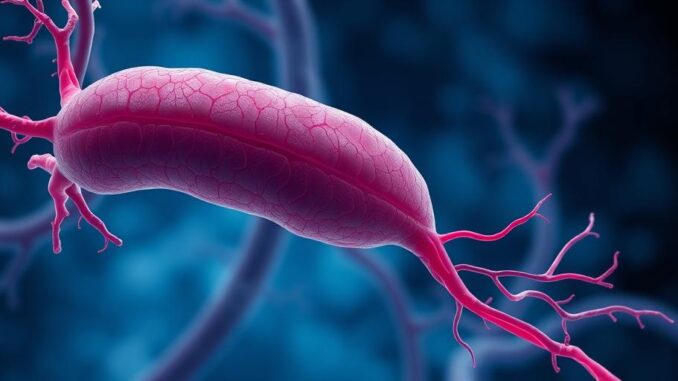
Abstract
The study of pancreatic islet biology, particularly in the context of diabetes mellitus, has been significantly advanced by the advent of stem cell-derived models. These models offer unprecedented opportunities to investigate islet development, function, and dysfunction in both health and disease. This report provides a comprehensive overview of various stem cell-based approaches for modeling the endocrine pancreas, with a focus on recent advancements and their potential impact on drug discovery and personalized medicine. We critically evaluate the strengths and limitations of different stem cell sources, differentiation protocols, and functional assays used in the field. Further, we discuss the application of these models to dissect the pathophysiology of specific diabetes subtypes, including Type 1 Diabetes (T1D), Type 2 Diabetes (T2D), and monogenic forms of diabetes. Finally, we explore future directions for the field, highlighting the need for improved model complexity, enhanced reproducibility, and the integration of multi-omics data to achieve more physiologically relevant representations of the human pancreatic islet.
Many thanks to our sponsor Esdebe who helped us prepare this research report.
1. Introduction
The endocrine pancreas, comprising the islets of Langerhans, plays a critical role in glucose homeostasis through the regulated secretion of hormones, primarily insulin from β-cells and glucagon from α-cells. Dysregulation of islet function is central to the pathogenesis of diabetes mellitus, a global health crisis characterized by chronic hyperglycemia. Understanding the intricate mechanisms governing islet development, cell-type specific function, and inter-islet communication is essential for developing effective strategies for diabetes prevention and treatment. However, research has been hampered by limited access to high-quality human islet tissue and the inherent limitations of animal models, which often fail to fully recapitulate human islet physiology.
Stem cell-derived models offer a powerful alternative, providing a renewable and scalable source of human islet cells for research. These models can be generated from various stem cell sources, including embryonic stem cells (ESCs), induced pluripotent stem cells (iPSCs), and adult stem cells. Through carefully orchestrated differentiation protocols, these stem cells can be directed to differentiate into pancreatic progenitor cells and, ultimately, into mature hormone-producing islet cells. The resulting stem cell-derived islets (SC-islets) represent a valuable tool for studying islet biology, disease modeling, drug screening, and potentially, cell-based therapies for diabetes.
This report will delve into the different types of stem cell models used in diabetes research, evaluate their advantages and limitations, discuss specific protocols for creating and utilizing these models, and explore their potential applications in drug discovery and personalized medicine. The analysis will encompass the broader context of stem cell research in diabetes, extending beyond a single alpha-cell model to consider the entire islet landscape and its intricate cellular interactions. The focus will remain on applications for basic science and translational research.
Many thanks to our sponsor Esdebe who helped us prepare this research report.
2. Stem Cell Sources for Endocrine Pancreas Differentiation
2.1 Embryonic Stem Cells (ESCs)
ESCs, derived from the inner cell mass of blastocysts, possess the capacity for self-renewal and pluripotency, meaning they can differentiate into any cell type in the body. Their well-characterized developmental potential has made them a foundational source for generating pancreatic cells in vitro. Differentiation protocols typically involve mimicking key developmental signals, such as activin A, Wnt3a, and retinoic acid, to guide ESCs through stages resembling definitive endoderm, pancreatic progenitor, and endocrine precursor development [1]. While ESCs offer a consistent and readily expandable source of cells, ethical concerns surrounding their derivation remain a significant hurdle, and the possibility of teratoma formation after transplantation warrants careful consideration.
2.2 Induced Pluripotent Stem Cells (iPSCs)
iPSCs, generated by reprogramming somatic cells (e.g., skin fibroblasts or blood cells) using defined transcription factors (e.g., Oct4, Sox2, Klf4, and c-Myc), circumvent the ethical issues associated with ESCs. iPSC technology enables the creation of patient-specific cell lines, opening doors to personalized medicine and disease modeling. iPSC-derived pancreatic cells have been successfully generated using similar differentiation protocols as those used for ESCs, with modifications to optimize efficiency and maturation [2]. However, iPSC lines can exhibit variability in differentiation potential due to factors such as incomplete reprogramming or epigenetic memory, requiring careful characterization and clonal selection.
2.3 Adult Stem Cells
Adult stem cells, residing within mature tissues, possess a more restricted differentiation potential compared to ESCs and iPSCs. Several adult stem cell populations, including pancreatic ductal cells and mesenchymal stem cells (MSCs), have been explored as potential sources for generating islet cells. While these cells offer the advantage of being readily accessible and lacking ethical concerns, their differentiation potential towards pancreatic lineages is often limited, and their contribution to functional islet formation remains controversial. MSCs, for example, have been shown to secrete factors that promote β-cell survival and function, but their direct differentiation into functional insulin-producing cells is less well-established [3]. Furthermore, issues of replicative senescence in adult stem cells can create logistical limitations.
Many thanks to our sponsor Esdebe who helped us prepare this research report.
3. Differentiation Protocols and Stages
Generating functional SC-islets from pluripotent stem cells is a complex and multistep process that mimics embryonic pancreas development. Differentiation protocols typically involve sequential exposure to growth factors and small molecules that activate or inhibit key signaling pathways involved in pancreas development [4]. The differentiation process can be broadly divided into the following stages:
3.1 Definitive Endoderm (DE) Induction
The first step involves inducing pluripotent stem cells to differentiate into definitive endoderm, the germ layer that gives rise to the pancreas, liver, lungs, and gastrointestinal tract. This is typically achieved by activating the Activin/Nodal signaling pathway using activin A, often in combination with a Wnt activator, such as CHIR99021. DE cells express markers such as SOX17 and FOXA2.
3.2 Pancreatic Progenitor (PP) Specification
DE cells are then directed towards a pancreatic fate by inhibiting the Activin/Nodal signaling pathway and activating the FGF and retinoic acid signaling pathways. This stage results in the formation of pancreatic progenitor cells that express markers such as PDX1, NKX6.1, and PTF1A.
3.3 Endocrine Differentiation
PP cells are further differentiated towards endocrine lineages by modulating Notch signaling and providing appropriate extracellular matrix components. This stage leads to the generation of endocrine precursor cells that express markers such as NGN3 and ISL1. The choice and timing of growth factor addition at this stage can significantly impact the ratio of alpha, beta, and other islet cells that are ultimately created. Specific signaling environments are used to drive maturation into functional alpha, beta, or other islet cells.
3.4 Maturation and Aggregation
Finally, endocrine precursor cells are allowed to mature into functional hormone-producing cells. This stage often involves culturing the cells in three-dimensional (3D) aggregates or embedding them in biomaterials to mimic the islet microenvironment. The resulting SC-islets can secrete insulin and glucagon in response to glucose and other stimuli [5].
Many thanks to our sponsor Esdebe who helped us prepare this research report.
4. Functional Characterization of Stem Cell-Derived Islet Cells
A crucial aspect of evaluating stem cell-derived islet cells is to assess their functionality using a range of in vitro and in vivo assays.
4.1 Static Glucose-Stimulated Insulin Secretion (GSIS) Assay
This is the most commonly used assay to assess β-cell function. SC-islets are incubated in media containing low glucose concentrations, followed by media containing high glucose concentrations. Insulin secretion is measured by ELISA. A functional β-cell will secrete significantly more insulin at high glucose concentrations compared to low glucose concentrations.
4.2 Dynamic GSIS Assay
This assay provides a more detailed assessment of insulin secretion kinetics. SC-islets are perifused with media containing varying glucose concentrations, and insulin secretion is continuously monitored using an automated system. This assay can reveal defects in the first and second phases of insulin secretion, which are often observed in T2D.
4.3 Glucose Uptake Assay
This assay measures the ability of SC-islets to uptake glucose. SC-islets are incubated with a fluorescently labeled glucose analog (e.g., 2-NBDG), and glucose uptake is quantified using flow cytometry or microscopy.
4.4 Electrophysiology
Patch-clamp electrophysiology can be used to assess the electrical activity of SC-islet cells. This technique can measure the opening and closing of ion channels, which are crucial for regulating insulin secretion. Changes to ion channel activity can be indicative of dysfunction.
4.5 In Vivo Transplantation Studies
To assess the in vivo functionality of SC-islets, they can be transplanted into immunocompromised mice. The transplanted SC-islets can engraft and secrete insulin, leading to improved glucose homeostasis in diabetic animals. This is generally considered the gold standard to assess the therapeutic potential of SC-islets.
4.6 Alpha Cell Function Assays
While GSIS is focused on Beta cell function, understanding Alpha cell function, glucagon secretion, and response to low glucose is critical to understanding overall islet function. Measurement of glucagon secretion in response to low glucose conditions is vital in assessing overall islet health.
Many thanks to our sponsor Esdebe who helped us prepare this research report.
5. Applications of Stem Cell-Derived Islet Models
5.1 Disease Modeling
Stem cell-derived islet models are invaluable tools for studying the pathogenesis of diabetes. iPSCs generated from patients with T1D, T2D, or monogenic forms of diabetes can be differentiated into islet cells to investigate the underlying cellular and molecular mechanisms of these diseases. For example, iPSCs from patients with T1D can be used to study the immune-mediated destruction of β-cells, while iPSCs from patients with T2D can be used to investigate β-cell dysfunction and insulin resistance [6].
5.2 Drug Discovery
SC-islets can be used as a platform for high-throughput drug screening to identify novel compounds that promote β-cell survival, function, or regeneration. These models offer a more physiologically relevant alternative to traditional cell lines, as they recapitulate key aspects of human islet biology. Furthermore, patient-specific iPSC-derived islet cells can be used to screen for personalized therapies that are tailored to individual patients.
5.3 Personalized Medicine
The ability to generate patient-specific iPSC-derived islet cells opens the door to personalized medicine for diabetes. These cells can be used to predict an individual’s response to different diabetes treatments, allowing clinicians to select the most effective therapy for each patient. Furthermore, patient-specific iPSC-derived islet cells can be used to develop cell-based therapies that are perfectly matched to the patient’s immune system, minimizing the risk of rejection [7].
5.4 Understanding Alpha-Cell Dysfunction
As highlighted in the initial prompt, a stem cell model specifically designed to study alpha-cell dysfunction is a crucial addition to the field. Alpha-cell dysfunction contributes to both hypoglycemia and hyperglycemia in diabetes. Stem cell models allowing for detailed study of these cells is vital.
Many thanks to our sponsor Esdebe who helped us prepare this research report.
6. Limitations and Challenges
Despite the significant advances in stem cell-derived islet models, several limitations and challenges remain:
6.1 Incomplete Maturation
SC-islets often exhibit an immature phenotype compared to adult human islets. They may express lower levels of key islet-specific genes and exhibit reduced glucose responsiveness. Further research is needed to optimize differentiation protocols and promote full maturation of SC-islets [8].
6.2 Heterogeneity
SC-islet preparations often contain a mixture of different cell types, including β-cells, α-cells, δ-cells, and PP cells. This heterogeneity can complicate the interpretation of experimental results and limit the reproducibility of studies. Strategies to improve cell-type purity, such as FACS sorting or microfluidic devices, are needed.
6.3 Scalability and Cost
Generating large quantities of SC-islets can be technically challenging and expensive. Developing scalable and cost-effective differentiation protocols is essential for widespread adoption of these models.
6.4 Immune Rejection
Transplanted SC-islets are susceptible to immune rejection, particularly in the context of allogeneic transplantation. Strategies to overcome immune rejection, such as encapsulation or genetic modification, are needed to develop cell-based therapies for diabetes. Recent advances in creating hypoimmunogenic cells offer a promising path forward.
6.5 Glucose Responsiveness
Stem cell-derived beta cells frequently exhibit reduced glucose responsiveness compared to native islet beta cells. Improving glucose sensing and insulin secretion capacity is vital for achieving therapeutic results.
Many thanks to our sponsor Esdebe who helped us prepare this research report.
7. Future Directions
The field of stem cell-derived islet models is rapidly evolving. Future research directions include:
7.1 Improving Differentiation Protocols
Developing more efficient and robust differentiation protocols that consistently generate fully mature and functional SC-islets. This may involve the use of novel growth factors, small molecules, or biomaterials. Furthermore, mimicking the 3D architecture of the native islet in vitro is crucial for achieving optimal islet function.
7.2 Incorporating Vascularization and Innervation
Developing methods to vascularize and innervate SC-islets in vitro or in vivo. This would improve nutrient and oxygen delivery to the cells and allow for more physiological regulation of hormone secretion.
7.3 Integrating Multi-Omics Data
Integrating multi-omics data (e.g., genomics, transcriptomics, proteomics, and metabolomics) to gain a more comprehensive understanding of SC-islet development, function, and dysfunction. This will allow for the identification of novel targets for drug discovery and personalized medicine.
7.4 CRISPR-Based Gene Editing
Utilizing CRISPR-based gene editing to correct genetic defects in iPSCs from patients with monogenic forms of diabetes. This would allow for the generation of isogenic control lines and facilitate the study of disease mechanisms.
7.5 Microfluidics and Organ-on-a-Chip Technology
Implementing microfluidic devices and organ-on-a-chip technology to create more physiologically relevant in vitro models of the pancreatic islet. These platforms can mimic the islet microenvironment, including cell-cell interactions, fluid flow, and mechanical forces.
7.6 Focusing on Islet Subtypes
There is a growing understanding of islet heterogeneity. Developing differentiation protocols and model systems that allow for focusing on generating specific islet subtypes, like specific subtypes of alpha or beta cells, will likely be of benefit.
Many thanks to our sponsor Esdebe who helped us prepare this research report.
8. Conclusion
Stem cell-derived islet models represent a powerful tool for studying pancreatic islet biology and developing new therapies for diabetes. While significant progress has been made in this field, several challenges remain. Addressing these challenges through continued research and innovation will pave the way for the widespread application of stem cell-derived islet models in drug discovery, personalized medicine, and ultimately, cell-based therapies for diabetes. The focus on more complex, accurate, and physiologically relevant models will accelerate advancements in understanding diabetes and designing effective treatments.
Many thanks to our sponsor Esdebe who helped us prepare this research report.
References
[1] D’Amour, K. A., et al. “Production of pancreatic hormone–expressing endocrine cells from human embryonic stem cells.” Nature biotechnology 24.11 (2006): 1392-1401.
[2] Maeve A Duffin, Gulam A Manan, Maria I Arvanites, Shuibing Chen. Generation of human pancreatic progenitors from induced pluripotent stem cells. Methods Mol Biol. 2015;1257:293-304. doi: 10.1007/978-1-4939-2242-7_20.
[3] Lee, R. H., et al. “Human mesenchymal stem cells induce metabolic changes in glucose-sensitive pancreatic cells.” Stem cells 24.8 (2006): 1809-1816.
[4] Nostro, M. C., et al. “Stage-specific signaling through TGFβ family members and WNT regulates patterning and pancreatic progenitor formation from human embryonic stem cells.” Development 138.5 (2011): 861-871.
[5] Pagliuca, F. W., et al. “Generation of functional human pancreatic β cells in vitro.” Cell 159.2 (2014): 428-439.
[6] Maeve A Duffin, Shuibing Chen. Stem cell modeling of pancreatic beta cell defects in diabetes. Curr Diab Rep. 2014 Jul;14(7):502. doi: 10.1007/s11892-014-0502-7.
[7] Melton, D. A. “Diabetes: cell therapy’s next steps.” Nature 502.7472 (2013): 488-489.
[8] Ben-Othman, N., et al. “Long-term GABA treatment induces human beta-cell proliferation and regulates glucose homeostasis.” Cell 168.4 (2017): 529-541.e11.
So, if we can now build replacement pancreatic islets, can we finally have that all-bacon-and-donut diet we’ve always dreamed of? Asking for purely scientific reasons, of course!
That’s a delicious question! While replacement islets offer hope, remember they still need a functioning body to support them. Overloading on bacon and donuts might overwhelm even the best replacement parts. Let’s focus on healthy habits while we advance the science!
Editor: MedTechNews.Uk
Thank you to our Sponsor Esdebe
The discussion on SC-islet heterogeneity raises important questions about reproducibility. Are there emerging techniques, such as single-cell RNA sequencing, being used to characterize and potentially sort these cell populations for more consistent results in drug screening or disease modeling?
That’s a fantastic point! Single-cell RNA sequencing is definitely gaining traction for characterizing SC-islet heterogeneity. Beyond characterization, researchers are exploring FACS and microfluidic sorting techniques to isolate specific cell populations. This precision could significantly improve the reproducibility and reliability of drug screening and disease modeling using these models. It’s an exciting area of development!
Editor: MedTechNews.Uk
Thank you to our Sponsor Esdebe
The discussion of limitations highlights the need for improved glucose responsiveness in stem cell-derived beta cells. What strategies, such as genetic modification or advanced culture techniques, show the most promise for enhancing this crucial function?
That’s a great question! Beyond genetic modification and culture techniques, I think exploring the role of the extracellular matrix in beta cell maturation could also be key. Mimicking the native islet microenvironment more closely might unlock enhanced glucose responsiveness. It’s definitely a multi-faceted challenge!
Editor: MedTechNews.Uk
Thank you to our Sponsor Esdebe
Given the challenges of SC-islet immaturity, could advancements in 3D bioprinting, which allow for precise spatial control over cell placement and microenvironment creation, play a crucial role in generating more functional and mature islet models?
That’s a great point! 3D bioprinting offers exciting possibilities for creating more mature SC-islets. Mimicking the native islet architecture and precisely controlling the cellular microenvironment could significantly improve their functionality and glucose responsiveness. Exploring different biomaterials and printing techniques will be crucial for optimizing islet development. I am very excited to see the progress in this field!
Editor: MedTechNews.Uk
Thank you to our Sponsor Esdebe