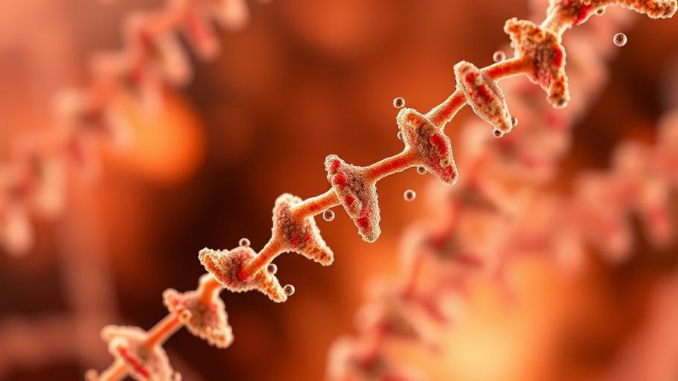
Abstract
Telomeres, the nucleoprotein complexes capping the ends of linear chromosomes, have emerged as critical regulators of cellular lifespan and genomic stability. Their primary function is to protect chromosome ends from degradation, fusion, and recognition as DNA damage. The progressive shortening of telomeres with each cell division, driven by the end-replication problem and oxidative stress, eventually triggers cellular senescence or apoptosis, limiting replicative capacity and contributing to organismal aging. However, the role of telomeres extends far beyond this well-established function. This report delves into the multifaceted nature of telomeres, exploring their intricate structure, the mechanisms governing their length regulation, and the diverse factors that influence their dynamics. We examine the expanding repertoire of telomere functions beyond genomic stability, including their influence on gene expression, DNA damage response, and stem cell maintenance. Furthermore, we critically evaluate current research on interventions aimed at modulating telomere length, from lifestyle modifications to pharmacological and genetic approaches, while addressing the inherent ethical considerations associated with such manipulations. Finally, we discuss the implications of telomere biology in various diseases, including cancer, aging-related disorders, and inherited syndromes, highlighting the potential for telomeres as therapeutic targets and biomarkers.
Many thanks to our sponsor Esdebe who helped us prepare this research report.
1. Introduction
Telomeres, derived from the Greek words telos (end) and meros (part), are specialized DNA-protein structures located at the termini of eukaryotic chromosomes. These structures play a vital role in maintaining genomic integrity and regulating cellular lifespan. Initially discovered by Hermann Muller and Barbara McClintock in the 1930s, their significance became fully appreciated with the elucidation of their structure and function in the following decades. Elizabeth Blackburn, Carol Greider, and Jack Szostak were awarded the Nobel Prize in Physiology or Medicine in 2009 for their discovery of telomerase, the enzyme responsible for telomere maintenance. This discovery revolutionized our understanding of telomere biology and its implications for aging, cancer, and various human diseases.
The primary function of telomeres is to protect chromosome ends from being recognized as DNA double-strand breaks (DSBs). Without telomeres, the cell’s DNA repair machinery would attempt to repair chromosome ends, leading to aberrant chromosome fusions and genomic instability. Telomeres also prevent the activation of DNA damage response pathways, which would otherwise trigger cellular senescence or apoptosis in response to unprotected chromosome ends. Furthermore, telomeres play a critical role in chromosome segregation during cell division, ensuring that chromosomes are accurately distributed to daughter cells.
In most somatic cells, telomeres progressively shorten with each cell division due to the end-replication problem. This problem arises because DNA polymerase cannot fully replicate the ends of linear chromosomes, resulting in a gradual loss of telomeric DNA. Once telomeres reach a critically short length, they trigger cellular senescence, a state of irreversible growth arrest, or apoptosis, programmed cell death. This process, known as replicative senescence or the Hayflick limit, limits the replicative capacity of cells and contributes to organismal aging. However, in certain cell types, such as germ cells and stem cells, telomere length is maintained by the enzyme telomerase, allowing these cells to divide indefinitely.
Beyond their role in genomic stability and cellular lifespan, telomeres have been implicated in a wide range of cellular processes, including gene expression, DNA damage response, and stem cell maintenance. Dysfunctional telomeres have been linked to various diseases, including cancer, aging-related disorders, and inherited syndromes. Therefore, understanding the intricacies of telomere biology is crucial for developing effective strategies to prevent and treat these diseases.
Many thanks to our sponsor Esdebe who helped us prepare this research report.
2. Structure and Composition of Telomeres
Telomeres are composed of repetitive DNA sequences and associated proteins, collectively forming a dynamic nucleoprotein complex. The DNA component consists of tandem repeats of a guanine-rich sequence, typically TTAGGG in vertebrates. These repeats can range in length from a few kilobases to over 15 kilobases, depending on the cell type and organism.
At the very end of the telomere, the G-rich strand extends beyond the C-rich strand, forming a single-stranded overhang. This overhang invades the double-stranded telomeric DNA, forming a loop-like structure called the t-loop. The formation of the t-loop protects the chromosome end from being recognized as a DNA break and prevents the activation of DNA damage response pathways. The displaced single-stranded DNA forms a displacement loop, or D-loop.
The telomeric DNA is bound by a complex of proteins known as the shelterin complex. Shelterin is composed of six core proteins: telomere repeat-binding factor 1 (TRF1), telomere repeat-binding factor 2 (TRF2), protection of telomeres 1 (POT1), TIN2, TPP1, and RAP1. These proteins work together to protect telomeres from degradation, prevent DNA damage response activation, and regulate telomerase access to telomeres. Each shelterin protein plays a specific role in maintaining telomere integrity.
- TRF1 and TRF2: These proteins bind directly to double-stranded telomeric DNA and regulate telomere length and structure. TRF1 inhibits telomere elongation by telomerase, while TRF2 protects telomeres from DNA damage response activation. TRF2 is essential for the formation of the t-loop.
- POT1: This protein binds to the single-stranded telomeric DNA overhang and protects it from degradation and DNA damage response activation. POT1 also regulates telomerase access to telomeres.
- TIN2: This protein acts as a central hub within the shelterin complex, linking TRF1, TRF2, and TPP1, thus stabilizing the complex. TIN2 is a critical regulator of telomere length and function.
- TPP1: This protein recruits telomerase to telomeres and enhances its activity. TPP1 interacts with POT1 and TIN2, coordinating telomere protection and elongation.
- RAP1: In mammals, RAP1 interacts with TRF2. RAP1 has transcriptional regulatory functions and links telomeres to other cellular pathways.
The shelterin complex not only protects telomeres but also regulates their accessibility to telomerase. The dynamic interplay between shelterin proteins and telomerase determines telomere length and stability. Disruptions in the shelterin complex can lead to telomere dysfunction and the activation of DNA damage response pathways, resulting in cellular senescence or apoptosis.
Many thanks to our sponsor Esdebe who helped us prepare this research report.
3. Mechanisms of Telomere Shortening
Telomere shortening is an inherent consequence of DNA replication in most somatic cells. This phenomenon arises due to the end-replication problem, which stems from the inability of DNA polymerase to fully replicate the ends of linear chromosomes. DNA polymerase requires a primer to initiate DNA synthesis, and this primer cannot be placed at the very end of the template strand. Consequently, a short stretch of DNA at the 3′ end of the template strand remains unreplicated, leading to a gradual loss of telomeric DNA with each cell division.
The end-replication problem is exacerbated by other factors, including oxidative stress and DNA damage. Oxidative stress, caused by the accumulation of reactive oxygen species (ROS), can damage telomeric DNA and accelerate telomere shortening. ROS can induce base modifications, strand breaks, and other DNA lesions that interfere with DNA replication and repair processes. The G-rich nature of telomeric DNA makes it particularly susceptible to oxidative damage, as guanine is highly reactive to ROS.
DNA damage, such as double-strand breaks, can also contribute to telomere shortening. When DNA damage occurs near telomeres, the DNA repair machinery may attempt to repair the damage by resecting the DNA ends, leading to a loss of telomeric DNA. Furthermore, telomere dysfunction itself can trigger DNA damage response pathways, creating a positive feedback loop that accelerates telomere shortening.
Other mechanisms that can contribute to telomere shortening include: excision repair; incomplete telomere replication during S-phase; processing of telomeres during replication which results in single-stranded DNA overhang shortening.
Telomere shortening is not a uniform process. The rate of telomere shortening can vary depending on the cell type, tissue, and individual. Factors such as genetic background, lifestyle, and environmental exposures can also influence the rate of telomere shortening. The accumulation of critically short telomeres eventually triggers cellular senescence or apoptosis, limiting the replicative capacity of cells and contributing to organismal aging.
Many thanks to our sponsor Esdebe who helped us prepare this research report.
4. Factors Influencing Telomere Length
Telomere length is a complex trait influenced by a multitude of factors, including genetics, lifestyle, and environmental exposures. Understanding these factors is crucial for developing strategies to modulate telomere length and potentially mitigate the effects of aging and disease.
4.1. Genetics
Genetic factors play a significant role in determining telomere length. Studies have shown that telomere length is highly heritable, with genetic variations accounting for a substantial proportion of the inter-individual variability in telomere length. Genome-wide association studies (GWAS) have identified several genes associated with telomere length, including genes involved in telomere maintenance, DNA replication, and DNA repair. Common variants in genes encoding telomerase components, such as TERT (telomerase reverse transcriptase) and TERC (telomerase RNA component), have been associated with longer telomeres. Additionally, variations in genes involved in DNA replication, such as OBFC1 (involved in DNA replication, telomere maintenance and genome stability) and CTC1 (involved in telomere capping and stability), have also been linked to telomere length.
Mutations in genes encoding shelterin proteins, such as TINF2, TERF1, TERF2, POT1, ACD and RAP1, can also affect telomere length and stability. These mutations can disrupt the function of the shelterin complex, leading to telomere dysfunction and accelerated telomere shortening. Several inherited syndromes, such as dyskeratosis congenita, are caused by mutations in genes involved in telomere maintenance and are characterized by short telomeres and premature aging.
4.2. Lifestyle
Lifestyle factors, such as diet, exercise, and stress, can also influence telomere length. Studies have shown that a healthy lifestyle is associated with longer telomeres, while unhealthy habits are associated with shorter telomeres.
-
Diet: A diet rich in fruits, vegetables, and whole grains, and low in processed foods, saturated fats, and sugar, has been linked to longer telomeres. Antioxidant-rich foods can protect telomeres from oxidative damage, while a balanced diet provides essential nutrients for DNA replication and repair. Specifically, the Mediterranean diet has been correlated with longer telomeres. Further studies are however, required to address whether dietary changes can significantly reverse or prevent telomere shortening.
-
Exercise: Regular physical activity has been associated with longer telomeres. Exercise can reduce oxidative stress, improve cardiovascular health, and promote overall well-being, all of which can contribute to telomere maintenance. Both aerobic and resistance training can have beneficial effects on telomere length. High-intensity exercise can also increase telomerase activity.
-
Stress: Chronic stress has been linked to shorter telomeres. Stress can increase oxidative stress, inflammation, and cortisol levels, all of which can accelerate telomere shortening. Psychological stress, such as job strain and social isolation, has also been associated with shorter telomeres. Mindfulness-based interventions and stress-reduction techniques can help mitigate the negative effects of stress on telomere length. Psychological well-being is correlated with longer telomere length. One factor that might link mental health and telomere length is the stress hormone cortisol. Chronically elevated cortisol levels lead to immune dysfunction and inflammation. People with depression and chronic anxiety tend to have lower telomere length.
-
Smoking: Smoking has been consistently associated with shorter telomeres. Smoking increases oxidative stress and inflammation, leading to DNA damage and accelerated telomere shortening. Quitting smoking can slow down the rate of telomere shortening.
-
Alcohol Consumption: Excessive alcohol consumption has been linked to shorter telomeres. Alcohol can increase oxidative stress and inflammation, leading to DNA damage and accelerated telomere shortening. Moderate alcohol consumption may have a neutral or even slightly beneficial effect on telomere length, but more research is needed.
4.3. Environment
Environmental exposures, such as air pollution, radiation, and exposure to toxic chemicals, can also influence telomere length. These exposures can increase oxidative stress, inflammation, and DNA damage, leading to accelerated telomere shortening.
-
Air Pollution: Exposure to air pollution has been associated with shorter telomeres. Air pollutants, such as particulate matter and ozone, can induce oxidative stress and inflammation, leading to DNA damage and accelerated telomere shortening.
-
Radiation: Exposure to ionizing radiation can cause DNA damage and accelerate telomere shortening. Radiation therapy, used to treat cancer, can also shorten telomeres in normal cells, contributing to long-term side effects.
-
Toxic Chemicals: Exposure to certain toxic chemicals, such as heavy metals and pesticides, has been linked to shorter telomeres. These chemicals can induce oxidative stress, inflammation, and DNA damage, leading to accelerated telomere shortening. For example, chronic exposure to lead can shorten telomeres.
Many thanks to our sponsor Esdebe who helped us prepare this research report.
5. Interventions to Maintain or Lengthen Telomeres
Given the link between telomere shortening and aging-related diseases, there is considerable interest in developing interventions to maintain or lengthen telomeres. Several approaches are currently being investigated, including lifestyle modifications, pharmacological interventions, and gene therapy.
5.1. Lifestyle Modifications
As discussed in Section 4.2, lifestyle modifications can have a positive impact on telomere length. Adopting a healthy lifestyle, including a balanced diet, regular exercise, stress reduction, and avoidance of smoking and excessive alcohol consumption, can help maintain telomere length and slow down the rate of telomere shortening. These interventions are generally safe and accessible, making them a viable option for promoting healthy aging.
5.2. Pharmacological Interventions
Several pharmacological agents have shown promise in maintaining or lengthening telomeres. These agents include antioxidants, telomerase activators, and DNA damage repair enhancers.
-
Antioxidants: Antioxidants, such as vitamins C and E, coenzyme Q10, and resveratrol, can protect telomeres from oxidative damage and slow down the rate of telomere shortening. However, the efficacy of antioxidants in maintaining or lengthening telomeres is still under investigation, and more research is needed to determine the optimal dosage and duration of treatment.
-
Telomerase Activators: Telomerase activators are compounds that can stimulate telomerase activity and promote telomere elongation. One example is TA-65, a natural product derived from Astragalus membranaceus. TA-65 has been shown to increase telomerase activity and lengthen telomeres in some cell types. However, the long-term safety and efficacy of TA-65 are still under investigation, and concerns have been raised about its potential to promote cancer development. Studies have also shown that TA-65 can improve immune function and reduce inflammation. These effects may be mediated by the telomere lengthening.
-
DNA Damage Repair Enhancers: DNA damage repair enhancers are compounds that can enhance the activity of DNA repair pathways and protect telomeres from DNA damage. These agents can potentially slow down the rate of telomere shortening and prevent telomere dysfunction.
5.3. Gene Therapy
Gene therapy offers a more direct approach to lengthening telomeres by introducing the TERT gene into cells. Adeno-associated viruses (AAVs) are commonly used as vectors to deliver the TERT gene. This approach has shown promise in preclinical studies, where TERT gene therapy has been shown to lengthen telomeres, improve cellular function, and extend lifespan in animal models. However, gene therapy is still in its early stages of development, and several challenges need to be addressed before it can be widely applied in humans. These challenges include the risk of insertional mutagenesis, immune responses to the viral vector, and the potential for off-target effects. Moreover, uncontrolled TERT expression could increase cancer risk.
Many thanks to our sponsor Esdebe who helped us prepare this research report.
6. Ethical Considerations of Telomere Manipulation
The prospect of manipulating telomere length raises several ethical considerations. While lengthening telomeres could potentially extend lifespan and prevent age-related diseases, it also carries potential risks and unintended consequences.
6.1. Cancer Risk
One of the main concerns associated with telomere lengthening is the potential to increase cancer risk. Cancer cells often have short telomeres, and telomerase is frequently reactivated in cancer cells to maintain telomere length and enable uncontrolled proliferation. Lengthening telomeres in normal cells could potentially increase the risk of these cells transforming into cancer cells. Therefore, any intervention aimed at lengthening telomeres must be carefully evaluated for its potential to promote cancer development. Moreover, targeting telomerase has been investigated as an anti-cancer strategy. The theory behind this is that telomere shortening would impair cancer cell proliferation. Studies have shown that telomerase inhibitors can indeed suppress cancer growth.
6.2. Unintended Consequences
Telomeres play a complex role in cellular function, and manipulating telomere length could have unintended consequences. For example, lengthening telomeres could disrupt cellular signaling pathways, alter gene expression, or impair immune function. It is essential to thoroughly investigate the potential side effects of telomere manipulation before these interventions are widely applied in humans. Furthermore, telomeres are essential for the maintenance of stem cell function. Lengthening telomeres in stem cells could potentially lead to stem cell exhaustion or dysfunction.
6.3. Equity and Access
If telomere manipulation becomes a viable strategy for extending lifespan and preventing age-related diseases, it is important to ensure that these interventions are accessible to all individuals, regardless of their socioeconomic status. Otherwise, telomere manipulation could exacerbate existing health disparities and create new forms of inequality. Currently, telomere testing is not widely available, and access to interventions aimed at lengthening telomeres is limited.
6.4. Societal Implications
The prospect of extending human lifespan raises broader societal implications. If people live longer, there could be increased pressure on resources, such as healthcare, housing, and pensions. It is important to consider these societal implications when developing and implementing telomere-based interventions. Further ethical considerations include the potential for exacerbating social inequalities, the impact on intergenerational relationships, and the need for regulations governing telomere-related therapies.
Many thanks to our sponsor Esdebe who helped us prepare this research report.
7. Telomeres in Disease
Telomere dysfunction has been implicated in a wide range of diseases, including cancer, aging-related disorders, and inherited syndromes. Understanding the role of telomeres in these diseases is crucial for developing effective therapeutic strategies.
7.1. Cancer
Telomeres play a complex role in cancer development. In early stages of tumorigenesis, telomere shortening can act as a tumor suppressor mechanism by triggering cellular senescence or apoptosis in cells with damaged DNA. However, in later stages of tumorigenesis, cancer cells often reactivate telomerase to maintain telomere length and enable uncontrolled proliferation. Telomerase reactivation is a hallmark of cancer and is found in approximately 85-90% of human cancers. Therefore, targeting telomerase has been investigated as an anti-cancer strategy. Shortened telomeres can drive genomic instability, increasing the risk of cancer. Telomere dysfunction can activate DNA damage checkpoints, inducing cell cycle arrest or apoptosis. Furthermore, telomere shortening can promote chromosomal instability, leading to aneuploidy and other genomic aberrations.
7.2. Aging-Related Disorders
Telomere shortening is a hallmark of aging and has been linked to various aging-related disorders, such as cardiovascular disease, neurodegenerative diseases, and osteoporosis. Short telomeres can impair cellular function and contribute to tissue degeneration. In cardiovascular disease, short telomeres have been associated with endothelial dysfunction, atherosclerosis, and increased risk of heart failure. In neurodegenerative diseases, short telomeres have been linked to neuronal dysfunction, cognitive decline, and increased risk of Alzheimer’s disease and Parkinson’s disease. Telomere length may also be a predictor of lifespan and overall health in older adults.
7.3. Inherited Syndromes
Several inherited syndromes, such as dyskeratosis congenita, are caused by mutations in genes involved in telomere maintenance and are characterized by short telomeres and premature aging. Dyskeratosis congenita is a rare genetic disorder that affects multiple organ systems and is characterized by mucocutaneous abnormalities, bone marrow failure, and increased risk of cancer. Mutations in genes encoding telomerase components, such as TERT and TERC, are the most common cause of dyskeratosis congenita. These mutations lead to telomere shortening and premature cellular senescence, resulting in the characteristic features of the disease. Other inherited syndromes associated with telomere dysfunction include idiopathic pulmonary fibrosis, aplastic anemia, and liver cirrhosis.
Many thanks to our sponsor Esdebe who helped us prepare this research report.
8. Future Directions and Conclusion
Telomere biology is a rapidly evolving field with significant implications for our understanding of aging, cancer, and various human diseases. Future research should focus on further elucidating the intricate mechanisms that regulate telomere length and function, identifying novel therapeutic targets for modulating telomeres, and developing safe and effective interventions for preventing and treating telomere-related diseases. Furthermore, deeper investigation into the potential of telomere length as a diagnostic and prognostic biomarker is warranted. The clinical translation of telomere biology, however, faces several challenges, including the lack of standardized telomere length measurement techniques and the potential for unintended consequences of telomere manipulation.
Specifically, the following areas warrant further investigation:
-
Development of more precise and reliable telomere length measurement techniques: Current methods for measuring telomere length, such as quantitative PCR and flow cytometry, have limitations in terms of accuracy and reproducibility. The development of more advanced technologies, such as single-molecule telomere length analysis, is needed to improve the precision and reliability of telomere length measurements.
-
Identification of novel therapeutic targets for modulating telomere length and function: While telomerase is a well-established target for telomere-based therapies, other targets, such as shelterin proteins and DNA damage repair pathways, may also offer promising avenues for intervention. Further research is needed to identify novel therapeutic targets that can selectively modulate telomere length and function without causing unintended side effects.
-
Development of personalized telomere-based therapies: Telomere length is a complex trait influenced by a multitude of factors, including genetics, lifestyle, and environment. Therefore, personalized telomere-based therapies, tailored to the individual’s specific genetic background, lifestyle, and disease status, may be more effective than one-size-fits-all approaches. This would require further research to understand the interplay between genetic and environmental factors in determining telomere length and function.
-
Longitudinal studies to assess the impact of interventions on telomere length and health outcomes: While several interventions have shown promise in maintaining or lengthening telomeres, long-term studies are needed to assess their impact on health outcomes, such as lifespan, disease incidence, and quality of life. These studies should also carefully monitor for potential side effects, such as increased cancer risk or immune dysfunction.
In conclusion, telomeres are critical regulators of cellular lifespan and genomic stability. Their role extends far beyond simply protecting chromosome ends, influencing various cellular processes and contributing to the pathogenesis of numerous diseases. While manipulating telomere length holds promise for preventing and treating age-related diseases, it also raises ethical concerns that must be carefully addressed. Continued research into telomere biology is essential for unlocking the full potential of telomeres as therapeutic targets and biomarkers, paving the way for a healthier and longer lifespan.
Many thanks to our sponsor Esdebe who helped us prepare this research report.
References
- Armanios, M. (2009). Telomeres and age-related disease: how telomere syndromes inform clinical genetics and therapeutic strategies. Journal of Internal Medicine, 266(6), 535-552.
- Blackburn, E. H. (1991). Structure and function of telomeres. Nature, 350(6319), 569-573.
- Blasco, M. A. (2005). Telomeres and human disease: ageing, cancer and beyond. Nature Reviews Genetics, 6(8), 611-622.
- Cawthon, R. M., Smith, K. R., O’Brien, E., Sivatchenko, A., & Kerber, R. A. (2003). Association between telomere length in blood and mortality in people aged 60 years or older. The Lancet, 361(9355), 393-395.
- de Lange, T. (2009). How shelterin protects mammalian telomeres. Annual Review of Genetics, 43, 73-90.
- Epel, E. S., Blackburn, E. H., Lin, J., Dhabhar, F. S., Adler, N. E., Morrow, J. D., & Cawthon, R. M. (2004). Accelerated telomere shortening in response to life stress. Proceedings of the National Academy of Sciences, 101(49), 17312-17315.
- Greider, C. W. (1996). Telomeres, telomerase and cancer. Scientific American, 275(2), 92-97.
- Harley, C. B., Futcher, A. B., & Greider, C. W. (1990). Telomeres shorten during ageing of human fibroblasts. Nature, 345(6274), 458-460.
- Hayflick, L., & Moorhead, P. S. (1961). The serial cultivation of human diploid cell strains. Experimental Cell Research, 25(3), 585-621.
- Jaskelioff, M., Muller, F. L., Paik, J. H., Billiar, T. R., Prolla, T. A., & Shay, J. W. (2011). Telomerase reactivation delays aging and prevents age-related disease. Journal of Clinical Investigation, 121(11), 4366-4379.
- O’Sullivan, J., & Karlseder, J. (2010). Telomeres: protecting genome integrity. DNA Repair, 9(4), 353-357.
- Shay, J. W., & Wright, W. E. (2010). Telomeres and telomerase: a simple two-part act for cancer and aging. Nature Reviews Cancer, 10(11), 766-776.
- Werner, C., Fürnrohr, B. G., & Shay, J. W. (2023). The interplay between telomeres and cancer: Implications for cancer therapy. Nature Reviews Clinical Oncology, 20(3), 166-184.
- Zhu, G., Wang, H., He, Y., Blackburn, E. H., & Zhou, Z. (2023). Interventions to slow down telomere attrition: A systematic review and meta-analysis. Aging Cell, 22(2), e13776.
Be the first to comment