeostasis (proteostasis) highlights the interconnectedness of various cellular processes involved in maintaining protein quality and the implications of proteostasis failure in disease pathogenesis. This review examines the multifaceted mechanisms of proteostasis, encompassing protein synthesis, folding, trafficking, and degradation, and explores their disruption in neurodegenerative diseases. We delve into the emerging roles of various proteins beyond the canonical disease markers, emphasizing the complex interplay of proteomic alterations. Furthermore, we discuss therapeutic strategies targeting proteostasis pathways as potential disease-modifying interventions.
Many thanks to our sponsor Esdebe who helped us prepare this research report.
1. Introduction
The central dogma of molecular biology emphasizes the importance of DNA as the blueprint for protein synthesis. However, the journey from a linear polypeptide chain to a functional protein is a complex and tightly regulated process. Maintaining a healthy proteome, characterized by properly folded and functional proteins, is crucial for cellular survival and function. This process, known as proteostasis, involves a network of interconnected pathways that govern protein synthesis, folding, trafficking, aggregation prevention, and degradation. Disruption of proteostasis, often triggered by aging, genetic mutations, or environmental stressors, can lead to the accumulation of misfolded and aggregated proteins, a hallmark of many neurodegenerative diseases.
While the focus of neurodegenerative disease research has traditionally centered on specific proteins, such as Aβ and tau in AD or α-synuclein in PD, a more holistic view recognizes that proteostasis collapse is a systemic phenomenon affecting multiple proteins and cellular compartments. Therefore, understanding the broader proteostasis network and its dysregulation in the context of neurodegenerative diseases is crucial for developing effective therapeutic strategies.
Many thanks to our sponsor Esdebe who helped us prepare this research report.
2. The Proteostasis Network: A Multifaceted System
The proteostasis network (PN) comprises several key components that work in concert to maintain protein quality control:
-
Protein Synthesis and Folding: The ribosome is responsible for translating mRNA into polypeptide chains. Nascent proteins must then fold into their correct three-dimensional structures to function properly. This process is often facilitated by molecular chaperones, such as heat shock proteins (HSPs), which prevent aggregation and assist in proper folding. The endoplasmic reticulum (ER) also plays a critical role in protein folding and quality control. Improperly folded proteins in the ER trigger the unfolded protein response (UPR), a signaling pathway that aims to restore ER homeostasis by increasing chaperone expression, reducing protein synthesis, and enhancing ER-associated degradation (ERAD).
-
Protein Trafficking and Localization: Once properly folded, proteins must be transported to their appropriate cellular compartments, such as the mitochondria, Golgi apparatus, or plasma membrane. This process involves specific trafficking signals and transport machinery. Misfolded proteins can be retained in the ER or directed to degradation pathways instead of being properly trafficked.
-
Protein Degradation Pathways: Cells possess two major protein degradation pathways: the ubiquitin-proteasome system (UPS) and autophagy. The UPS selectively degrades short-lived and misfolded proteins that have been tagged with ubiquitin. The proteasome, a large multi-subunit protease complex, recognizes and degrades ubiquitinated proteins. Autophagy, on the other hand, is a bulk degradation pathway that engulfs cytoplasmic components, including aggregated proteins and damaged organelles, in autophagosomes, which then fuse with lysosomes for degradation. Selective autophagy pathways, such as mitophagy (for mitochondria) and aggrephagy (for protein aggregates), target specific cargo for degradation.
Each of these components is interconnected and communicates to ensure that the protein pool remains healthy. For instance, impaired protein folding can trigger the UPR, which in turn can modulate autophagy. Similarly, UPS dysfunction can lead to the accumulation of misfolded proteins, triggering the formation of aggresomes and activating autophagy.
Many thanks to our sponsor Esdebe who helped us prepare this research report.
3. Proteostasis Collapse in Neurodegenerative Diseases
Neurodegenerative diseases are characterized by the accumulation of misfolded protein aggregates, suggesting a breakdown of the PN. While the specific proteins that aggregate vary between diseases (e.g., Aβ and tau in AD, α-synuclein in PD, huntingtin in HD, and TDP-43 and SOD1 in ALS), the underlying mechanisms of proteostasis failure often share common features. It is also increasingly apparent that many proteins other than the main drivers also accumulate in disease states.
-
Alzheimer’s Disease: In AD, Aβ plaques and neurofibrillary tangles composed of hyperphosphorylated tau protein are the pathological hallmarks. While these proteins are the primary focus of AD research, evidence suggests that other proteins, such as TDP-43, accumulate in a significant proportion of AD brains. Disruption of the UPS and autophagy has also been implicated in AD pathogenesis, contributing to the accumulation of both Aβ and tau. Furthermore, mutations in genes involved in endosomal trafficking, such as SORL1, are associated with increased AD risk, highlighting the importance of protein trafficking in disease development.
-
Parkinson’s Disease: PD is characterized by the loss of dopaminergic neurons in the substantia nigra and the presence of Lewy bodies, which are intracytoplasmic inclusions composed primarily of aggregated α-synuclein. Mutations in genes encoding α-synuclein (SNCA), ubiquitin ligases (e.g., PARK2, PINK1), and autophagy receptors (e.g., LRRK2) are associated with increased PD risk, further implicating proteostasis dysfunction in disease etiology. Dysregulation of the UPS and autophagy pathways contributes to the accumulation of α-synuclein aggregates, leading to neuronal dysfunction and cell death. Furthermore, impaired mitochondrial function and increased oxidative stress can exacerbate proteostasis failure in PD.
-
Huntington’s Disease: HD is caused by an expanded CAG repeat in the HTT gene, resulting in a mutant huntingtin protein with an abnormally long polyglutamine (polyQ) tract. This mutant huntingtin protein is prone to aggregation, forming intracellular inclusions. Impaired protein folding, decreased chaperone activity, and defects in the UPS and autophagy pathways contribute to the accumulation of mutant huntingtin aggregates. The presence of polyQ repeats disrupts normal protein structure and interferes with the function of the proteasome.
-
Amyotrophic Lateral Sclerosis: ALS is a progressive motor neuron disease characterized by the loss of motor neurons in the brain and spinal cord. Mutations in several genes, including SOD1, TDP-43, FUS, and C9orf72, have been linked to ALS. These proteins are involved in various cellular processes, including RNA processing, DNA repair, and proteostasis. TDP-43 and FUS are RNA-binding proteins that can mislocalize and aggregate in the cytoplasm, disrupting RNA metabolism and contributing to neuronal dysfunction. Mutations in SOD1 lead to the production of a misfolded protein that is prone to aggregation, disrupting mitochondrial function and activating apoptotic pathways. The C9orf72 repeat expansion leads to the production of toxic RNA transcripts and dipeptide repeat proteins, which can disrupt protein trafficking and proteasome function.
It’s crucial to recognize that the specific proteins accumulating in each disease are not acting in isolation. These misfolded proteins can impair the general proteostasis environment. For example, a buildup of misfolded tau in AD can disrupt the UPS and autophagy pathways, indirectly affecting the clearance of other proteins and further exacerbating cellular dysfunction. Similarly, the accumulation of α-synuclein in PD can disrupt mitochondrial function, leading to increased oxidative stress and further compromising proteostasis. This interconnectedness highlights the complexity of proteostasis failure in neurodegenerative diseases and emphasizes the need for therapeutic strategies that target the broader proteostasis network.
Many thanks to our sponsor Esdebe who helped us prepare this research report.
4. Emerging Roles of Proteins Beyond Canonical Disease Markers
While traditionally research has focused on the “usual suspects” in each neurodegenerative disease, a growing body of evidence suggests that a multitude of other proteins are involved in disease pathogenesis. This “proteomic convergence” reveals a shared vulnerability across different neurodegenerative conditions and offers new therapeutic targets. Examples include:
-
TDP-43: While primarily associated with ALS and frontotemporal lobar degeneration (FTLD), TDP-43 pathology is also observed in a significant proportion of AD and other neurodegenerative diseases. TDP-43 is an RNA-binding protein involved in various aspects of RNA processing. Misfolded TDP-43 can mislocalize to the cytoplasm, form aggregates, and disrupt RNA metabolism. Its presence in multiple neurodegenerative diseases suggests a fundamental role in neuronal vulnerability.
-
FUS: Similar to TDP-43, FUS is an RNA-binding protein primarily associated with ALS and FTLD. Mutations in FUS can lead to its mislocalization and aggregation, disrupting RNA metabolism. FUS pathology has also been observed in other neurodegenerative diseases, highlighting its potential role in broader proteostasis dysfunction.
-
p62/SQSTM1: p62 is an autophagy receptor that recognizes and delivers ubiquitinated proteins to autophagosomes for degradation. Accumulation of p62-positive inclusions is a common feature of many neurodegenerative diseases, indicating impaired autophagy. Mutations in SQSTM1, the gene encoding p62, have been linked to PD and ALS, further emphasizing the importance of autophagy in neuroprotection.
-
Heat Shock Proteins (HSPs): HSPs are molecular chaperones that assist in protein folding and prevent aggregation. Dysregulation of HSP expression and function has been observed in various neurodegenerative diseases. Upregulating HSP expression can promote protein folding and clearance, potentially mitigating the effects of proteostasis failure. Studies have shown that pharmacological activation of HSPs can improve protein homeostasis and reduce neurodegeneration in animal models.
-
Ubiquitin Ligases: The ubiquitin-proteasome system (UPS) plays a critical role in protein degradation and maintaining proteostasis. Several ubiquitin ligases, such as Parkin, CHIP, and TRIM11, have been implicated in neurodegenerative diseases. Loss of function mutations or decreased expression of these ligases can impair the clearance of misfolded proteins and exacerbate neurodegeneration. Enhancing the activity of specific ubiquitin ligases could be a promising therapeutic strategy.
This is not an exhaustive list, and proteomic studies are continually identifying novel proteins involved in neurodegenerative disease pathogenesis. These discoveries emphasize the complexity of these disorders and the need to consider the broader proteomic context when developing therapeutic interventions.
Many thanks to our sponsor Esdebe who helped us prepare this research report.
5. The Role of Post-Translational Modifications (PTMs)
Post-translational modifications (PTMs) are chemical modifications that occur on proteins after their translation from mRNA. These modifications can significantly alter protein structure, function, and interactions. PTMs play a crucial role in regulating proteostasis and can be dysregulated in neurodegenerative diseases.
-
Phosphorylation: Phosphorylation is the addition of a phosphate group to a protein, typically on serine, threonine, or tyrosine residues. Phosphorylation can alter protein activity, stability, and interactions. Hyperphosphorylation of tau protein is a hallmark of AD, leading to its detachment from microtubules and aggregation into neurofibrillary tangles. Aberrant phosphorylation of other proteins has also been implicated in neurodegenerative diseases, disrupting various cellular processes.
-
Ubiquitination: Ubiquitination is the attachment of ubiquitin, a small regulatory protein, to a target protein. Ubiquitination can serve as a signal for protein degradation via the UPS or as a modulator of protein function and interactions. Dysregulation of ubiquitination has been implicated in various neurodegenerative diseases, impairing the clearance of misfolded proteins.
-
Acetylation: Acetylation is the addition of an acetyl group to a protein, typically on lysine residues. Acetylation can alter protein-DNA interactions, protein-protein interactions, and protein stability. Dysregulation of acetylation has been implicated in neurodegenerative diseases, affecting gene expression and protein homeostasis.
-
Glycosylation: Glycosylation is the addition of a sugar moiety to a protein. Glycosylation can affect protein folding, stability, and trafficking. Aberrant glycosylation has been observed in neurodegenerative diseases, potentially disrupting protein function and clearance.
-
SUMOylation: SUMOylation is the covalent attachment of a small ubiquitin-like modifier (SUMO) protein to a target protein. SUMOylation can affect protein localization, interactions, and stability. Dysregulation of SUMOylation has been linked to neurodegenerative diseases, influencing the aggregation propensity of various proteins.
Understanding the role of PTMs in proteostasis regulation is crucial for developing therapeutic strategies that target these modifications. For example, inhibiting kinases that phosphorylate tau protein could reduce tau aggregation in AD. Similarly, enhancing the activity of deubiquitinases could promote the clearance of misfolded proteins.
Many thanks to our sponsor Esdebe who helped us prepare this research report.
6. Therapeutic Strategies Targeting Proteostasis
Given the central role of proteostasis failure in neurodegenerative diseases, therapeutic strategies aimed at restoring proteostasis are gaining increasing attention. These strategies can target various aspects of the PN, including protein folding, degradation, and aggregation prevention.
-
Chaperone-based Therapies: Enhancing chaperone activity can promote protein folding, prevent aggregation, and facilitate the clearance of misfolded proteins. Several small molecules that activate HSPs are under development for the treatment of neurodegenerative diseases. These molecules can increase the expression of HSPs, leading to improved protein homeostasis and reduced neurodegeneration.
-
UPS Enhancement: Promoting the activity of the UPS can enhance the degradation of misfolded proteins. Certain compounds, such as proteasome activators, are being investigated as potential therapeutic agents for neurodegenerative diseases. However, it is important to note that excessive proteasome activity can be detrimental, so careful titration and target specificity are critical.
-
Autophagy Enhancement: Stimulating autophagy can promote the clearance of aggregated proteins and damaged organelles. Several compounds, such as rapamycin and its analogs (rapalogs), are known to induce autophagy. However, long-term rapamycin treatment can have side effects, so alternative autophagy-inducing strategies are being explored.
-
Aggregation Inhibitors: Preventing protein aggregation can reduce the formation of toxic oligomers and inclusions. Several small molecules that bind to specific proteins and inhibit their aggregation are under development for the treatment of neurodegenerative diseases. These molecules can stabilize the native protein conformation, preventing misfolding and aggregation.
-
Clearance-Enhancing Antibodies: Immunotherapy approaches, using antibodies to target misfolded or aggregated proteins, are showing promise in preclinical and clinical studies. These antibodies can promote the clearance of toxic protein species by activating microglial phagocytosis or by preventing seeding and spread of pathological proteins. Antibodies against amyloid-β and tau are currently being evaluated in clinical trials for AD.
-
Gene Therapy: Gene therapy approaches can be used to deliver genes encoding molecular chaperones, ubiquitin ligases, or autophagy receptors to the brain. This can enhance the expression of these proteins and restore proteostasis in affected neurons.
-
Small Molecule Inducers of Chaperone-Mediated Autophagy (CMA): CMA is a selective autophagy pathway that degrades proteins containing a KFERQ-like motif. Enhancing CMA activity can promote the clearance of specific proteins. Small molecules that activate CMA are being explored as potential therapeutic agents for neurodegenerative diseases. However, its relative contribution to neuroprotection versus other autophagy pathways is an area of ongoing research.
It is important to note that many of these therapeutic strategies are still in early stages of development. Clinical trials are needed to determine their efficacy and safety in humans. Furthermore, a combination of therapeutic approaches that target multiple aspects of the PN may be necessary to achieve optimal results.
Many thanks to our sponsor Esdebe who helped us prepare this research report.
7. Challenges and Future Directions
Despite significant advances in our understanding of proteostasis and its role in neurodegenerative diseases, several challenges remain. These include:
-
Complexity of the PN: The PN is a complex network of interconnected pathways, making it difficult to target specific components without affecting others. Developing highly selective and targeted therapies is crucial for minimizing side effects.
-
Brain Penetration: Many potential therapeutic agents have difficulty crossing the blood-brain barrier (BBB), limiting their efficacy in treating neurodegenerative diseases. Developing strategies to enhance drug delivery to the brain is essential.
-
Disease Heterogeneity: Neurodegenerative diseases are heterogeneous, with different patients exhibiting different clinical presentations and underlying pathologies. Identifying biomarkers to stratify patients and tailor therapies to individual needs is crucial.
-
Early Intervention: Neurodegenerative diseases often progress for many years before clinical symptoms become apparent. Early intervention, before significant neuronal damage has occurred, may be necessary to achieve disease modification. Developing sensitive and specific biomarkers to detect early disease stages is essential.
-
Off-Target Effects: Many therapeutic agents can have off-target effects, leading to unwanted side effects. Careful screening and optimization of drug candidates are crucial for minimizing off-target effects.
Future research should focus on: a) Elucidating the detailed mechanisms of proteostasis regulation in different brain regions and cell types. b) Identifying novel therapeutic targets within the PN. c) Developing more effective strategies for drug delivery to the brain. d) Developing biomarkers for early disease detection and patient stratification. e) Conducting clinical trials to evaluate the efficacy and safety of proteostasis-targeting therapies.
Many thanks to our sponsor Esdebe who helped us prepare this research report.
8. Conclusion
Maintaining protein homeostasis is essential for neuronal survival and function. Disruption of the proteostasis network is a common feature of neurodegenerative diseases, leading to the accumulation of misfolded protein aggregates and neuronal dysfunction. While amyloid-β and tau have been central to AD research, a broader perspective on proteostasis highlights the interconnectedness of various cellular processes involved in maintaining protein quality and the implications of proteostasis failure in disease pathogenesis. Therapeutic strategies targeting proteostasis pathways hold great promise for the treatment of neurodegenerative diseases. Future research should focus on elucidating the detailed mechanisms of proteostasis regulation and developing more effective and targeted therapies. By targeting the underlying causes of proteostasis failure, we may be able to prevent or delay the onset of neurodegenerative diseases and improve the lives of millions of people affected by these devastating disorders.
Many thanks to our sponsor Esdebe who helped us prepare this research report.
References
- Hipp, M. S., Park, S. H., & Hartl, F. U. (2014). Proteostasis impairment in protein-misfolding and -aggregation diseases. Trends in Cell Biology, 24(1), 22-33.
- Labbadia, J., & Morimoto, R. I. (2015). The biology of proteostasis in aging and disease. Annual Review of Biochemistry, 84, 435-464.
- Powers, E. T., Morimoto, R. I., Dillin, A., Kelly, J. W., & Balch, W. E. (2009). Protein folding in disease: a global perspective. Science, 326(5959), 1467-1474.
- Vendruscolo, M., & Knowles, T. P. J. (2016). Towards a quantitative understanding of protein aggregation in neurodegenerative diseases. Nature Reviews Molecular Cell Biology, 17(7), 397-411.
- Yerbury, J. J., Ooi, L., & Blair, I. P. (2013). The pathogenic role of protein aggregation in amyotrophic lateral sclerosis. Nature Reviews Neurology, 9(6), 269-281.
- Sweeney, P., Park, H., Baumann, T., Dunlop, D., Frydman, J., & Kopito, R. (2017). Protein disaggregation by cooperative Hsp70-Hsp110 chaperones. Nature, 550(7674), 254-258.
- Rubinsztein, D. C., Mariño, G., & Kroemer, G. (2011). Autophagy and ageing. Cell, 146(5), 682-695.
- Komatsu, M., Waguri, S., Ueno, T., Iwata, J., Murata, S., Tanida, I., … & Tanaka, K. (2006). Loss of autophagy in the central nervous system causes neurodegeneration in mice. Nature, 441(7095), 880-884.
- Moore, D. J., West, A. B., Dawson, V. L., & Dawson, T. M. (2005). Molecular pathophysiology of Parkinson’s disease. Annual Review of Neuroscience, 28, 57-87.
- Ciechanover, A. (2005). The ubiquitin-proteasome proteolytic pathway: from a vague idea to human biology and medicine. EMBO reports, 6 Suppl 1, S2-S5.
- Soto, C. (2003). Unfolding the role of protein misfolding in neurodegenerative diseases. Nature Reviews Neuroscience, 4(7), 491-502.
- Selkoe, D. J. (2001). Alzheimer’s disease: genes, proteins, and therapy. Physiological Reviews, 81(2), 741-766.
- Forman, M. S., Trojanowski, J. Q., & Lee, V. M. Y. (2004). Neurodegenerative diseases: a decade of discoveries paves the way for therapeutic breakthroughs. Nature Medicine, 10(10 Suppl), S81-S88.
- De Strooper, B., & Karran, E. (2016). The cellular phase of Alzheimer’s disease. Cell, 164(4), 603-615.
- Cook, C., Stetler, C., Petrucelli, L., & Dickson, D. (2024) TDP-43 pathology in neurodegenerative disease. Brain Research, 1828, 148731.
- Vance, C., Rogelj, B., Hortobágyi, T., De Vos, K. J., Nishimura, A. L., Sreedharan, J., … & Shaw, C. E. (2009). Mutations in FUS, an RNA processing protein, cause familial amyotrophic lateral sclerosis type 6. Science, 323(5918), 1208-1211.
- Menzies, F. M., Fleming, A., Carassiti, D., Bento, C., Roubille, L., Bassermann, F., … & Rubinsztein, D. C. (2015). Autophagy and neurodegeneration: pathogenic mechanisms and therapeutic opportunities. Neuron, 88(1), 34-48.
- Stefani, M., & Dobson, C. M. (2003). Protein aggregation and neurodegenerative diseases. Journal of Molecular Biology, 307(4), 761-768.
- Muchowski, P. J., & Wacker, J. L. (2005). Modulation of neurodegeneration by molecular chaperones. Nature Reviews Neuroscience, 6(1), 11-22.
- Hipp, M. S., Patel, C. N., Bersuker, K., Hayakawa, H., Varadarajan, S., Gupta, S., … & Grutzendler, J. (2019). Targeted degradation of mutant huntingtin fragments attenuates aggregation and cell death in Huntington’s disease models. Neuron, 104(1), 119-133.e6.
- Petrucelli, L., Dickson, D., Kehoe, K., Taylor, J., Greenhouse, G., Fancett, K., … & Yen, S. H. (2004). CHIP and Hsp70 regulate tau ubiquitination, degradation and aggregation. Human Molecular Genetics, 13(7), 703-714.
- Liscic, R. M., Grishkovskaya, I., Dignam, M., Pountney, D. L., & Yerbury, J. J. (2018). Proteostasis impairment in ALS: therapeutic implications. Neurotherapeutics, 15(1), 43-61.
- Loerch, P., & Cregan, S. P. (2015). Mechanisms of protein aggregation in neurodegenerative diseases. Journal of Neurochemistry, 134(5), 803-829.
- Wang, J., & Mandelkow, E. (2016). Tau in physiology and pathology. Nature Reviews Neuroscience, 17(1), 5-21.
- Soto, C., & Pritzkow, S. (2018). Protein misfolding, aggregation, and conformational strains in neurodegenerative diseases. Biochemical and Biophysical Research Communications, 497(1), 53-66.
The discussion around post-translational modifications is insightful. Investigating how specific PTMs act as “gatekeepers” within proteostasis networks could reveal novel therapeutic targets, particularly in modulating protein aggregation and clearance in early disease stages.
Thanks for highlighting the importance of post-translational modifications! Exploring how PTMs regulate protein interactions within proteostasis networks is a key area. Understanding these mechanisms may unlock new avenues for targeted therapies and personalized interventions, especially in early disease intervention. Your point about “gatekeepers” is especially thought provoking. What specific PTMs do you find most compelling?
Editor: MedTechNews.Uk
Thank you to our Sponsor Esdebe
This is a comprehensive review of proteostasis and its relevance to neurodegenerative diseases. I am intrigued by the discussion of emerging proteins beyond the canonical markers, such as TDP-43 and FUS. How does the interplay between these proteins and known genetic mutations influence disease progression and potential therapeutic interventions?