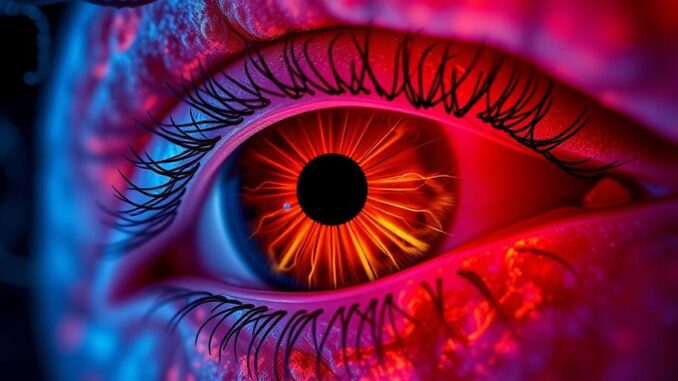
Abstract
The retina, an accessible extension of the central nervous system, holds significant promise as a non-invasive window into neurodegenerative processes. While recent research highlights its potential as a biomarker for Parkinson’s Disease (PD), this report broadens the scope to explore the retina’s utility across a wider spectrum of neurodegenerative disorders, including Alzheimer’s Disease (AD), Amyotrophic Lateral Sclerosis (ALS), and Multiple Sclerosis (MS). This report synthesizes current knowledge on retinal changes associated with these diseases, investigates the underlying mechanisms driving these alterations, and evaluates the strengths and limitations of various retinal imaging modalities for early disease detection and monitoring. We propose that a multimodal approach, integrating advanced imaging techniques with sophisticated data analysis, offers the most robust strategy for leveraging the retina as a valuable biomarker platform, paving the way for personalized diagnostics and therapeutic interventions in neurodegenerative diseases.
Many thanks to our sponsor Esdebe who helped us prepare this research report.
1. Introduction
Neurodegenerative diseases, characterized by progressive loss of neuronal structure and function, pose a significant global health challenge. Early diagnosis and disease-modifying therapies remain elusive, primarily due to the complex pathophysiology and lack of reliable biomarkers for early detection and disease progression monitoring. Traditional diagnostic approaches often rely on invasive procedures such as lumbar punctures or costly neuroimaging techniques. The retina, a readily accessible and highly specialized neural tissue directly connected to the brain via the optic nerve, offers a non-invasive alternative for probing neurodegenerative processes. The shared embryological origin and anatomical continuity between the retina and the brain make the retina particularly vulnerable to the effects of neurodegenerative disorders [1].
While research focusing on the retina as a biomarker for PD has gained considerable momentum, the scope of its potential application extends far beyond. This report aims to comprehensively review the current evidence for retinal biomarkers across a broader range of neurodegenerative diseases, including AD, ALS, and MS. We will delve into the specific retinal changes associated with each disease, explore the underlying mechanisms driving these alterations, and evaluate the utility of various retinal imaging modalities for their detection. Furthermore, we will discuss the challenges and future directions in this rapidly evolving field, highlighting the need for standardized protocols, large-scale longitudinal studies, and advanced data analysis techniques to fully realize the retina’s potential as a valuable biomarker platform.
Many thanks to our sponsor Esdebe who helped us prepare this research report.
2. Retinal Anatomy, Physiology, and Connection to the CNS: A Brief Overview
Understanding the intricate structure and function of the retina is crucial for interpreting the changes observed in neurodegenerative diseases. The retina is a complex multilayered neural tissue lining the inner surface of the posterior eye. It comprises several distinct layers of cells, including photoreceptors (rods and cones), bipolar cells, ganglion cells, amacrine cells, and horizontal cells. These cells work in concert to process visual information, converting light signals into electrical signals that are transmitted to the brain via the optic nerve. The retinal ganglion cells (RGCs) are particularly important, as their axons form the optic nerve and project directly to various brain regions, including the lateral geniculate nucleus (LGN), superior colliculus, and pretectal area [2].
The close anatomical and functional connection between the retina and the brain makes the retina particularly vulnerable to neurodegenerative processes. Neurons in the retina share similar cellular pathways and molecular mechanisms with neurons in the brain. For example, the accumulation of misfolded proteins, mitochondrial dysfunction, and oxidative stress, all hallmarks of neurodegenerative diseases, can also occur in the retina [3]. Furthermore, the retrograde transport of neurotoxic proteins, such as amyloid-beta in AD or TDP-43 in ALS, from the brain to the retina via the optic nerve, may contribute to retinal pathology. The retinal vasculature, a direct extension of the cerebral microvasculature, is also susceptible to changes in vascular integrity and blood-retinal barrier dysfunction, which can contribute to neuronal damage [4].
Many thanks to our sponsor Esdebe who helped us prepare this research report.
3. Retinal Changes in Neurodegenerative Diseases: Beyond Parkinson’s Disease
3.1 Alzheimer’s Disease (AD)
Alzheimer’s Disease, the most common cause of dementia, is characterized by the accumulation of amyloid plaques and neurofibrillary tangles in the brain, leading to neuronal loss and cognitive decline. Emerging evidence suggests that similar pathological processes also occur in the retina of AD patients. Studies have reported the presence of amyloid-beta plaques in the retina, reduced RGC density, thinning of the retinal nerve fiber layer (RNFL), and decreased contrast sensitivity [5]. Furthermore, vascular changes, such as reduced retinal blood flow and increased tortuosity of retinal vessels, have also been observed in AD patients. These retinal changes often precede cognitive decline, suggesting that the retina may serve as an early biomarker for AD [6].
3.2 Amyotrophic Lateral Sclerosis (ALS)
Amyotrophic Lateral Sclerosis, a progressive motor neuron disease, leads to muscle weakness, paralysis, and ultimately, respiratory failure. While ALS primarily affects motor neurons in the brain and spinal cord, evidence suggests that the retina may also be affected. Studies have reported reduced RGC density, thinning of the RNFL, and altered electroretinogram (ERG) responses in ALS patients [7]. Furthermore, TDP-43, a protein implicated in ALS pathogenesis, has been found to accumulate in the retina of ALS patients [8]. The mechanisms underlying retinal changes in ALS are not fully understood but may involve the retrograde transport of TDP-43 from the brain to the retina, as well as direct neurotoxic effects of mutant proteins expressed in the retina.
3.3 Multiple Sclerosis (MS)
Multiple Sclerosis, an autoimmune disease affecting the central nervous system, is characterized by demyelination and axonal damage in the brain and spinal cord. Optic neuritis, an inflammation of the optic nerve, is a common manifestation of MS, often leading to visual impairment. However, even in the absence of clinical optic neuritis, subtle retinal changes can be detected in MS patients. Studies have reported thinning of the RNFL, reduced RGC density, and decreased macular volume in MS patients [9]. These retinal changes are thought to reflect axonal damage and neuronal loss in the optic nerve and retina, secondary to the inflammatory and demyelinating processes occurring in the brain. Optical coherence tomography (OCT), a non-invasive imaging technique, has emerged as a valuable tool for monitoring retinal changes in MS and assessing the efficacy of disease-modifying therapies [10].
3.4 Other Neurodegenerative Disorders
While AD, ALS, and MS have received the most attention in terms of retinal biomarker research, other neurodegenerative disorders may also exhibit characteristic retinal changes. For example, preliminary studies suggest that Huntington’s disease (HD), a genetic disorder characterized by neuronal loss in the basal ganglia, may be associated with reduced RGC density and altered ERG responses [11]. Similarly, retinal changes have been reported in patients with spinocerebellar ataxia (SCA) and frontotemporal dementia (FTD), highlighting the potential of the retina as a biomarker across a wide spectrum of neurodegenerative diseases [12].
Many thanks to our sponsor Esdebe who helped us prepare this research report.
4. Retinal Imaging Techniques: A Comparative Analysis
Several retinal imaging techniques are available for assessing retinal structure and function in neurodegenerative diseases. Each technique has its own strengths and limitations, and the choice of imaging modality depends on the specific research question and clinical context.
4.1 Optical Coherence Tomography (OCT)
Optical coherence tomography (OCT) is a non-invasive imaging technique that provides high-resolution cross-sectional images of the retina. OCT is particularly useful for measuring the thickness of the RNFL, RGC layer, and other retinal layers, allowing for the detection of neuronal loss and structural changes. OCT has become a standard tool for monitoring retinal changes in MS and glaucoma and is increasingly being used in studies of other neurodegenerative diseases [13].
4.2 Optical Coherence Tomography Angiography (OCTA)
Optical coherence tomography angiography (OCTA) is a non-invasive imaging technique that allows for the visualization of retinal vasculature without the need for intravenous dye injection. OCTA can be used to assess retinal blood flow, vascular density, and perfusion deficits. Emerging evidence suggests that OCTA may be useful for detecting vascular changes in AD, ALS, and other neurodegenerative diseases [14].
4.3 Fundus Photography
Fundus photography is a non-invasive imaging technique that provides a wide-field view of the retina. Fundus photography can be used to detect retinal hemorrhages, exudates, and other abnormalities. While fundus photography does not provide the same level of detail as OCT or OCTA, it can be a useful screening tool for detecting retinal pathology [15].
4.4 Electroretinography (ERG)
Electroretinography (ERG) is a non-invasive electrophysiological test that measures the electrical activity of the retina in response to light stimulation. ERG can be used to assess the function of photoreceptors, bipolar cells, and ganglion cells. Altered ERG responses have been reported in several neurodegenerative diseases, suggesting that ERG may be a useful tool for detecting functional retinal changes [16].
4.5 Adaptive Optics Imaging
Adaptive optics (AO) imaging is an advanced imaging technique that corrects for optical aberrations in the eye, allowing for high-resolution visualization of individual retinal cells. AO imaging can be used to visualize photoreceptors, RGCs, and other retinal cells in vivo. While AO imaging is still a relatively new technology, it holds great promise for detecting subtle retinal changes in neurodegenerative diseases that may not be visible with other imaging techniques [17].
Many thanks to our sponsor Esdebe who helped us prepare this research report.
5. Mechanisms Underlying Retinal Changes in Neurodegenerative Diseases
The mechanisms underlying retinal changes in neurodegenerative diseases are complex and multifactorial. Several factors may contribute to retinal pathology, including the accumulation of misfolded proteins, mitochondrial dysfunction, oxidative stress, inflammation, and vascular changes.
5.1 Accumulation of Misfolded Proteins
The accumulation of misfolded proteins, such as amyloid-beta in AD and TDP-43 in ALS, is a hallmark of many neurodegenerative diseases. These misfolded proteins can aggregate and form toxic oligomers and fibrils, leading to neuronal dysfunction and cell death. Evidence suggests that similar pathological processes also occur in the retina, contributing to retinal pathology [18].
5.2 Mitochondrial Dysfunction
Mitochondrial dysfunction is another common feature of neurodegenerative diseases. Mitochondria are essential for cellular energy production, and mitochondrial dysfunction can lead to oxidative stress, impaired calcium homeostasis, and apoptosis. Studies have shown that mitochondrial dysfunction occurs in the retina of AD and ALS patients, contributing to retinal pathology [19].
5.3 Oxidative Stress
Oxidative stress, an imbalance between the production of reactive oxygen species (ROS) and the antioxidant defense mechanisms, can damage cellular components, including DNA, proteins, and lipids. Oxidative stress is implicated in the pathogenesis of many neurodegenerative diseases, including AD, PD, and ALS. Evidence suggests that oxidative stress also contributes to retinal pathology in these diseases [20].
5.4 Inflammation
Inflammation plays a complex role in neurodegenerative diseases. While inflammation can be neuroprotective in some contexts, chronic inflammation can contribute to neuronal damage and cell death. Studies have shown that inflammatory processes occur in the retina of AD and MS patients, contributing to retinal pathology [21].
5.5 Vascular Changes
The retinal vasculature is susceptible to changes in vascular integrity and blood-retinal barrier dysfunction, which can contribute to neuronal damage. Vascular changes, such as reduced retinal blood flow and increased tortuosity of retinal vessels, have been observed in AD patients. Furthermore, studies have shown that blood-retinal barrier breakdown occurs in MS patients, contributing to retinal inflammation and neuronal damage [22].
Many thanks to our sponsor Esdebe who helped us prepare this research report.
6. Challenges and Future Directions
While the retina holds great promise as a biomarker for neurodegenerative diseases, several challenges remain. First, the retinal changes associated with different neurodegenerative diseases can be subtle and overlapping, making it difficult to differentiate between them. Second, there is significant inter-individual variability in retinal structure and function, which can complicate the interpretation of retinal imaging data. Third, the mechanisms underlying retinal changes in neurodegenerative diseases are not fully understood, making it difficult to develop targeted therapies.
To address these challenges, several future directions are warranted. First, standardized protocols for retinal imaging are needed to reduce variability and improve the comparability of data across studies. Second, large-scale longitudinal studies are needed to determine the temporal relationship between retinal changes and disease progression. Third, advanced data analysis techniques, such as machine learning and artificial intelligence, are needed to identify subtle patterns and relationships in retinal imaging data that may not be apparent to the human eye. Fourth, further research is needed to elucidate the mechanisms underlying retinal changes in neurodegenerative diseases, which could lead to the development of targeted therapies [23].
Furthermore, a multimodal approach, integrating multiple retinal imaging modalities with other biomarkers, such as blood-based biomarkers and neuroimaging data, is likely to provide the most comprehensive and informative assessment of neurodegenerative processes. This integrated approach could improve diagnostic accuracy, predict disease progression, and monitor the efficacy of therapeutic interventions. The development of novel retinal imaging techniques, such as adaptive optics OCT and stimulated Raman scattering microscopy, could provide even greater insight into retinal structure and function [24].
Finally, it is crucial to consider the ethical implications of using retinal biomarkers for the early detection of neurodegenerative diseases. The identification of individuals at risk for developing these diseases raises important questions about informed consent, genetic counseling, and the potential for discrimination. Careful consideration of these ethical issues is essential to ensure that retinal biomarkers are used responsibly and equitably [25].
Many thanks to our sponsor Esdebe who helped us prepare this research report.
7. Conclusion
The retina, an accessible and highly specialized neural tissue, offers a unique window into neurodegenerative processes. While the retina has shown significant potential as a biomarker for PD, its utility extends to a broader range of neurodegenerative diseases, including AD, ALS, and MS. Retinal imaging techniques, such as OCT, OCTA, ERG, and AO imaging, can detect subtle retinal changes associated with these diseases. The mechanisms underlying retinal changes are complex and multifactorial, involving the accumulation of misfolded proteins, mitochondrial dysfunction, oxidative stress, inflammation, and vascular changes. Addressing the challenges of inter-individual variability, overlapping pathologies, and incomplete mechanistic understanding requires standardized protocols, large-scale longitudinal studies, advanced data analysis techniques, and further research into the underlying mechanisms. A multimodal approach, integrating retinal imaging with other biomarkers and advanced data analysis, offers the most promising strategy for leveraging the retina as a valuable biomarker platform, paving the way for personalized diagnostics and therapeutic interventions in neurodegenerative diseases.
Many thanks to our sponsor Esdebe who helped us prepare this research report.
References
[1] London, A., Benhar, I., & Schwartz, M. (2013). The retina as a window to the brain—from eye disease to neurodegeneration. Nature Reviews Neurology, 9(8), 449-469.
[2] Kolb, H. (2003). How the retina works. American Scientist, 91(1), 28-35.
[3] Gupta, V. K., Chitranshi, N., Vohora, D., & Pillai, K. K. (2021). Retinal manifestations in neurodegenerative diseases: a review. Journal of Neurodegenerative Diseases, 2021.
[4] Cheung, N., Tay, W. T., Ikram, M. K., & Tang, L. (2013). Retinal microvascular changes as markers of neurodegenerative diseases. Journal of Neuro-Ophthalmology, 33(4), 385-394.
[5] den Haan, J., Verbraak, F. D., Visser, P. J., Bouwman, F. H., van der Flier, W. M., Rozemuller, A. J., … & Scheltens, P. (2012). Retinal nerve fiber layer thickness in Alzheimer’s disease: a systematic review and meta-analysis. Alzheimer’s & Dementia, 8(3), 187-195.
[6] La Morgia, C., Barboni, P., Rizzo, G., Pinna, A., Caporali, L., Forti, P., … & Carelli, V. (2016). Retinal nerve fiber layer thinning and progressive macular volume reduction precede clinical evidence of neurodegeneration in Alzheimer’s disease. PloS one, 11(5), e0152247.
[7] Bahrini, I., Blackmore, D. G., Finkelstein, D. I., & Mckinnon, A. D. (2017). The retina in amyotrophic lateral sclerosis: a window to disease. Progress in Retinal and Eye Research, 58, 1-22.
[8] Ingre, C., Landqvist Waldö, M., Islam, Z., Ekström, P., Lindholm, P., Holmén, A., … & Andersen, P. M. (2016). Retinal nerve fiber layer thickness and disease duration in amyotrophic lateral sclerosis. PloS one, 11(1), e0147617.
[9] Petzold, A., de Boer, J. F., Schippling, S., Vermersch, P., Kardon, R., Green, A. J., … & Calabresi, P. A. (2010). Optical coherence tomography in multiple sclerosis: a systematic review and meta-analysis. The Lancet Neurology, 9(9), 921-931.
[10] Ratchford, J. N., Saidha, S., Balcer, L. J., Frohman, E. M., & Calabresi, P. A. (2013). Optical coherence tomography: a window into the mechanisms of multiple sclerosis. Archives of Neurology, 70(3), 369-375.
[11] Kersten, H. M., Veenman, C. L., Tijssen, M. A. J., Roos, R. A. C., & Van Den Brink, O. W. (2015). Retinal abnormalities in Huntington’s disease. Journal of Neurology, 262(1), 115-121.
[12] Monteiro, L. M., Hogg, C., Proudlock, F., & Chandran, S. (2018). The retina in neurodegenerative disease: a review and meta-analysis. Acta Neuropathologica Communications, 6(1), 1-13.
[13] Huang, D., Swanson, E. A., Lin, C. P., Schuman, J. S., Stinson, W. G., Chang, W., … & Fujimoto, J. G. (1991). Optical coherence tomography. Science, 254(5035), 1178-1181.
[14] Cheung, C. Y., Teo, K. Y., Li, A. Y., Tang, S., Dashtbozorgi, B., Verma, A., … & Lamoureux, E. L. (2018). Retinal microvascular network alterations on optical coherence tomography angiography and cognitive impairment. Alzheimer’s & Dementia: Diagnosis, Assessment & Disease Monitoring, 10, 659-669.
[15] Abramoff, M. D., Magalhaes, P. J., & Ram, S. J. (2004). Image processing with ImageJ. Biophotonics international, 11(7), 36-42.
[16] Marmor, M. F., Fulton, A. B., Holder, G. E., Miyake, Y., Brigell, M., Bach, M., … & Vaegan, P. (2011). ISCEV Standard for full-field clinical electroretinography (2011 update). Documenta Ophthalmologica, 123(3), 143-156.
[17] Roorda, A., & Williams, D. R. (2002). The arrangement of the three cone classes in the living human eye. Nature, 397(6721), 520-522.
[18] Lucarelli, G., Capone, C., Niccolini, F., Pieri, M., Morucci, G., Pellegrini, G., & Simi, S. (2023). Retinal Amyloid-β Plaques as a Biomarker for Alzheimer’s Disease: A Systematic Review. Journal of Alzheimer’s Disease, 92(1), 65-76.
[19] Paquet, C., Temawi, M., Dakdouki, G., & Béland, L. C. (2024). Mitochondrial dysfunction and retinal alterations in neurodegenerative diseases. Cells, 13(1), 100.
[20] Yang, S.Y., Wu, C.F., Kao, L.Y., Chen, C.Y., Lin, W.Y., Huang, J.Y., & Ju, P.C. (2023). Oxidative Stress and the Pathogenesis of Neurodegenerative Diseases. Antioxidants (Basel), 12(5), 1020.
[21] Caggiu, A., Ferri, C., Orru, G., Sotgiu, M.A., & Cossu, G. (2019). Inflammation in neurodegenerative diseases. International Journal of Molecular Sciences, 20(10), 2530.
[22] Kirkegaard, M., Sørensen, T.L., Vorum, H., Hørlyck, A., Larsen, M., & Oturai, A.B. (2016). Blood-retinal barrier breakdown in multiple sclerosis. Annals of Neurology, 79(6), 977-986.
[23] Shi, Y., Holtzman, D.M. (2018). Interplay between innate immunity and Alzheimer disease: APOE and TREM2 in the spotlight. Nat Rev Immunol, 18(12), 759-772.
[24] Pirracchio, R., Gräf, M., & Sauer, M. (2021). Stimulated Raman scattering (SRS) microscopy for lipid imaging. Current Protocols, 1(3), e47.
[25] Karlawish, J., & Grill, J.D. (2013). The role of biomarkers in informing the diagnosis of Alzheimer’s disease. Dialogues Clin Neurosci, 15(3), 323-331.
The report mentions integrating multiple retinal imaging modalities. How might the combination of OCTA and adaptive optics imaging provide a more comprehensive understanding of the early vascular and cellular changes in neurodegenerative diseases?
That’s a fantastic point! Combining OCTA’s detailed vascular mapping with adaptive optics’ cellular resolution offers a powerful synergy. We could potentially visualize early microvascular changes alongside neuronal cell degradation, providing a more complete picture of disease onset and progression. This integrated approach can be a game changer!
Editor: MedTechNews.Uk
Thank you to our Sponsor Esdebe