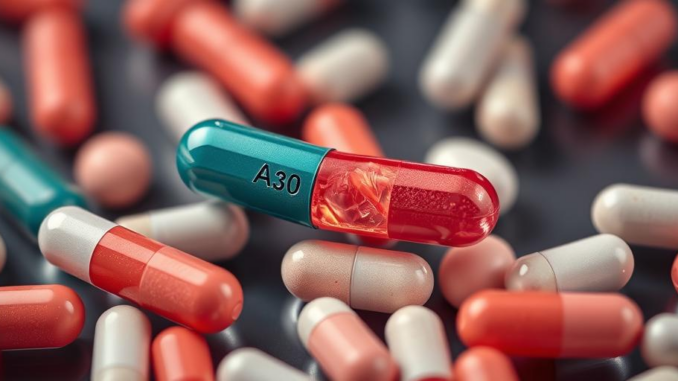
Abstract
The rise of antibiotic resistance poses a significant threat to global public health. This report provides a comprehensive overview of the antibiotic landscape, encompassing the history of antibiotic discovery and use, the mechanisms of antibiotic action and resistance, the current state of antibiotic development, economic and regulatory factors influencing research, and potential alternative therapeutic strategies. It further examines the crucial role of antibiotic stewardship in mitigating resistance and preserving the efficacy of existing and future antimicrobial agents. The report synthesizes current research and expert perspectives to highlight the complexities and challenges associated with addressing the global antibiotic resistance crisis, ultimately advocating for a multi-faceted approach involving scientific innovation, responsible usage, and policy changes.
Many thanks to our sponsor Esdebe who helped us prepare this research report.
1. Introduction
The discovery of penicillin by Alexander Fleming in 1928 marked a watershed moment in medical history, ushering in the era of antibiotics and transforming the treatment of infectious diseases. These “wonder drugs” revolutionized healthcare, significantly reducing morbidity and mortality associated with bacterial infections. However, the widespread and often indiscriminate use of antibiotics has led to the emergence and proliferation of antibiotic-resistant bacteria, a global health crisis that threatens to reverse the progress made in combating infectious diseases. This report provides a comprehensive overview of the antibiotic landscape, addressing the historical context, mechanisms of action and resistance, the current state of antibiotic development, economic considerations, alternative therapies, and the imperative for antibiotic stewardship. The goal is to provide a robust understanding of the multifaceted challenges and potential solutions in this critical area of public health.
Many thanks to our sponsor Esdebe who helped us prepare this research report.
2. A Historical Perspective on Antibiotic Development and Use
The ‘golden age’ of antibiotic discovery, spanning from the 1940s to the 1960s, witnessed the rapid identification and development of numerous antibiotic classes, including aminoglycosides, tetracyclines, macrolides, and cephalosporins. These compounds were primarily derived from natural sources, particularly soil bacteria and fungi. The ease and effectiveness with which bacterial infections could be treated led to a surge in antibiotic use, not only in human medicine but also in agriculture as growth promoters in livestock. This widespread use, coupled with incomplete courses of antibiotics and self-medication, created selective pressure favoring the survival and proliferation of resistant bacteria.
Over time, the rate of new antibiotic approvals has declined significantly, creating a widening gap between the emergence of resistant bacteria and the availability of effective treatment options. This decline is attributable to a combination of factors, including the scientific challenges associated with discovering novel antibiotics, the economic disincentives for pharmaceutical companies to invest in antibiotic research, and increasingly stringent regulatory requirements. The consequences of this ‘discovery void’ are profound, threatening to render many common infections untreatable and jeopardizing the success of modern medical procedures such as surgery, transplantation, and cancer chemotherapy.
Many thanks to our sponsor Esdebe who helped us prepare this research report.
3. Mechanisms of Antibiotic Action and Resistance
Antibiotics exert their antimicrobial effects through a variety of mechanisms, targeting essential bacterial processes such as cell wall synthesis, protein synthesis, DNA replication, and metabolic pathways. These mechanisms provide a basis for classifying antibiotics into different groups:
- Cell Wall Synthesis Inhibitors: Beta-lactams (penicillins, cephalosporins, carbapenems) and glycopeptides (vancomycin) interfere with the synthesis of peptidoglycan, a critical component of the bacterial cell wall. Resistance often arises through the production of beta-lactamases (enzymes that inactivate beta-lactams) or modifications in peptidoglycan structure that reduce the affinity for glycopeptides.
- Protein Synthesis Inhibitors: Aminoglycosides, tetracyclines, macrolides, and chloramphenicol bind to bacterial ribosomes, disrupting protein synthesis. Resistance mechanisms include enzymatic modification of the antibiotic, mutations in ribosomal RNA, and efflux pumps that actively expel the antibiotic from the bacterial cell.
- DNA Replication Inhibitors: Quinolones (ciprofloxacin, levofloxacin) inhibit bacterial DNA gyrase and topoisomerase IV, enzymes essential for DNA replication and repair. Resistance commonly develops through mutations in these target enzymes or increased expression of efflux pumps.
- Metabolic Pathway Inhibitors: Sulfonamides and trimethoprim inhibit enzymes involved in the synthesis of folic acid, a precursor to essential cofactors. Resistance can occur through mutations in the target enzymes or the acquisition of genes encoding alternative enzymes that are less sensitive to the antibiotics.
Bacteria employ various mechanisms to evade the effects of antibiotics. These mechanisms can be broadly categorized as follows:
- Enzymatic Inactivation: Bacteria produce enzymes that modify or degrade the antibiotic, rendering it inactive. Examples include beta-lactamases, aminoglycoside-modifying enzymes, and chloramphenicol acetyltransferases.
- Target Modification: Mutations in the target site of the antibiotic can reduce its binding affinity and effectiveness. Examples include mutations in penicillin-binding proteins (PBPs) in beta-lactam resistance and mutations in ribosomal RNA in macrolide resistance.
- Efflux Pumps: Bacteria express membrane-bound pumps that actively transport antibiotics out of the cell, reducing their intracellular concentration. Efflux pumps can confer resistance to multiple antibiotics simultaneously.
- Reduced Permeability: Changes in the bacterial cell envelope can reduce the influx of antibiotics into the cell. This can occur through mutations in porins (membrane channels) or alterations in the lipid composition of the cell membrane.
- Bypass Pathways: Some bacteria can circumvent the effects of antibiotics by utilizing alternative metabolic pathways or acquiring genes that encode resistant enzymes.
Horizontal gene transfer (HGT) plays a crucial role in the spread of antibiotic resistance genes. Resistance genes can be transferred between bacteria through plasmids, transposons, and bacteriophages, facilitating the rapid dissemination of resistance across different bacterial species and even between different genera. The widespread use of antibiotics in human medicine and agriculture has created a selective pressure that favors the survival and proliferation of bacteria carrying resistance genes, accelerating the spread of resistance.
Many thanks to our sponsor Esdebe who helped us prepare this research report.
4. The Current State of Antibiotic Development: Challenges and Opportunities
The development of new antibiotics has slowed considerably in recent decades, creating a critical need for novel antimicrobial agents to combat the growing threat of antibiotic resistance. Several factors contribute to this slowdown, including scientific challenges, economic disincentives, and regulatory hurdles.
- Scientific Challenges: Identifying novel antibiotic targets and developing compounds that effectively inhibit these targets while minimizing toxicity to human cells is a complex and challenging process. Many potential antibiotic targets are already exploited by existing antibiotics, making it difficult to find new targets that are both essential for bacterial survival and amenable to drug development. Furthermore, the increasing prevalence of multidrug-resistant bacteria makes it necessary to develop antibiotics that are effective against a broad range of resistant strains.
- Economic Disincentives: Antibiotics are often used for relatively short durations, and their use is typically restricted to specific patient populations. This limits the potential market for new antibiotics, making it difficult for pharmaceutical companies to recoup their investment in research and development. Furthermore, the development of resistance to new antibiotics can further reduce their market potential.
- Regulatory Hurdles: The regulatory approval process for new antibiotics is often lengthy and complex, requiring extensive clinical trials to demonstrate safety and efficacy. The high cost and lengthy timeline associated with clinical trials can further discourage pharmaceutical companies from investing in antibiotic development.
Despite these challenges, there are several promising approaches to antibiotic development currently being pursued:
- Targeting Novel Bacterial Processes: Research is focused on identifying new antibiotic targets that are essential for bacterial survival and have not yet been exploited by existing antibiotics. These targets may include bacterial virulence factors, metabolic pathways, or regulatory mechanisms.
- Developing New Classes of Antibiotics: Researchers are exploring new classes of antibiotics that have novel mechanisms of action and are effective against resistant bacteria. Examples include lipopeptides (daptomycin), oxazolidinones (linezolid), and glycylcyclines (tigecycline). These newer drugs are often reserved as last resort treatments.
- Optimizing Existing Antibiotics: Efforts are underway to improve the efficacy and safety of existing antibiotics through chemical modifications, formulation improvements, and combination therapies. Combining existing antibiotics can broaden their spectrum of activity and reduce the risk of resistance development.
- Harnessing Host Defenses: Approaches that enhance the host immune response to bacterial infections are being explored as potential alternatives to traditional antibiotics. These approaches may include the use of immunomodulatory agents or vaccines.
- Phage Therapy: Bacteriophages (viruses that infect bacteria) are being investigated as a potential alternative to antibiotics. Phage therapy has the potential to be highly specific and effective against resistant bacteria, but further research is needed to optimize phage selection and delivery.
- Antimicrobial Peptides: These naturally occurring peptides have broad-spectrum antimicrobial activity and are being developed as potential therapeutic agents. Antimicrobial peptides can disrupt bacterial cell membranes, inhibit bacterial enzymes, or modulate the host immune response.
Many thanks to our sponsor Esdebe who helped us prepare this research report.
5. Economic and Regulatory Factors Influencing Antibiotic Research and Development
The economic landscape surrounding antibiotic research and development presents a significant barrier to innovation. The short duration of antibiotic treatment, the potential for rapid resistance development, and the pressure to reserve novel antibiotics for last-resort use create limited market opportunities for pharmaceutical companies. This, in turn, makes it difficult to justify the substantial investment required for drug discovery and clinical trials. The “market failure” in antibiotic development has prompted calls for government intervention and policy changes to incentivize research and ensure access to new antibiotics.
Several economic models have been proposed to address this market failure:
- Push Incentives: These incentives aim to reduce the upfront costs of antibiotic development. Examples include direct funding for research grants, tax credits for research expenses, and public-private partnerships. The CARB-X (Combating Antibiotic-Resistant Bacteria Biopharmaceutical Accelerator) initiative is a prime example of a push incentive that provides funding and technical assistance to companies developing novel antibiotics.
- Pull Incentives: These incentives aim to increase the potential returns on investment for successful antibiotic developers. Examples include market exclusivity extensions, transferable exclusivity vouchers (TEVs), and revenue guarantees. TEVs allow companies to extend the market exclusivity of another drug in their portfolio, providing a financial reward for developing a new antibiotic. Revenue guarantees provide a guaranteed minimum revenue stream for new antibiotics, reducing the financial risk for pharmaceutical companies.
Regulatory pathways also play a critical role in antibiotic development. Streamlining the regulatory approval process for new antibiotics can reduce the time and cost associated with clinical trials. The FDA’s Qualified Infectious Disease Product (QIDP) designation provides certain incentives for the development of new antibiotics targeting serious or life-threatening infections. These incentives include priority review and extended market exclusivity.
International collaborations and partnerships are essential for addressing the global antibiotic resistance crisis. Organizations such as the World Health Organization (WHO) and the European Medicines Agency (EMA) are working to coordinate efforts to monitor antibiotic resistance, promote responsible antibiotic use, and support the development of new antibiotics.
Many thanks to our sponsor Esdebe who helped us prepare this research report.
6. Alternative Therapies to Antibiotics: A New Frontier in Infection Control
Given the pressing need to reduce reliance on traditional antibiotics, alternative therapeutic strategies are gaining increasing attention. These approaches aim to combat bacterial infections through mechanisms distinct from those employed by conventional antibiotics, thereby circumventing existing resistance mechanisms. Several promising alternative therapies are currently under investigation:
- Phage Therapy: Bacteriophages, viruses that specifically target and kill bacteria, represent a potential alternative to antibiotics. Phage therapy offers the advantage of being highly specific, minimizing disruption to the host microbiome. However, challenges remain in identifying and characterizing appropriate phages, optimizing phage delivery, and addressing potential immune responses.
- Antimicrobial Peptides (AMPs): AMPs are naturally occurring peptides with broad-spectrum antimicrobial activity. They typically disrupt bacterial cell membranes, leading to cell death. AMPs are being investigated as potential therapeutic agents for a variety of infections, including skin infections, wound infections, and respiratory infections. Challenges include optimizing AMP stability and bioavailability, as well as minimizing potential toxicity.
- Immunomodulatory Therapies: These therapies aim to enhance the host immune response to bacterial infections. Examples include cytokines, antibodies, and Toll-like receptor agonists. Immunomodulatory therapies can help to clear bacterial infections and prevent the development of resistance.
- Probiotics and Prebiotics: Probiotics are live microorganisms that confer a health benefit to the host, while prebiotics are non-digestible food ingredients that promote the growth of beneficial bacteria. Probiotics and prebiotics can help to restore the balance of the gut microbiome, which can be disrupted by antibiotic use. This can reduce the risk of antibiotic-associated diarrhea and other complications.
- CRISPR-Cas Systems: CRISPR-Cas systems are bacterial defense mechanisms that can be engineered to target and kill specific bacteria. CRISPR-Cas technology is being explored as a potential therapeutic agent for targeted eradication of antibiotic-resistant bacteria.
- Virulence Inhibitors: Rather than killing bacteria, virulence inhibitors target bacterial virulence factors, such as toxins and adhesins, that are essential for causing disease. By inhibiting virulence, these compounds can reduce the severity of infection and allow the host immune system to clear the bacteria.
Many thanks to our sponsor Esdebe who helped us prepare this research report.
7. Antibiotic Stewardship: A Cornerstone of Resistance Mitigation
Antibiotic stewardship programs are essential for promoting responsible antibiotic use and mitigating the development and spread of antibiotic resistance. These programs aim to optimize antibiotic prescribing practices, reduce unnecessary antibiotic use, and improve patient outcomes. Effective antibiotic stewardship programs typically involve a multidisciplinary team, including physicians, pharmacists, nurses, and infection control specialists.
Key components of antibiotic stewardship programs include:
- Education and Training: Providing education and training to healthcare professionals on appropriate antibiotic prescribing practices, antibiotic resistance, and the importance of antibiotic stewardship.
- Prescription Review and Feedback: Regularly reviewing antibiotic prescriptions and providing feedback to prescribers on their prescribing practices.
- Formulary Restriction: Restricting the use of certain antibiotics to specific indications or prescribers.
- Clinical Practice Guidelines: Developing and implementing clinical practice guidelines for the management of common infections.
- Antimicrobial Susceptibility Testing: Ensuring that appropriate antimicrobial susceptibility testing is performed to guide antibiotic selection.
- Public Awareness Campaigns: Raising public awareness about antibiotic resistance and the importance of responsible antibiotic use.
The implementation of antibiotic stewardship programs has been shown to reduce antibiotic use, decrease the incidence of antibiotic-resistant infections, and improve patient outcomes. Successful stewardship programs require a commitment from healthcare leaders, ongoing monitoring and evaluation, and a collaborative approach involving all stakeholders.
Many thanks to our sponsor Esdebe who helped us prepare this research report.
8. The Role of Artificial Intelligence in Combating Antibiotic Resistance
Artificial intelligence (AI) and machine learning (ML) are increasingly being used to address the challenges posed by antibiotic resistance. AI-powered tools can analyze large datasets of genomic, clinical, and epidemiological data to identify patterns and predict antibiotic resistance. These tools can be used to:
- Predict Antibiotic Resistance: ML models can be trained on historical data to predict the likelihood of antibiotic resistance in specific bacterial isolates. This can help clinicians to select appropriate antibiotics for empirical therapy and avoid the use of ineffective antibiotics.
- Identify Novel Antibiotic Targets: AI can be used to analyze bacterial genomes and proteomes to identify novel antibiotic targets. This can accelerate the discovery of new antibiotics and provide new avenues for combating resistance.
- Optimize Antibiotic Stewardship: AI can be used to analyze antibiotic prescribing data to identify areas where antibiotic use can be optimized. This can help to reduce unnecessary antibiotic use and promote responsible antibiotic stewardship.
- Develop Personalized Treatment Strategies: AI can be used to analyze patient-specific data to develop personalized treatment strategies for bacterial infections. This can help to improve patient outcomes and reduce the risk of resistance development.
While AI holds great promise for combating antibiotic resistance, it is important to acknowledge the limitations of these technologies. AI models are only as good as the data they are trained on, and biases in the data can lead to inaccurate predictions. Furthermore, AI models require careful validation and monitoring to ensure that they are performing as expected.
Many thanks to our sponsor Esdebe who helped us prepare this research report.
9. Conclusion
The global antibiotic resistance crisis poses a significant threat to public health and requires a multi-faceted approach involving scientific innovation, responsible antibiotic use, and policy changes. The development of new antibiotics is essential, but it is not the sole solution. Alternative therapies, such as phage therapy and immunomodulatory therapies, offer promising avenues for combating bacterial infections. Antibiotic stewardship programs are crucial for promoting responsible antibiotic use and mitigating the spread of resistance. Furthermore, Artificial Intelligence can be used to improve diagnostic tools and predict resistance. A concerted effort involving researchers, clinicians, policymakers, and the public is needed to address this complex challenge and ensure the continued effectiveness of antibiotics for future generations.
Many thanks to our sponsor Esdebe who helped us prepare this research report.
References
- Alekshun, M. N., & Levy, S. B. (2007). Antimicrobial resistance: mechanisms of resistance and adaptive responses. Chemical reviews, 107(4), 1548-1585.
- Boucher, H. W., Talbot, G. H., Bradley, J. S., Edwards, J. E., Gilbert, D., Rice, L. B., … & Spellberg, B. (2009). Bad bugs, no drugs: no ESKAPE! An update from the Infectious Diseases Society of America. Clinical infectious diseases, 48(1), 1-12.
- Fair, R. J., & Tor, Y. (2014). Antibiotics and bacterial resistance in the 21st century. Perspectives in medicinal chemistry, 6, PMC-S14425.
- Fischbach, M. A., & Walsh, C. T. (2009). Antibiotics for emerging pathogens. Science, 325(5944), 1089-1093.
- Lerminiaux, W. J., & Cameron, A. D. (2019). Horizontal transfer of antibiotic resistance genes in clinical settings. Canadian journal of microbiology, 65(1), 34-44.
- Nathan, C., & Cars, O. (2014). Antibiotic resistance—problems, causes, and solutions. BMJ, 348.
- O’Neill, J. (2016). Tackling drug-resistant infections globally: final report and recommendations. Review on Antimicrobial Resistance.
- Ventola, C. L. (2015). The antibiotic resistance crisis: part 1: causes and threats. Pharmacy and therapeutics, 40(4), 277.
- WHO. (2014). Antimicrobial resistance: global report on surveillance. World Health Organization.
- Zaman, S., Hussain, M. A., Nye, R., Mehta, V., Mamun, Y., & Hossain, N. (2017). A review on antibiotic resistance: alarm bells are ringing. Cureus, 9(6).
- Stokes, J. M., Yang, K., Swanson, K., Jin, W., Cubillos-Ruiz, A., Donghia, N. M., … & Collins, J. J. (2020). A deep learning approach to antibiotic discovery. Cell, 180(4), 688-702.
- Silverman, J. A., & Vakulenko, S. B. (2021). Insights into mechanisms of bacterial resistance to antibiotics. Antibiotics, 10(6), 642.
AI predicting antibiotic resistance? Finally, a computer that understands my insistence on finishing my antibiotics, even when I feel like a superhero after three days. Maybe it can also predict when I’ll finally fold my laundry.
That’s a great point! The potential for AI to predict behaviors around health, like finishing medications, is fascinating. If it can nudge us towards better habits, that’s a big win. Though, if it starts nagging us about laundry, I’m not sure I’m ready for that level of AI intervention just yet!
Editor: MedTechNews.Uk
Thank you to our Sponsor Esdebe
Given AI’s promise in predicting resistance, how accurate are current models in diverse real-world clinical settings, and what steps are being taken to address potential biases within the training data?