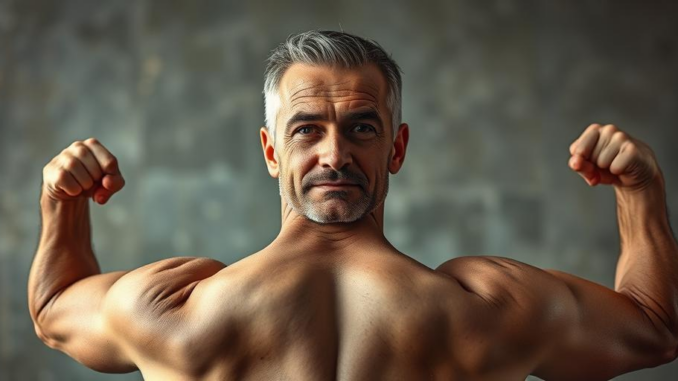
Abstract
Skeletal muscle is a crucial organ for locomotion, metabolic regulation, and overall health. Maintaining adequate muscle mass is paramount throughout the lifespan, particularly as age-related muscle loss (sarcopenia) and metabolic dysfunction become increasingly prevalent. This review synthesizes current research on the intricate relationship between muscle mass, aging, and metabolic health. We explore the mechanisms underlying age-related muscle decline, including hormonal changes, inflammation, and altered protein turnover. Furthermore, we examine the impact of muscle mass on metabolic parameters such as glucose homeostasis, insulin sensitivity, and lipid metabolism. Finally, we discuss various interventions, including exercise, nutrition, and pharmacological approaches, aimed at preserving and enhancing muscle mass in the context of aging and metabolic disorders. This review aims to provide a comprehensive overview for experts in the field, highlighting knowledge gaps and future research directions in this critical area.
Many thanks to our sponsor Esdebe who helped us prepare this research report.
1. Introduction
Skeletal muscle, constituting approximately 40% of total body mass, is far more than just a tissue responsible for movement. It is now recognized as a crucial endocrine organ, secreting myokines that influence a wide range of physiological processes, including glucose metabolism, inflammation, and even brain function (Pedersen & Febbraio, 2012). Maintaining adequate muscle mass is essential for overall health and longevity, impacting physical function, metabolic health, and quality of life. Conversely, a decline in muscle mass, particularly with aging, is associated with increased risk of falls, disability, metabolic disorders like type 2 diabetes, and even mortality (Newman et al., 2003).
The global population is aging rapidly, leading to an increased prevalence of age-related conditions, including sarcopenia and dynapenia (muscle weakness). Sarcopenia, characterized by a loss of muscle mass and function, is a significant public health concern due to its profound impact on independence and healthcare costs (Cruz-Jentoft et al., 2019). Furthermore, the rising prevalence of obesity and metabolic syndrome contributes to muscle dysfunction and accelerated muscle loss.
This review will delve into the complex interplay of muscle mass, aging, and metabolic health. We will explore the underlying mechanisms of age-related muscle decline, focusing on the molecular and physiological changes that contribute to sarcopenia. Furthermore, we will examine the critical role of muscle mass in metabolic regulation, discussing its impact on glucose homeostasis, insulin sensitivity, and lipid metabolism. Finally, we will critically evaluate various interventions designed to preserve and enhance muscle mass, including exercise, nutrition, and pharmacological approaches, particularly in the context of aging and metabolic disorders.
Many thanks to our sponsor Esdebe who helped us prepare this research report.
2. Mechanisms of Age-Related Muscle Decline (Sarcopenia)
Sarcopenia is a multifactorial syndrome characterized by the progressive and generalized loss of skeletal muscle mass and strength with a risk of adverse outcomes such as physical disability, poor quality of life and death (Cruz-Jentoft et al., 2019). The underlying mechanisms of sarcopenia are complex and involve a combination of factors, including:
2.1. Hormonal Changes
The endocrine system plays a critical role in regulating muscle protein synthesis and breakdown. Several hormones, including testosterone, growth hormone (GH), and insulin-like growth factor-1 (IGF-1), decline with age, contributing to muscle loss. Testosterone, a potent anabolic hormone, stimulates muscle protein synthesis and inhibits muscle protein breakdown (Bhasin et al., 1996). Age-related decline in testosterone levels leads to reduced muscle mass and strength. GH and IGF-1 are also crucial for muscle growth and repair. GH stimulates the liver to produce IGF-1, which promotes muscle protein synthesis and satellite cell activation (Florini et al., 1996). The age-related decline in GH secretion and IGF-1 levels contributes to reduced muscle regeneration and repair capacity. Furthermore, the decline in these hormones can alter substrate utilization leading to reduced lean mass.
2.2. Inflammation
Chronic low-grade inflammation, often referred to as “inflammaging,” is a hallmark of aging and contributes to muscle wasting. Elevated levels of pro-inflammatory cytokines, such as tumor necrosis factor-alpha (TNF-α), interleukin-6 (IL-6), and interleukin-1β (IL-1β), can impair muscle protein synthesis and promote muscle protein breakdown (Goodman, 2019). These cytokines activate catabolic pathways, such as the ubiquitin-proteasome system and autophagy, leading to the degradation of muscle proteins. Furthermore, chronic inflammation can impair satellite cell function, reducing the muscle’s ability to repair and regenerate after injury. Macrophages also play a role in inflammation and muscle health, with M1 macrophages promoting inflammation and M2 macrophages promoting tissue repair.
2.3. Altered Protein Turnover
Muscle mass is determined by the balance between muscle protein synthesis (MPS) and muscle protein breakdown (MPB). With aging, there is a decline in MPS and/or an increase in MPB, leading to a net loss of muscle protein. Reduced MPS responsiveness to anabolic stimuli, such as amino acids and resistance exercise, is a key feature of sarcopenia (Volpi et al., 2001). This phenomenon, known as “anabolic resistance,” makes it more difficult for older adults to build and maintain muscle mass. Furthermore, increased MPB, driven by factors like inflammation and oxidative stress, contributes to the overall loss of muscle protein. Autophagy, a cellular process that removes damaged proteins and organelles, can also contribute to muscle wasting if it becomes dysregulated.
2.4. Mitochondrial Dysfunction
Mitochondria are the powerhouses of the cell, responsible for producing energy (ATP) through oxidative phosphorylation. With aging, mitochondrial function declines, leading to reduced ATP production, increased oxidative stress, and accumulation of damaged mitochondria (Picard et al., 2013). Mitochondrial dysfunction impairs muscle function and contributes to muscle wasting. Furthermore, damaged mitochondria can trigger inflammation, further exacerbating muscle loss. Mitophagy, the selective removal of damaged mitochondria, is also impaired with aging, leading to the accumulation of dysfunctional mitochondria.
2.5. Neuromuscular Junction Dysfunction
The neuromuscular junction (NMJ) is the synapse between a motor neuron and a muscle fiber. The NMJ transmits signals from the nervous system to the muscle, enabling muscle contraction. With aging, the NMJ undergoes structural and functional changes, leading to reduced signaling efficiency and impaired muscle activation (Deschenes et al., 2010). These changes contribute to muscle weakness and reduced motor control. Denervation, the loss of nerve connections to muscle fibers, can also occur with aging, leading to muscle atrophy.
2.6. Reduced Satellite Cell Function
Satellite cells are muscle stem cells that play a critical role in muscle repair and regeneration. They are located between the muscle fiber and the basal lamina and are activated in response to muscle damage. Upon activation, satellite cells proliferate, differentiate into myoblasts, and fuse with existing muscle fibers to repair the damage or form new muscle fibers. With aging, satellite cell number and function decline, reducing the muscle’s ability to repair and regenerate after injury (Sousa-Victor et al., 2014). This impaired regenerative capacity contributes to muscle wasting.
Many thanks to our sponsor Esdebe who helped us prepare this research report.
3. The Role of Muscle Mass in Metabolic Regulation
Skeletal muscle plays a crucial role in regulating metabolic homeostasis, particularly glucose metabolism, insulin sensitivity, and lipid metabolism. Reduced muscle mass is strongly associated with metabolic dysfunction and increased risk of metabolic disorders.
3.1. Glucose Metabolism and Insulin Sensitivity
Muscle is the primary site of glucose disposal in the body, accounting for approximately 80% of insulin-stimulated glucose uptake (DeFronzo et al., 1981). Muscle mass is positively correlated with insulin sensitivity, meaning that individuals with more muscle mass are more responsive to insulin. Reduced muscle mass leads to decreased glucose uptake and insulin resistance, increasing the risk of type 2 diabetes. Muscle also stores glucose in the form of glycogen, providing a readily available energy source for muscle contraction. Impaired glycogen storage in muscle contributes to hyperglycemia and insulin resistance. Myokines released from muscle also play a role in regulating glucose metabolism. For example, irisin, a myokine released during exercise, has been shown to improve glucose tolerance and insulin sensitivity (Boström et al., 2012).
3.2. Lipid Metabolism
Muscle plays a critical role in fatty acid oxidation. It is a major site of fatty acid uptake and oxidation, contributing to overall energy expenditure. Reduced muscle mass leads to decreased fatty acid oxidation and increased lipid accumulation in other tissues, such as the liver and adipose tissue, contributing to dyslipidemia and non-alcoholic fatty liver disease (NAFLD). Muscle also produces myokines that influence lipid metabolism. For example, brain-derived neurotrophic factor (BDNF), a myokine released during exercise, has been shown to improve lipid profiles and reduce body fat (Wrann et al., 2013).
3.3. Energy Expenditure
Muscle is a metabolically active tissue that contributes significantly to resting metabolic rate (RMR). RMR is the amount of energy the body expends at rest to maintain vital functions. Reduced muscle mass leads to a decrease in RMR, making it more difficult to maintain a healthy weight. This reduction in RMR can contribute to weight gain and obesity. Furthermore, muscle is responsible for a significant portion of postprandial thermogenesis, the increase in energy expenditure that occurs after eating. Reduced muscle mass can impair postprandial thermogenesis, contributing to weight gain.
3.4. Systemic Inflammation
As previously discussed, the systemic inflammatory state contributes to muscle loss, but it also works in the other direction. Reduced muscle mass, particularly when coupled with increased adiposity, can exacerbate systemic inflammation. Adipose tissue, particularly visceral fat, releases pro-inflammatory cytokines that can contribute to insulin resistance and metabolic dysfunction. Muscle, on the other hand, can release anti-inflammatory myokines that counteract the effects of pro-inflammatory cytokines. The balance between pro-inflammatory and anti-inflammatory factors is disrupted in individuals with reduced muscle mass and increased adiposity, leading to a chronic inflammatory state.
Many thanks to our sponsor Esdebe who helped us prepare this research report.
4. Interventions to Preserve and Enhance Muscle Mass
Given the critical role of muscle mass in health and longevity, it is essential to implement strategies to preserve and enhance muscle mass throughout the lifespan. The following interventions have shown promise in combating age-related muscle decline and improving metabolic health:
4.1. Exercise
Exercise, particularly resistance exercise, is a potent stimulus for muscle protein synthesis and muscle hypertrophy (increase in muscle size). Resistance exercise stimulates muscle protein synthesis by activating signaling pathways, such as the mTOR pathway, which promotes the translation of mRNA into muscle proteins (Baar, 2014). Resistance exercise also stimulates satellite cell activation, promoting muscle repair and regeneration. The optimal frequency, intensity, and volume of resistance exercise for muscle hypertrophy vary depending on individual factors such as age, training status, and genetics. However, generally, performing resistance exercise 2-3 times per week, targeting all major muscle groups, with moderate to high intensity (60-85% of 1 repetition maximum) is recommended.
Endurance exercise can also contribute to muscle health, although its effects on muscle hypertrophy are less pronounced than resistance exercise. Endurance exercise improves mitochondrial function and increases muscle capillarization, enhancing oxygen delivery to the muscle. Furthermore, endurance exercise can stimulate the release of myokines that have beneficial effects on metabolic health.
Combining resistance and endurance exercise, known as concurrent training, can provide the benefits of both types of exercise. However, the optimal sequencing and timing of resistance and endurance exercise may influence the adaptation to each type of training.
4.2. Nutrition
Adequate protein intake is crucial for muscle protein synthesis and muscle maintenance. The recommended dietary allowance (RDA) for protein is 0.8 grams per kilogram of body weight per day. However, older adults may require higher protein intakes to counteract anabolic resistance and maintain muscle mass. Studies have shown that protein intakes of 1.2-1.5 grams per kilogram of body weight per day may be beneficial for older adults (Deutz et al., 2014). The timing and distribution of protein intake throughout the day are also important. Consuming protein-rich meals or snacks throughout the day, rather than concentrating protein intake in a single meal, may be more effective at stimulating muscle protein synthesis. The type of protein consumed is also a factor. High-quality protein sources, such as animal-based proteins (e.g., meat, poultry, fish, eggs, dairy), contain all essential amino acids in sufficient amounts to support muscle protein synthesis. Whey protein, a dairy protein, is particularly effective at stimulating muscle protein synthesis due to its high leucine content.
In addition to protein, other nutrients play a role in muscle health. Creatine supplementation has been shown to increase muscle mass and strength, particularly when combined with resistance exercise. Vitamin D is important for muscle function, and vitamin D deficiency is associated with muscle weakness and increased risk of falls. Omega-3 fatty acids have anti-inflammatory properties and may help to preserve muscle mass. Adequate calorie intake is also essential for muscle maintenance. Calorie restriction can lead to muscle loss, particularly in older adults.
4.3. Pharmacological Interventions
While exercise and nutrition are the cornerstones of muscle health, pharmacological interventions may be considered in certain cases, particularly for individuals with severe sarcopenia or other medical conditions. Testosterone replacement therapy can increase muscle mass and strength in men with low testosterone levels. However, testosterone therapy is associated with potential side effects, such as prostate enlargement and cardiovascular risks. Growth hormone (GH) therapy can also increase muscle mass, but it is also associated with potential side effects, such as joint pain and insulin resistance. Selective androgen receptor modulators (SARMs) are a class of drugs that selectively bind to androgen receptors in muscle and bone, with fewer side effects than testosterone. However, SARMs are not yet approved for widespread use and are considered performance-enhancing drugs by many sports organizations. Myostatin inhibitors are another class of drugs that are being investigated for their potential to increase muscle mass. Myostatin is a protein that inhibits muscle growth. Inhibiting myostatin can lead to increased muscle mass and strength. However, the long-term effects of myostatin inhibitors are not yet known. Other potential pharmacological interventions include ACE inhibitors, Vitamin D and Beta-hydroxy-beta-methylbutyrate (HMB).
4.4. Emerging Therapies
Several emerging therapies are being investigated for their potential to improve muscle health. Gene therapy, which involves delivering genes that promote muscle growth or inhibit muscle breakdown, is a promising area of research. Mitochondrial therapies, which aim to improve mitochondrial function, are also being explored. These therapies include mitochondrial transplantation and the use of mitochondrial-targeted antioxidants. Senolytics, drugs that selectively kill senescent cells (aged and dysfunctional cells), are also being investigated for their potential to improve muscle health by reducing inflammation and promoting tissue regeneration. Cell-based therapies, such as satellite cell transplantation, are also being explored as a potential treatment for muscle wasting.
Many thanks to our sponsor Esdebe who helped us prepare this research report.
5. Future Research Directions
Despite significant advances in our understanding of muscle mass, aging, and metabolic health, many questions remain unanswered. Future research should focus on the following areas:
- Personalized interventions: Developing personalized exercise and nutrition programs based on individual genetic profiles, metabolic characteristics, and training status. This will require the development of biomarkers that can predict an individual’s response to different interventions.
- Longitudinal studies: Conducting long-term studies to assess the effects of different interventions on muscle mass, function, and metabolic health over the lifespan. These studies should also examine the impact of these interventions on chronic disease risk and mortality.
- Mechanistic studies: Further elucidating the molecular mechanisms underlying age-related muscle decline and the beneficial effects of interventions. This will require the use of advanced techniques, such as proteomics, metabolomics, and transcriptomics.
- Clinical trials: Conducting large-scale clinical trials to evaluate the efficacy and safety of novel pharmacological and emerging therapies for muscle wasting. These trials should include diverse populations and assess clinically relevant outcomes, such as physical function and quality of life.
- Developing new biomarkers: Identifying new biomarkers of muscle health that can be used to diagnose sarcopenia early and monitor the effectiveness of interventions. These biomarkers should be non-invasive and readily accessible.
- Impact of GLP-1 therapies on long-term muscle health: Further research is required into the long-term impact of GLP-1 therapies on muscle health. While research has shown that GLP-1 therapies can lead to loss of lean muscle mass, the long-term health implications need to be investigated.
Many thanks to our sponsor Esdebe who helped us prepare this research report.
6. Conclusion
Maintaining adequate muscle mass is crucial for overall health, particularly as we age. Age-related muscle decline (sarcopenia) is a complex process involving hormonal changes, inflammation, altered protein turnover, mitochondrial dysfunction, and neuromuscular junction dysfunction. Reduced muscle mass is strongly associated with metabolic dysfunction, including insulin resistance, dyslipidemia, and decreased energy expenditure. Exercise, particularly resistance exercise, and adequate protein intake are essential for preserving and enhancing muscle mass. Pharmacological interventions may be considered in certain cases, but they are associated with potential side effects. Future research should focus on personalized interventions, longitudinal studies, mechanistic studies, and clinical trials to further advance our understanding of muscle mass, aging, and metabolic health. The development of new biomarkers of muscle health is also crucial for early diagnosis and monitoring of interventions. Furthermore, more research into the long term implications of GLP-1 therapies on muscle health is needed so doctors can best advise their patients.
Many thanks to our sponsor Esdebe who helped us prepare this research report.
References
- Baar, K. (2014). Training for muscle growth: interfering stimuli. European Journal of Applied Physiology, 114(6), 1249-1260.
- Bhasin, S., Storer, T. W., Berman, N., Callegari, C., Clevenger, B., Phillips, J., … & Swerdloff, R. S. (1996). The effects of supraphysiologic doses of testosterone on muscle size and strength in normal men. New England Journal of Medicine, 335(1), 1-7.
- Boström, P., Wu, J., Jedrychowski, M. P., Korde, A., Ye, L., Lo, J. C., … & Spiegelman, B. M. (2012). A PGC1-α-dependent myokine that drives brown-fat differentiation and glucose homeostasis. Nature, 482(7386), 450-452.
- Cruz-Jentoft, A. J., Bahat, G., Bauer, J., Boirie, Y., Bruyère, O., Cederholm, T., … & ESSOP. (2019). Sarcopenia: revised European consensus on definition and diagnosis. Age and Ageing, 48(1), 16-31.
- DeFronzo, R. A., Jacot, E., Jequier, E., Maeder, E., Wahren, J., & Felber, J. P. (1981). Insulin sensitivity and glucose metabolism for both skeletal muscle and whole body in man. The Journal of Clinical Investigation, 68(6), 1468-1474.
- Deschenes, M. R., Roby, F. B., & Ruiz, R. J. (2010). Neurotrophic factors and neuromuscular aging. Exercise and Sport Sciences Reviews, 38(2), 76-82.
- Deutz, N. E., Bauer, J. M., Barazzoni, R., Biolo, G., Boirie, Y., Bosy-Westphal, A., … & Calder, P. C. (2014). Protein intake and exercise for optimal muscle function with aging: recommendations from the ESPEN Expert Group. Clinical Nutrition, 33(6), 929-936.
- Florini, J. R., Ewton, D. Z., & Coolican, S. A. (1996). Growth hormone and insulin-like growth factor-I in muscle cell growth. Journal of Animal Science, 74(10), 2381-2392.
- Goodman, M. N. (2019). Interleukin-6 in aging and disease. Aging and Disease, 10(1), 135.
- Newman, A. B., Kupelian, V., Visser, M., Simonsick, E. M., Goodpaster, B. H., Kritchevsky, S. B., … & Harris, T. B. (2003). Strength, but not muscle mass, is associated with mortality in the health, aging and body composition study. The Journals of Gerontology Series A: Biological Sciences and Medical Sciences, 58(1), 72-77.
- Pedersen, B. K., & Febbraio, M. A. (2012). Muscles as endocrine organs: focus on muscle-derived interleukin-6. Physiological Reviews, 88(4), 1219-1247.
- Picard, M., Turnbull, D. M., & Garesse, R. (2013). Nuclear control of mitochondrial quantity and quality in mammalian cells. American Journal of Physiology-Cell Physiology, 304(2), C147-C160.
- Sousa-Victor, P., García-Prat, L., Muñoz-Cánoves, P. (2014). Mechanisms controlling stem cell behaviour in muscle regeneration. Semin Cell Dev Biol. 30: 58-66.
- Volpi, E., Kobayashi, H., Sheffield-Moore, M., Mittendorfer, B., & Wolfe, R. R. (2001). Essential amino acids are primarily responsible for the amino acid stimulation of muscle protein anabolism in healthy elderly adults. American Journal of Clinical Nutrition, 78(2), 250-258.
- Wrann, C. D., Tabakman, A., Shoer, A., Garcia-Caceres, C., Goekjian, J. R., Goldberg, E. L., … & Spiegelman, B. M. (2013). Exercise induces hippocampal BDNF and protects against cognitive impairment. Cell Metabolism, 18(5), 649-657.
So, muscle is an endocrine organ now? I always knew skipping leg day was messing with more than just my physique. Guess I should start invoicing my biceps for their contribution to my brain function. Any suggestions for hourly rates?
That’s a great point! Viewing muscle as an endocrine organ highlights its far-reaching effects. And the idea of invoicing those biceps is hilarious. Maybe base the rates on improved cognitive function or reduced risk of metabolic diseases? It’s a novel business model to consider for sure!
Editor: MedTechNews.Uk
Thank you to our Sponsor Esdebe
So, muscles have their own PR team now, releasing myokines like celebrity endorsements! Is there a talent agency for organs? Asking for my increasingly neglected liver.
That’s a hilarious analogy! The ‘talent agency for organs’ – I love it! It really emphasizes the importance of looking after our internal ecosystems. Perhaps that’s what doctors are, in a way, the organ talent agents making sure they are looked after and healthy! Thanks for the fun perspective!
Editor: MedTechNews.Uk
Thank you to our Sponsor Esdebe
Given sarcopenia’s complex interplay of hormonal, inflammatory, and protein turnover factors, how do these mechanisms differentially impact various muscle fiber types, and what are the implications for targeted interventions?