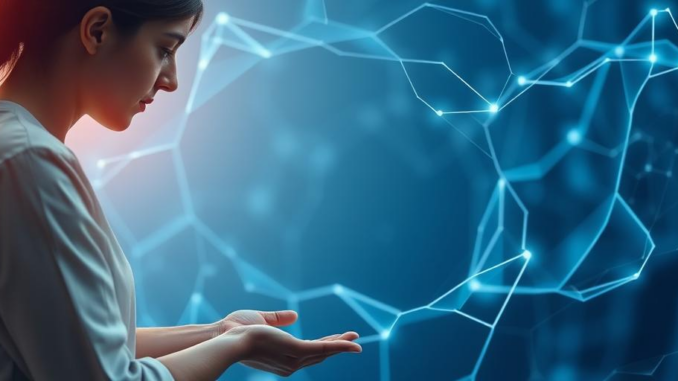
Abstract
Stem cell therapy has emerged as a promising therapeutic avenue for a wide range of diseases, driven by the unique capacity of stem cells to self-renew and differentiate into specialized cell types. This research report provides a comprehensive overview of stem cell therapy, encompassing the diverse types of stem cells used, methodologies for directing differentiation, clinical trial progress across various disease areas, inherent challenges, and pertinent ethical considerations. We delve into the nuances of embryonic stem cells (ESCs), induced pluripotent stem cells (iPSCs), mesenchymal stem cells (MSCs), and hematopoietic stem cells (HSCs), examining their respective advantages and limitations in therapeutic contexts. Differentiation strategies, including growth factor manipulation, genetic engineering, and three-dimensional culture systems, are critically evaluated. Furthermore, we scrutinize the progress of clinical trials targeting conditions such as diabetes, cardiovascular diseases, neurodegenerative disorders, and autoimmune diseases, highlighting successes, setbacks, and ongoing investigations. The report also addresses critical challenges, including immune rejection, tumorigenicity, scalability of cell production, and the need for precise control over differentiation. Finally, we explore the complex ethical landscape surrounding stem cell research and therapy, emphasizing issues of informed consent, equitable access, and the moral status of the embryo. This review aims to provide a valuable resource for researchers, clinicians, and policymakers involved in advancing the field of stem cell therapy towards safe and effective clinical translation.
Many thanks to our sponsor Esdebe who helped us prepare this research report.
1. Introduction
Stem cell therapy represents a revolutionary approach to medicine, harnessing the regenerative potential of stem cells to repair damaged tissues, replace lost cells, and restore organ function. Unlike conventional therapies that primarily address symptoms, stem cell therapy targets the underlying causes of disease by directly addressing cellular dysfunction or loss. The field has witnessed exponential growth in recent decades, fueled by advancements in stem cell biology, cell engineering, and clinical trial design. This has expanded potential applications from well-established treatments, such as bone marrow transplantation, to experimental therapies for a wide range of debilitating conditions.
The defining characteristic of stem cells is their capacity for self-renewal, the ability to divide and create more stem cells, and differentiation, the process by which they transform into specialized cell types with distinct functions. This dual capacity makes stem cells ideal candidates for regenerative medicine, where they can be used to replenish damaged or diseased tissues. Stem cells are categorized based on their developmental origin and differentiation potential. Totipotent stem cells, such as the zygote, have the capacity to generate all cell types in the body, including extraembryonic tissues. Pluripotent stem cells, like ESCs and iPSCs, can differentiate into all cell types of the three germ layers (ectoderm, mesoderm, and endoderm), but cannot form extraembryonic tissues. Multipotent stem cells, such as HSCs and MSCs, are restricted to differentiating into a limited range of cell types within a specific tissue lineage. The choice of stem cell type for a particular therapeutic application depends on the specific disease, the desired cell type for replacement, and the potential for immune rejection or tumorigenicity.
This report aims to provide a comprehensive overview of stem cell therapy, exploring the different types of stem cells, methodologies for directing differentiation, clinical trial progress, challenges, and ethical considerations. By synthesizing the current state of knowledge and highlighting key areas of ongoing research, this review seeks to inform and guide future efforts in translating stem cell therapy into effective clinical treatments.
Many thanks to our sponsor Esdebe who helped us prepare this research report.
2. Types of Stem Cells
2.1 Embryonic Stem Cells (ESCs)
ESCs, derived from the inner cell mass of the blastocyst, are pluripotent cells with the potential to differentiate into any cell type in the body. Their pluripotency makes them a valuable resource for regenerative medicine, offering the possibility of generating an unlimited supply of specialized cells for transplantation. ESCs can be cultured indefinitely in vitro while maintaining their pluripotency, providing a stable and expandable source of cells for research and therapy. However, the use of ESCs raises significant ethical concerns due to their derivation from human embryos, which involves the destruction of the embryo. This ethical dilemma has fueled the search for alternative sources of pluripotent stem cells, such as iPSCs.
From a technical standpoint, ESC differentiation protocols are complex and often result in heterogeneous cell populations. Controlling the differentiation process to generate specific cell types with high purity and functionality remains a significant challenge. Furthermore, ESC-derived cells can form teratomas, tumors composed of multiple tissue types, if undifferentiated cells are present in the transplant. Careful quality control and elimination of undifferentiated cells are crucial to ensure the safety of ESC-based therapies. Despite these challenges, ESCs remain a valuable tool for studying early human development and modeling disease in vitro.
2.2 Induced Pluripotent Stem Cells (iPSCs)
iPSCs are generated by reprogramming somatic cells, such as skin fibroblasts or blood cells, to a pluripotent state through the introduction of specific transcription factors. This groundbreaking discovery, pioneered by Shinya Yamanaka, revolutionized the field of stem cell biology by providing a means to generate pluripotent stem cells without the ethical concerns associated with ESCs. iPSCs exhibit similar characteristics to ESCs, including pluripotency, self-renewal, and the ability to differentiate into all three germ layers. iPSCs offer several advantages over ESCs, including the ability to generate patient-specific cells, which can reduce the risk of immune rejection after transplantation. The generation of iPSCs also provides a powerful tool for studying disease mechanisms and developing personalized therapies.
However, iPSC technology also faces several challenges. The reprogramming process can be inefficient and may result in cells with incomplete or aberrant reprogramming. iPSCs can retain epigenetic memory of their tissue of origin, which may influence their differentiation potential. Furthermore, the use of viral vectors for introducing reprogramming factors can raise safety concerns due to the risk of insertional mutagenesis and tumorigenicity. Although newer reprogramming methods using non-integrating vectors or small molecules have emerged, they often result in lower reprogramming efficiency. Additionally, there are concerns about the genomic stability of iPSCs after prolonged culture. Rigorous characterization and quality control are essential to ensure the safety and efficacy of iPSC-based therapies. Some recent work suggests that iPSCs may also be prone to accelerated aging, impacting their long-term viability and function post-transplantation.
2.3 Mesenchymal Stem Cells (MSCs)
MSCs are multipotent stem cells that can be isolated from various tissues, including bone marrow, adipose tissue, and umbilical cord blood. They have the capacity to differentiate into a variety of cell types, including osteoblasts, chondrocytes, and adipocytes. MSCs are known for their immunomodulatory properties, which make them attractive candidates for treating autoimmune diseases and inflammatory conditions. MSCs secrete a variety of growth factors and cytokines that can promote tissue repair and regeneration. They can be easily isolated and expanded in vitro, making them a readily available source of cells for therapeutic applications. Furthermore, MSCs are generally considered to be immune privileged, meaning that they can be transplanted across histocompatibility barriers without eliciting a strong immune response.
However, the differentiation potential of MSCs is limited compared to pluripotent stem cells. MSCs are not capable of differentiating into all cell types of the body, restricting their use to specific tissue lineages. The therapeutic mechanisms of MSCs are not fully understood, and their efficacy in clinical trials has been variable. Some studies suggest that MSCs exert their therapeutic effects primarily through paracrine signaling, rather than direct cell replacement. The heterogeneity of MSC populations and the lack of standardized isolation and culture protocols can also contribute to variability in therapeutic outcomes. Furthermore, there are concerns about the potential for MSCs to promote tumor growth in certain contexts. Careful consideration of the source, culture conditions, and therapeutic application of MSCs is essential to maximize their therapeutic potential and minimize potential risks.
2.4 Hematopoietic Stem Cells (HSCs)
HSCs reside in the bone marrow and are responsible for generating all blood cell types, including red blood cells, white blood cells, and platelets. HSC transplantation is a well-established therapy for treating hematological malignancies, such as leukemia and lymphoma, as well as certain genetic blood disorders. HSCs can be isolated from bone marrow, peripheral blood, or umbilical cord blood. HSC transplantation involves replacing the patient’s diseased bone marrow with healthy HSCs, which can then reconstitute the patient’s blood system.
The success of HSC transplantation depends on the availability of a suitable donor, either a matched sibling or an unrelated donor. Graft-versus-host disease (GVHD) is a major complication of allogeneic HSC transplantation, in which the donor’s immune cells attack the patient’s tissues. Immunosuppressive drugs are used to prevent or treat GVHD. Autologous HSC transplantation, in which the patient’s own HSCs are collected and reinfused after high-dose chemotherapy or radiation, eliminates the risk of GVHD but may not be effective in treating certain malignancies. Gene therapy approaches are being developed to modify HSCs ex vivo before transplantation, offering the potential to correct genetic defects or enhance their anti-tumor activity.
Many thanks to our sponsor Esdebe who helped us prepare this research report.
3. Differentiation Strategies
The ability to control the differentiation of stem cells into specific cell types is crucial for realizing the full potential of stem cell therapy. Numerous differentiation strategies have been developed, ranging from simple growth factor manipulation to complex genetic engineering approaches.
3.1 Growth Factor Manipulation
Growth factors are signaling molecules that bind to receptors on stem cells and trigger intracellular signaling pathways that regulate differentiation. By carefully selecting and combining growth factors, researchers can direct stem cells towards specific cell lineages. For example, bone morphogenetic proteins (BMPs) can induce differentiation of MSCs into osteoblasts, while nerve growth factor (NGF) can promote differentiation of neuronal stem cells into neurons. The optimal combination and concentration of growth factors vary depending on the stem cell type and the desired cell fate. This process often requires iterative optimization and careful monitoring of cell morphology and gene expression.
3.2 Genetic Engineering
Genetic engineering techniques can be used to introduce genes that promote differentiation or to silence genes that inhibit differentiation. For example, transcription factors that are essential for the development of a particular cell type can be expressed in stem cells to drive their differentiation along that lineage. CRISPR-Cas9 gene editing technology allows for precise modification of the stem cell genome, enabling the correction of genetic defects or the introduction of specific mutations that enhance differentiation. However, genetic engineering approaches also raise safety concerns, such as the risk of insertional mutagenesis and off-target effects.
3.3 Three-Dimensional Culture Systems
Traditional two-dimensional (2D) cell culture systems can limit cell-cell interactions and fail to mimic the complex microenvironment of tissues in vivo. Three-dimensional (3D) culture systems provide a more physiologically relevant environment for stem cell differentiation, allowing for enhanced cell-cell and cell-matrix interactions. 3D culture systems can be created using various materials, such as hydrogels, scaffolds, and microcarriers. These systems can promote the formation of tissue-like structures and enhance the differentiation and function of stem cells.
3.4 Small Molecule Approaches
Small molecules can modulate intracellular signaling pathways and influence stem cell differentiation. These compounds offer the advantage of being easily synthesized and administered. Screening libraries of small molecules can identify compounds that promote differentiation towards specific cell lineages. Small molecules can also be used to replace growth factors, reducing the cost and complexity of differentiation protocols. Some small molecules can act synergistically with growth factors to enhance differentiation efficiency.
Many thanks to our sponsor Esdebe who helped us prepare this research report.
4. Clinical Trial Progress
Stem cell therapy has shown promise in clinical trials for a variety of diseases. Here are some key examples. It is not exhaustive, but illustrative of the breadth of research.
4.1 Diabetes
Type 1 diabetes is an autoimmune disease in which the immune system destroys insulin-producing beta cells in the pancreas. Stem cell therapy offers the potential to replace these lost beta cells and restore insulin production. Clinical trials using ESC-derived or iPSC-derived beta cells have shown some success in reducing or eliminating the need for insulin injections in patients with type 1 diabetes. However, challenges remain in preventing immune rejection of the transplanted cells and ensuring their long-term survival and function. Encapsulation strategies, in which beta cells are encased in a protective barrier that prevents immune cell access but allows for insulin secretion, are being developed to overcome the immune rejection problem.
4.2 Cardiovascular Diseases
Stem cell therapy has been investigated as a treatment for various cardiovascular diseases, including heart failure, myocardial infarction, and peripheral artery disease. MSCs and HSCs have been used in clinical trials to promote angiogenesis, reduce scar tissue formation, and improve cardiac function. While some studies have shown modest improvements in cardiac function and exercise capacity, the overall clinical benefits have been limited. The mechanisms of action of stem cells in cardiovascular disease are not fully understood, and the optimal cell type, delivery method, and timing of treatment remain to be determined. Some research has focused on using cardiac progenitor cells, stem cells already committed to the cardiac lineage, to enhance cell engraftment and regeneration.
4.3 Neurodegenerative Disorders
Stem cell therapy offers the potential to replace lost neurons and support the survival of existing neurons in neurodegenerative disorders such as Parkinson’s disease, Alzheimer’s disease, and amyotrophic lateral sclerosis (ALS). Clinical trials using neural stem cells (NSCs) or MSCs have shown some promise in improving motor function and cognitive function in patients with these disorders. However, challenges remain in ensuring the survival and integration of transplanted cells into the brain, as well as preventing immune rejection and tumor formation. Furthermore, the blood-brain barrier poses a significant obstacle to delivering stem cells to the brain. Some approaches involve delivering cells directly into the brain via stereotactic surgery, while others focus on developing methods to enhance stem cell migration across the blood-brain barrier. Early-stage clinical trials are exploring gene-edited stem cells for conditions like Huntington’s disease to address the underlying genetic cause.
4.4 Autoimmune Diseases
Stem cell therapy, particularly using autologous HSC transplantation, has shown significant efficacy in treating severe autoimmune diseases such as multiple sclerosis, rheumatoid arthritis, and systemic lupus erythematosus. The procedure involves harvesting the patient’s own HSCs, followed by high-dose chemotherapy to eliminate the patient’s immune system, and then reinfusing the HSCs to reconstitute a new, healthy immune system. This approach can effectively reset the immune system and induce long-term remission in some patients. However, HSC transplantation is an intensive and risky procedure with potential side effects, including infection, bleeding, and organ damage. Careful patient selection and monitoring are essential to minimize these risks.
Many thanks to our sponsor Esdebe who helped us prepare this research report.
5. Challenges and Future Directions
While stem cell therapy holds immense promise, several challenges must be addressed to realize its full potential.
5.1 Immune Rejection
Immune rejection is a major obstacle to successful stem cell transplantation. Allogeneic stem cells, derived from a donor, can be recognized as foreign by the recipient’s immune system and elicit an immune response that destroys the transplanted cells. Immunosuppressive drugs can be used to prevent or treat immune rejection, but these drugs can have significant side effects. Strategies to minimize immune rejection include using autologous stem cells, generating hypoimmunogenic stem cells through genetic engineering, and encapsulating stem cells in a protective barrier that prevents immune cell access. Developing universally compatible (off-the-shelf) stem cell lines is a key goal for future research.
5.2 Tumorigenicity
Stem cells, particularly pluripotent stem cells, have the potential to form tumors if undifferentiated cells are present in the transplant. Careful quality control and elimination of undifferentiated cells are crucial to ensure the safety of stem cell therapies. Differentiation protocols must be optimized to generate fully differentiated cells that are less likely to form tumors. Furthermore, long-term monitoring of patients after stem cell transplantation is essential to detect any signs of tumor formation. Research is focused on identifying and eliminating tumorigenic stem cell subpopulations and developing methods to control stem cell proliferation after transplantation.
5.3 Scalability of Cell Production
Producing sufficient quantities of stem cells for therapeutic applications is a major challenge. Traditional 2D cell culture methods are often labor-intensive and inefficient. Bioreactors and other large-scale culture systems are needed to produce the large numbers of cells required for clinical trials and commercialization. Optimization of culture conditions, including media composition, growth factors, and oxygen levels, is essential to maximize cell proliferation and maintain cell quality. Automation and closed-system processing can reduce the risk of contamination and improve the reproducibility of cell production.
5.4 Control of Differentiation
Precise control over stem cell differentiation is crucial for generating the desired cell type with high purity and functionality. Differentiation protocols must be optimized to minimize the formation of off-target cell types. Single-cell analysis techniques can be used to characterize the heterogeneity of stem cell populations and identify factors that influence differentiation. Microfluidic devices and other advanced technologies can be used to control the microenvironment of stem cells and enhance differentiation efficiency. The use of computational modeling can aid in the design and optimization of differentiation protocols.
5.5 Delivery Methods
The method of delivering stem cells to the target tissue can significantly impact therapeutic outcomes. Intravenous injection is a simple and non-invasive method, but it can result in poor cell engraftment and retention in the target tissue. Direct injection into the target tissue can improve cell engraftment, but it may be limited by accessibility and invasiveness. Scaffolds and hydrogels can be used to deliver stem cells in a controlled manner and promote tissue regeneration. Developing targeted delivery systems that can selectively deliver stem cells to the target tissue is a key area of ongoing research. Nanoparticles and exosomes are being explored as vehicles for delivering stem cells or their therapeutic factors to the target tissue.
Many thanks to our sponsor Esdebe who helped us prepare this research report.
6. Ethical Considerations
Stem cell research and therapy raise several ethical considerations that must be carefully addressed.
6.1 Informed Consent
Informed consent is essential for all patients participating in stem cell clinical trials. Patients must be fully informed about the potential risks and benefits of the therapy, as well as the alternative treatment options. They must also be informed about the experimental nature of the therapy and the possibility that it may not be effective. The informed consent process should be culturally sensitive and ensure that patients fully understand the information being presented.
6.2 Equitable Access
Stem cell therapies are often expensive and may not be accessible to all patients. Ensuring equitable access to these therapies is a major ethical challenge. Public funding and insurance coverage for stem cell therapies are needed to make them more accessible to underserved populations. Developing lower-cost stem cell production methods can also help to reduce the cost of these therapies.
6.3 Moral Status of the Embryo
The use of ESCs raises ethical concerns about the moral status of the embryo. Some people believe that the embryo has a right to life and should not be used for research or therapy. Others believe that the potential benefits of ESC research outweigh the moral concerns about the destruction of embryos. These ethical debates have led to restrictions on ESC research in some countries and states. The development of iPSC technology has provided an alternative source of pluripotent stem cells that does not involve the destruction of embryos, mitigating some of these ethical concerns.
6.4 Unproven Stem Cell Therapies
The proliferation of unproven stem cell therapies poses a significant ethical and safety concern. Many clinics offer stem cell treatments for a variety of diseases without adequate scientific evidence to support their safety and efficacy. These unproven therapies can be expensive and may carry significant risks, including infection, tumor formation, and immune rejection. Patients should be wary of clinics that make unsubstantiated claims about the benefits of stem cell therapy and should consult with qualified medical professionals before undergoing any stem cell treatment. Regulatory agencies need to strengthen oversight of stem cell clinics and take action against those that offer unproven and potentially harmful therapies.
Many thanks to our sponsor Esdebe who helped us prepare this research report.
7. Conclusion
Stem cell therapy holds enormous promise for treating a wide range of diseases and injuries. Significant progress has been made in understanding stem cell biology, developing differentiation strategies, and conducting clinical trials. However, several challenges remain, including immune rejection, tumorigenicity, scalability of cell production, and the need for precise control over differentiation. Continued research and development efforts are needed to overcome these challenges and translate stem cell therapy into safe and effective clinical treatments. Ethical considerations surrounding stem cell research and therapy must be carefully addressed to ensure that these technologies are used responsibly and equitably. As the field continues to evolve, it is essential to maintain a balance between scientific innovation and ethical responsibility, fostering a future where stem cell therapy can improve the lives of patients worldwide. The future likely lies in a combination of approaches: sophisticated gene editing to create safer and more effective stem cell lines, advanced biomaterials for improved cell delivery and integration, and a deeper understanding of the complex interactions between stem cells and their microenvironment.
Many thanks to our sponsor Esdebe who helped us prepare this research report.
References
- Blum, B., & Benvenisty, N. (2008). The tumorigenicity of human embryonic stem cells. Advances in Cancer Research, 100, 133-158.
- Daley, G. Q. (2012). The promise and perils of stem cell therapeutics. Cell Stem Cell, 10(6), 740-749.
- Hochedlinger, K., & Plath, K. (2009). Epigenetic inheritance and the memory of cell state. Cell, 136(3), 495-506.
- Lanza, R., Gearhart, J., Hogan, B., Melton, D., Pedersen, R., Thomson, J., & West, M. (1999). Opportunities for stem cell research. Science, 286(5449), 1891.
- Murry, C. E., Keller, G. (2008). Differentiation of embryonic stem cells to clinically relevant populations: lessons from cardiac differentiation. Cell Stem Cell, 2(4), 301-314.
- Trounson, A., McDonald, C. (2015). Stem cell therapies in clinical trials: progress and challenges. Cell Stem Cell, 17(1), 11-22.
- Volarevic, V., Nurkovic, J., Arsenijevic, N., & Stojkovic, M. (2017). Concise review: mesenchymal stem cells and their secretome in regenerative medicine. Regenerative Medicine, 12(1), 56-73.
- Yamanaka, S. (2012). Induced pluripotent stem cells: past, present, and future. Cell Stem Cell, 10(6), 678-684.
- Zakrzewski, W., Dobrzyński, M., Szymonowicz, M., Rybak, Z. (2019). Stem cells: past, present, and future. Stem Cell Research & Therapy, 10(1), 68.
- Reviewed the latest clinical trial information from the NIH Clinical Trials website: https://clinicaltrials.gov/
This comprehensive overview highlights the incredible potential of stem cell therapies. The discussion of directed differentiation strategies is particularly interesting; further research into optimizing these methods will be crucial for clinical translation and efficacy.
Thank you! I agree that directed differentiation is key. The ability to precisely control cell fate will not only improve efficacy but also reduce the risk of unwanted cell types arising post-transplantation. This is especially important when considering therapies for complex conditions such as neurodegenerative diseases where specificity is vital.
Editor: MedTechNews.Uk
Thank you to our Sponsor Esdebe
This report effectively highlights the ethical considerations surrounding stem cell therapies, particularly the challenges of equitable access. Further discussion on strategies to reduce the cost of these therapies, such as exploring innovative manufacturing processes, would be beneficial.
Thank you for your comment! I completely agree that reducing the cost of stem cell therapies is crucial for equitable access. Exploring innovative manufacturing processes, as you suggested, along with streamlined regulatory pathways, could significantly lower production costs and broaden access to these potentially life-changing treatments.
Editor: MedTechNews.Uk
Thank you to our Sponsor Esdebe