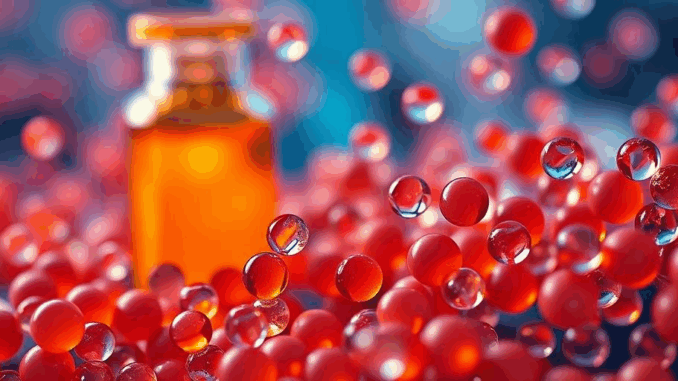
Abstract
Biologics represent a paradigm shift in pharmaceutical development, offering targeted therapies for a wide range of diseases, including cancers, autoimmune disorders, and infectious diseases. Unlike traditional small-molecule drugs, biologics are complex molecules derived from living organisms, presenting unique challenges and opportunities in manufacturing, regulation, and market access. This report examines the evolving landscape of biologics, exploring their scientific underpinnings, developmental complexities, market dynamics, and regulatory frameworks. We delve into the intricacies of biologics development, highlighting the high costs and risks involved, and analyze the impact of intellectual property protection and regulatory exclusivity on innovation. Furthermore, we critically assess the debate surrounding biosimilars, considering their potential to improve patient access and reduce healthcare costs while safeguarding innovation incentives. Finally, we explore emerging trends in biologics development, including personalized medicine, gene therapies, and the use of artificial intelligence in drug discovery and development, and discuss their potential to transform healthcare in the coming decades.
Many thanks to our sponsor Esdebe who helped us prepare this research report.
1. Introduction
The pharmaceutical industry has undergone a profound transformation in recent decades, driven by advancements in biotechnology and our growing understanding of molecular biology. This transformation has led to the emergence of biologics, a class of therapeutics that are fundamentally different from traditional small-molecule drugs. Biologics, also known as biopharmaceuticals or biological products, are derived from living organisms, such as bacteria, yeast, or mammalian cells. They encompass a wide range of therapeutic modalities, including monoclonal antibodies (mAbs), therapeutic proteins, vaccines, gene therapies, and cell therapies. Unlike small-molecule drugs, which are typically synthesized chemically and have a well-defined structure, biologics are complex molecules with heterogeneous structures and are often glycosylated or otherwise modified. This complexity presents unique challenges in manufacturing, characterization, and regulation.
The rise of biologics has revolutionized the treatment of many diseases, offering targeted therapies with improved efficacy and reduced side effects compared to traditional treatments. For example, monoclonal antibodies have transformed the treatment of cancers, autoimmune disorders, and infectious diseases. Gene therapies hold the promise of curing genetic diseases by correcting or replacing defective genes. Cell therapies offer the potential to regenerate damaged tissues and organs. However, the development and manufacturing of biologics are significantly more complex and expensive than those of small-molecule drugs. This complexity translates into higher prices, which can limit patient access and strain healthcare budgets. Furthermore, the longer development timelines and higher failure rates associated with biologics pose significant financial risks for pharmaceutical companies. As a result, the regulatory framework for biologics must strike a delicate balance between promoting innovation and ensuring patient safety and affordability.
Many thanks to our sponsor Esdebe who helped us prepare this research report.
2. The Science Behind Biologics
Biologics represent a diverse class of therapeutic products, each with its unique mechanism of action and application. Understanding the scientific principles underlying biologics is crucial for appreciating their potential and limitations. This section provides an overview of the major types of biologics and their mechanisms of action.
2.1. Monoclonal Antibodies (mAbs)
Monoclonal antibodies are among the most successful and widely used biologics. They are antibodies that are produced by a single clone of immune cells and are designed to bind to a specific target molecule, such as a protein on the surface of a cancer cell or an inflammatory cytokine. mAbs can exert their therapeutic effects through various mechanisms, including:
- Neutralization: Blocking the activity of a target molecule by preventing it from binding to its receptor.
- Antibody-dependent cell-mediated cytotoxicity (ADCC): Recruiting immune cells to kill target cells.
- Complement-dependent cytotoxicity (CDC): Activating the complement system to destroy target cells.
- Direct signaling: Inducing apoptosis (programmed cell death) or other cellular responses in target cells.
Examples of successful mAbs include adalimumab (Humira) for rheumatoid arthritis, trastuzumab (Herceptin) for breast cancer, and nivolumab (Opdivo) for melanoma.
2.2. Therapeutic Proteins
Therapeutic proteins are recombinant proteins that are produced in living cells and are used to treat a variety of diseases. They can be used to replace missing or deficient proteins, such as insulin for diabetes, or to deliver a therapeutic effect, such as erythropoietin (EPO) for anemia. Therapeutic proteins are typically produced in mammalian cells, such as Chinese hamster ovary (CHO) cells, because these cells are capable of performing the post-translational modifications, such as glycosylation, that are necessary for proper protein folding and function. The glycosylation pattern of a therapeutic protein can significantly affect its efficacy, safety, and immunogenicity.
2.3. Vaccines
Vaccines are biological preparations that stimulate the immune system to produce antibodies and cellular immunity against a specific pathogen. Traditional vaccines contain inactivated or attenuated pathogens, while newer vaccines may contain only specific antigens or genetic material from the pathogen. Biologics have revolutionized vaccine development, enabling the creation of vaccines against previously intractable diseases, such as human papillomavirus (HPV) and hepatitis B. mRNA vaccines, which use messenger RNA to instruct cells to produce viral proteins, have emerged as a powerful platform for developing vaccines against infectious diseases, as demonstrated by the rapid development of COVID-19 vaccines.
2.4. Gene Therapies
Gene therapies are a revolutionary approach to treating genetic diseases by correcting or replacing defective genes. Gene therapies can be delivered using viral vectors, such as adeno-associated viruses (AAVs), or non-viral vectors, such as plasmids or liposomes. Gene therapies have shown remarkable success in treating rare genetic diseases, such as spinal muscular atrophy (SMA) and inherited blindness. However, the development of gene therapies is challenging due to the difficulty of delivering the therapeutic gene to the target cells and the potential for off-target effects and immunogenicity.
2.5. Cell Therapies
Cell therapies involve the use of living cells to treat diseases. Cell therapies can be autologous, meaning that the cells are derived from the patient, or allogeneic, meaning that the cells are derived from a donor. Cell therapies have shown promise in treating cancers, autoimmune disorders, and regenerative medicine. For example, CAR-T cell therapy, which involves engineering a patient’s own immune cells to recognize and kill cancer cells, has revolutionized the treatment of certain types of leukemia and lymphoma. However, the development of cell therapies is challenging due to the difficulty of manufacturing and delivering the cells and the potential for immune rejection.
Many thanks to our sponsor Esdebe who helped us prepare this research report.
3. Biologics Development: Costs, Risks, and Innovation
The development of biologics is a complex and expensive undertaking, characterized by high costs, long timelines, and significant risks. This section examines the various stages of biologics development, highlighting the key challenges and opportunities.
3.1. Discovery and Preclinical Development
The discovery and preclinical development of biologics involve identifying a target molecule, developing a therapeutic candidate, and conducting preclinical studies to assess its safety and efficacy. This stage typically involves several years of research and development and can cost hundreds of millions of dollars. Key challenges include:
- Target identification and validation: Identifying a target molecule that is relevant to the disease and can be effectively modulated by a biologic.
- Therapeutic candidate development: Developing a therapeutic candidate that binds to the target molecule with high affinity and specificity and has desirable pharmacological properties.
- Preclinical studies: Conducting preclinical studies in cell cultures and animal models to assess the safety, efficacy, and pharmacokinetics of the therapeutic candidate.
3.2. Clinical Development
Clinical development involves conducting clinical trials in humans to assess the safety and efficacy of the biologic. Clinical trials are typically conducted in three phases:
- Phase 1: Assessing the safety and tolerability of the biologic in a small group of healthy volunteers or patients.
- Phase 2: Assessing the efficacy of the biologic in a larger group of patients with the target disease.
- Phase 3: Confirming the efficacy of the biologic in a large, randomized, controlled trial.
Clinical trials are expensive and time-consuming, often taking several years and costing hundreds of millions of dollars. The failure rate in clinical development is high, with many biologics failing to demonstrate sufficient efficacy or safety. This high failure rate contributes to the high cost of biologics development.
3.3. Manufacturing
The manufacturing of biologics is a complex and highly regulated process. Biologics are typically produced in living cells, such as CHO cells or bacteria, which require specialized equipment and expertise. The manufacturing process must be carefully controlled to ensure the quality, purity, and consistency of the product. Key challenges include:
- Cell line development: Developing a cell line that produces the biologic with high yield and quality.
- Upstream processing: Cultivating the cells in bioreactors and optimizing the culture conditions to maximize protein production.
- Downstream processing: Purifying the biologic from the cell culture medium using a series of chromatographic and filtration steps.
- Formulation and fill-finish: Formulating the biologic into a stable and easy-to-administer dosage form and filling it into vials or syringes.
The manufacturing of biologics is subject to strict regulatory requirements, including Good Manufacturing Practices (GMP), to ensure the safety and quality of the product.
3.4. Intellectual Property Protection and Regulatory Exclusivity
Intellectual property protection, in the form of patents, and regulatory exclusivity, which grants a period of market exclusivity to the original biologic developer, are crucial for incentivizing innovation in biologics development. Patents protect the invention of the biologic itself, as well as the manufacturing process and the formulation. Regulatory exclusivity provides a period of market exclusivity after the biologic is approved, during which biosimilars cannot be marketed. In the United States, biologics are eligible for 12 years of regulatory exclusivity under the Biologics Price Competition and Innovation Act (BPCIA). This exclusivity period is intended to provide biologic developers with a reasonable return on their investment, given the high costs and risks associated with biologics development. However, the length of the exclusivity period is a subject of debate, with some arguing that it is too long and others arguing that it is too short.
Many thanks to our sponsor Esdebe who helped us prepare this research report.
4. Biosimilars: Balancing Access and Innovation
Biosimilars are follow-on versions of innovator biologics that are approved by regulatory agencies after the innovator biologic’s patent and exclusivity periods have expired. Biosimilars are not identical to the innovator biologic, due to the inherent variability of biological manufacturing processes. However, biosimilars must demonstrate that they are highly similar to the innovator biologic in terms of safety, efficacy, and immunogenicity. The development of biosimilars is intended to improve patient access to biologics and reduce healthcare costs. However, the introduction of biosimilars also raises concerns about the potential impact on innovation.
4.1. Regulatory Approval of Biosimilars
The regulatory approval process for biosimilars is different from that for generic small-molecule drugs. Biosimilars must demonstrate that they are highly similar to the innovator biologic in terms of analytical characterization, nonclinical studies, and clinical studies. The clinical studies typically involve a comparative efficacy trial and a pharmacokinetic/pharmacodynamic (PK/PD) study. The regulatory agency may also require an immunogenicity study to assess the potential for the biosimilar to elicit an immune response. In the United States, the Food and Drug Administration (FDA) approves biosimilars under the BPCIA. The BPCIA also created a pathway for biosimilars to be designated as interchangeable with the innovator biologic, which means that they can be substituted for the innovator biologic by a pharmacist without the intervention of a physician. However, the FDA has only approved a few biosimilars as interchangeable to date.
4.2. Market Dynamics of Biosimilars
The market dynamics of biosimilars are complex and vary depending on the country and the therapeutic area. In some countries, biosimilars have achieved significant market penetration, while in others, their uptake has been slower. Factors that affect the market uptake of biosimilars include:
- Pricing: Biosimilars are typically priced at a discount to the innovator biologic, but the size of the discount can vary.
- Reimbursement policies: Reimbursement policies can favor the innovator biologic or the biosimilar.
- Physician and patient acceptance: Physicians and patients may be hesitant to switch from the innovator biologic to the biosimilar.
- Interchangeability: Biosimilars that are designated as interchangeable may have a greater market uptake.
4.3. Impact on Innovation
The introduction of biosimilars has the potential to reduce healthcare costs and improve patient access to biologics. However, it also raises concerns about the potential impact on innovation. Some argue that the lower prices of biosimilars may reduce the incentives for pharmaceutical companies to invest in the development of new biologics. Others argue that the competition from biosimilars may spur innovation by forcing pharmaceutical companies to develop more innovative biologics with improved efficacy or safety. The long-term impact of biosimilars on innovation is still uncertain and will depend on various factors, including the regulatory environment, the market dynamics, and the investment decisions of pharmaceutical companies.
Many thanks to our sponsor Esdebe who helped us prepare this research report.
5. Emerging Trends in Biologics Development
The field of biologics is rapidly evolving, with new technologies and approaches emerging that hold the promise of transforming healthcare. This section explores some of the emerging trends in biologics development.
5.1. Personalized Medicine
Personalized medicine, also known as precision medicine, involves tailoring medical treatment to the individual characteristics of each patient. Biologics are particularly well-suited for personalized medicine because they can be designed to target specific molecules or pathways that are relevant to a patient’s disease. For example, monoclonal antibodies can be designed to target specific cancer cells that express unique markers. Gene therapies can be used to correct or replace defective genes in patients with genetic diseases. As our understanding of the molecular basis of disease grows, personalized medicine is likely to play an increasingly important role in the development and use of biologics.
5.2. Gene Editing Technologies
Gene editing technologies, such as CRISPR-Cas9, offer the potential to precisely edit genes in living cells. Gene editing technologies can be used to correct or disable disease-causing genes, or to insert new genes into cells. These technologies have the potential to revolutionize the treatment of genetic diseases, cancers, and infectious diseases. However, gene editing technologies also raise ethical concerns, such as the potential for off-target effects and the use of gene editing for non-therapeutic purposes.
5.3. Artificial Intelligence (AI) and Machine Learning (ML)
Artificial intelligence (AI) and machine learning (ML) are increasingly being used in biologics discovery and development. AI and ML can be used to analyze large datasets of biological data to identify potential drug targets, predict the efficacy and safety of biologics, and optimize manufacturing processes. AI and ML can also be used to personalize medicine by predicting which patients are most likely to respond to a particular biologic. As the amount of biological data continues to grow, AI and ML are likely to play an increasingly important role in the development of biologics.
5.4. Combination Therapies
Combination therapies, which involve using two or more drugs together to treat a disease, are becoming increasingly common in the treatment of complex diseases, such as cancer. Biologics are often used in combination with other biologics or with small-molecule drugs to improve efficacy and reduce side effects. For example, monoclonal antibodies are often used in combination with chemotherapy to treat cancer. Combination therapies require careful planning and monitoring to ensure that the drugs are safe and effective when used together.
Many thanks to our sponsor Esdebe who helped us prepare this research report.
6. Conclusion
Biologics have revolutionized the treatment of many diseases, offering targeted therapies with improved efficacy and reduced side effects compared to traditional treatments. However, the development and manufacturing of biologics are significantly more complex and expensive than those of small-molecule drugs. This complexity translates into higher prices, which can limit patient access and strain healthcare budgets. Furthermore, the longer development timelines and higher failure rates associated with biologics pose significant financial risks for pharmaceutical companies. As a result, the regulatory framework for biologics must strike a delicate balance between promoting innovation and ensuring patient safety and affordability. Biosimilars offer the potential to improve patient access to biologics and reduce healthcare costs, but their introduction also raises concerns about the potential impact on innovation. Emerging trends in biologics development, such as personalized medicine, gene therapies, and the use of AI and ML, hold the promise of transforming healthcare in the coming decades. As the field of biologics continues to evolve, it is essential to foster a regulatory environment that promotes innovation while ensuring patient safety and affordability.
Many thanks to our sponsor Esdebe who helped us prepare this research report.
References
- U.S. Food and Drug Administration (FDA)
- European Medicines Agency (EMA)
- World Health Organization (WHO)
- National Institutes of Health (NIH)
- IQVIA Institute for Human Data Science
- EvaluatePharma
- Ledford, H. (2020). How COVID vaccines compare: Pfizer, Moderna and Oxford-AstraZeneca. Nature, 585(7824), 318-319.
- Walsh, G. (2018). Biopharmaceutical benchmarks 2018. Nature Biotechnology, 36(11), 1136-1145.
- Declerck, P. J. (2021). Biosimilars: current landscape and future trends. Journal of Internal Medicine, 289(2), 145-161.
- Leader, B., Baca, Q. J., & Golan, D. E. (2008). Protein therapeutics: a summary and pharmacological classification. Nature Reviews Drug Discovery, 7(1), 21-39.
- Carter, P. J., & Lazar, G. A. (2018). Next generation antibody therapeutics. Nature Reviews Drug Discovery, 17(3), 197-223.
Be the first to comment