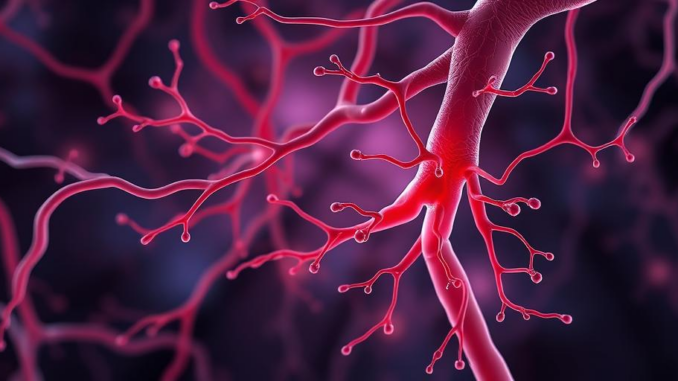
Abstract
The microvasculature, a complex network of capillaries, arterioles, and venules, serves as the critical interface between the circulating blood and the tissues, dictating nutrient delivery, waste removal, and immune cell trafficking. Its intricate structure and dynamic function are essential for maintaining tissue homeostasis and orchestrating appropriate responses to injury and inflammation. This research report delves into the multifaceted role of microvessels, exploring their intricate structure, diverse functions, and susceptibility to dysfunction in a variety of disease states. We examine the mechanisms of microvascular damage, including the roles of endothelial cell activation, platelet aggregation, leukocyte adhesion, and the coagulation cascade, with a particular focus on thromboinflammation. Furthermore, we discuss the challenges in assessing and treating microvascular dysfunction, highlighting the limitations of current imaging techniques and therapeutic strategies. Finally, we explore emerging therapeutic avenues targeting endothelial cell protection, inflammation resolution, and microvascular regeneration, paving the way for novel interventions to restore microvascular integrity and improve patient outcomes.
Many thanks to our sponsor Esdebe who helped us prepare this research report.
1. Introduction
The microvasculature, constituting the terminal branches of the circulatory system, is more than just a conduit for blood flow; it represents a dynamic and highly regulated microenvironment crucial for tissue viability and function. This intricate network of microvessels, composed primarily of endothelial cells (ECs) and a supporting basement membrane, permeates virtually every tissue in the body, ensuring efficient delivery of oxygen and nutrients while simultaneously removing metabolic waste products. Beyond this fundamental role, the microvasculature plays a pivotal part in regulating inflammation, immune cell trafficking, and angiogenesis, dynamically adapting to changing physiological and pathological conditions. Dysregulation of microvascular function, often manifested as endothelial dysfunction, microvascular rarefaction, or microvascular occlusion, contributes significantly to the pathogenesis of a wide range of diseases, including cardiovascular disease, diabetes, neurodegenerative disorders, and cancer. Understanding the complex interplay between microvascular structure, function, and its response to various stimuli is paramount for developing effective therapeutic strategies to prevent and treat microvascular-related diseases.
Many thanks to our sponsor Esdebe who helped us prepare this research report.
2. Structure and Function of the Microvasculature
The microvasculature is a heterogeneous network of vessels, varying in structure and function depending on the tissue and organ in which they reside. The key components of the microvasculature include:
- Arterioles: These small arteries, characterized by a relatively thick smooth muscle layer, play a critical role in regulating blood flow and blood pressure. Their ability to constrict and dilate allows for precise control of microvascular perfusion in response to local metabolic demands and systemic signals.
- Capillaries: The capillaries, the smallest blood vessels in the body, are the primary site of gas exchange, nutrient delivery, and waste removal. Their thin walls, consisting of a single layer of ECs and a basement membrane, facilitate efficient diffusion of substances between the blood and the surrounding tissues. Capillary structure and permeability vary significantly depending on the tissue type. For example, continuous capillaries in the brain have tight junctions between ECs, forming the blood-brain barrier, while fenestrated capillaries in the kidneys and intestines have pores that allow for greater permeability.
- Venules: These small veins collect blood from the capillaries and return it to the larger veins. Postcapillary venules are also important sites for leukocyte adhesion and extravasation during inflammation.
2.1 Endothelial Cells: The Master Regulators of Microvascular Function
ECs, forming the inner lining of all blood vessels, are far more than just a passive barrier. They are highly active and dynamic cells that play a crucial role in regulating microvascular tone, permeability, coagulation, inflammation, and angiogenesis. ECs respond to a variety of stimuli, including shear stress, growth factors, cytokines, and hormones, by releasing a range of vasoactive substances, such as nitric oxide (NO), prostacyclin (PGI2), and endothelin-1 (ET-1), which regulate vascular tone and blood flow. Endothelial cell glycocalyx, a layer composed of proteoglycans and glycoproteins on the luminal side of endothelial cells, is crucial for endothelial cell function and vascular integrity. It regulates vascular permeability, protects the endothelium from damage, and mediates the interactions between blood cells and the endothelium [1]. In addition, ECs express a variety of adhesion molecules, such as selectins, integrins, and immunoglobulin superfamily members, which mediate the adhesion and extravasation of leukocytes during inflammation. ECs also secrete growth factors, such as vascular endothelial growth factor (VEGF), which stimulate angiogenesis, the formation of new blood vessels. Endothelial dysfunction, characterized by impaired NO production, increased expression of adhesion molecules, and increased permeability, is a hallmark of many diseases, contributing to vasoconstriction, inflammation, and thrombosis.
2.2 Microvascular Blood Flow and Permeability
Microvascular blood flow is tightly regulated to match the metabolic demands of the surrounding tissues. Arteriolar tone, capillary density, and blood viscosity all influence microvascular perfusion. Autoregulation mechanisms maintain constant blood flow despite changes in systemic blood pressure. Pathological conditions, such as inflammation, ischemia, and hyperglycemia, can disrupt microvascular blood flow, leading to tissue hypoxia and damage. Microvascular permeability, the ability of substances to pass across the microvascular wall, is also tightly regulated. Endothelial cell junctions, the glycocalyx, and the surrounding basement membrane all contribute to the barrier function of the microvasculature. Increased microvascular permeability, often resulting from endothelial dysfunction, leads to edema and inflammation.
Many thanks to our sponsor Esdebe who helped us prepare this research report.
3. Mechanisms of Microvascular Damage in Disease
Microvascular damage is a prominent feature of numerous diseases, contributing significantly to their pathogenesis and progression. The underlying mechanisms of microvascular damage are complex and multifactorial, involving a combination of endothelial dysfunction, inflammation, thrombosis, and structural alterations. This section will examine the key mechanisms of microvascular damage in different disease states.
3.1 Endothelial Dysfunction: A Central Mediator of Microvascular Damage
As mentioned previously, endothelial dysfunction is a key driver of microvascular damage. It results from an imbalance between vasodilating and vasoconstricting factors, pro-inflammatory and anti-inflammatory mediators, and pro-coagulant and anti-coagulant molecules. Risk factors for endothelial dysfunction include hypertension, hyperlipidemia, diabetes, smoking, and inflammation. Endothelial dysfunction leads to impaired NO production, increased oxidative stress, increased expression of adhesion molecules, and increased permeability, all of which contribute to microvascular damage.
3.2 Inflammation and Thromboinflammation
Inflammation plays a significant role in microvascular damage in many diseases. Pro-inflammatory cytokines, such as TNF-α, IL-1β, and IL-6, activate ECs, leading to increased expression of adhesion molecules and increased permeability. Leukocyte adhesion and extravasation further exacerbate inflammation and cause microvascular damage through the release of reactive oxygen species (ROS) and proteases. Thromboinflammation, characterized by the interplay between inflammation and coagulation, is increasingly recognized as a critical mechanism of microvascular damage in various diseases [2]. Activation of the coagulation cascade leads to the formation of microthrombi, which obstruct microvascular blood flow and cause tissue ischemia. Platelets play a crucial role in thromboinflammation, adhering to activated ECs and releasing pro-inflammatory mediators. The interaction between platelets, leukocytes, and ECs amplifies the inflammatory response and promotes microvascular thrombosis.
3.3 Microvascular Occlusion: A Critical Consequence of Microvascular Damage
Microvascular occlusion, the blockage of small blood vessels, is a frequent consequence of microvascular damage. It can result from thrombus formation, leukocyte plugging, endothelial cell swelling, or structural alterations of the microvessels. Microvascular occlusion leads to tissue ischemia and hypoxia, which can cause irreversible tissue damage. Several factors contribute to microvascular occlusion, including increased blood viscosity, impaired red blood cell deformability, and reduced microvascular perfusion pressure.
3.4 Specific Examples of Microvascular Damage in Disease
- Diabetes: Hyperglycemia and insulin resistance induce endothelial dysfunction, leading to microvascular complications such as retinopathy, nephropathy, and neuropathy. Advanced glycation end products (AGEs) accumulate in the microvasculature, further contributing to endothelial damage and inflammation.
- Cardiovascular Disease: Atherosclerosis, hypertension, and heart failure are associated with microvascular dysfunction and rarefaction in the heart and peripheral tissues. Microvascular dysfunction contributes to myocardial ischemia, impaired angiogenesis, and reduced tissue perfusion.
- Neurodegenerative Disorders: Microvascular dysfunction and reduced cerebral blood flow are implicated in the pathogenesis of Alzheimer’s disease, Parkinson’s disease, and stroke. Amyloid-β deposition in the microvasculature can cause endothelial damage and impair cerebral blood flow.
- Sepsis: Sepsis-induced systemic inflammation leads to endothelial dysfunction, increased permeability, and microvascular thrombosis, resulting in tissue hypoxia and organ dysfunction.
- COVID-19: The SARS-CoV-2 virus can directly infect ECs, leading to endothelial dysfunction, inflammation, and microvascular thrombosis, contributing to the pathogenesis of severe COVID-19 [3].
Many thanks to our sponsor Esdebe who helped us prepare this research report.
4. Assessing Microvascular Health: Challenges and Advances
The accurate assessment of microvascular health is crucial for diagnosing and monitoring microvascular diseases, evaluating the efficacy of therapeutic interventions, and predicting patient outcomes. However, the microvasculature presents significant challenges for imaging and assessment due to its small size and complex network structure. Traditional methods such as angiography often lack the resolution to visualize the microvasculature effectively. This section will discuss the current challenges and advances in assessing microvascular health.
4.1 Current Imaging Techniques
- Laser Doppler Flowmetry (LDF): LDF measures microvascular blood flow by detecting changes in the frequency of laser light scattered by moving red blood cells. While LDF is non-invasive and relatively inexpensive, it provides only a global measure of microvascular perfusion and lacks spatial resolution.
- Orthogonal Polarization Spectral (OPS) Imaging: OPS imaging uses polarized light to visualize microvascular blood flow and vessel density in superficial tissues. However, OPS imaging is limited by its shallow penetration depth and susceptibility to motion artifacts.
- Sidestream Dark-Field (SDF) Imaging: SDF imaging, a modification of OPS imaging, uses a light source that is blocked from entering the objective lens, creating a dark-field effect that enhances the visualization of microvessels. SDF imaging is widely used to assess microvascular perfusion in critically ill patients.
- Optical Coherence Tomography (OCT): OCT uses near-infrared light to create high-resolution cross-sectional images of tissues. OCT angiography (OCTA) is a non-invasive technique that can visualize microvascular networks in the retina and skin.
- Contrast-Enhanced Ultrasound (CEUS): CEUS uses microbubbles as contrast agents to enhance the visualization of microvessels. CEUS can be used to assess microvascular perfusion in various organs, including the heart, liver, and kidneys.
- Magnetic Resonance Imaging (MRI): MRI can provide detailed anatomical and functional information about the microvasculature. Dynamic contrast-enhanced MRI (DCE-MRI) is used to assess microvascular permeability and perfusion. Arterial spin labeling (ASL) MRI is a non-invasive technique that can measure cerebral blood flow. While MRI offers good spatial resolution and tissue penetration, it is expensive and time-consuming.
- Two-Photon Microscopy: Two-photon microscopy is a powerful technique for imaging the microvasculature in vivo. It uses near-infrared light to excite fluorescent molecules, allowing for deep tissue penetration and high-resolution imaging. However, two-photon microscopy is limited by its invasiveness and is primarily used in animal studies.
4.2 Limitations and Challenges
While these imaging techniques have advanced our understanding of microvascular function, they still face several limitations and challenges:
- Limited spatial resolution: Many of the current imaging techniques lack the resolution to visualize the smallest microvessels and individual ECs.
- Shallow penetration depth: Some techniques, such as OPS and SDF imaging, are limited by their shallow penetration depth, making it difficult to assess microvascular function in deeper tissues.
- Susceptibility to artifacts: Motion artifacts, respiratory artifacts, and variations in skin pigmentation can affect the accuracy of microvascular imaging.
- Lack of standardization: There is a lack of standardization in image acquisition and analysis, making it difficult to compare results across different studies.
- Invasiveness: Some imaging techniques, such as two-photon microscopy, are invasive and cannot be used in humans.
4.3 Emerging Technologies
Several emerging technologies hold promise for improving the assessment of microvascular health:
- Super-resolution microscopy: Super-resolution microscopy techniques, such as stimulated emission depletion (STED) microscopy and structured illumination microscopy (SIM), can overcome the diffraction limit of light, allowing for visualization of subcellular structures and individual molecules in the microvasculature.
- Photoacoustic imaging: Photoacoustic imaging combines the high spatial resolution of ultrasound with the high contrast of optical imaging. It can be used to visualize microvascular networks and measure oxygen saturation.
- Microfluidic devices: Microfluidic devices can be used to mimic the microvascular environment in vitro, allowing for the study of EC function and drug responses.
- Artificial intelligence (AI): AI algorithms can be used to analyze microvascular images and extract quantitative parameters, such as vessel density, diameter, and tortuosity. AI can also be used to detect subtle changes in microvascular structure and function that may be missed by human observers.
Many thanks to our sponsor Esdebe who helped us prepare this research report.
5. Therapeutic Strategies Targeting Microvascular Dysfunction
Given the critical role of microvascular dysfunction in various diseases, therapeutic strategies aimed at restoring microvascular integrity and function hold great promise. These strategies can target different aspects of microvascular dysfunction, including endothelial cell protection, inflammation resolution, microvascular regeneration, and antithrombotic therapies. This section will discuss emerging therapeutic avenues targeting microvascular dysfunction.
5.1 Endothelial Cell Protection
Protecting ECs from damage is a key strategy for preventing and treating microvascular dysfunction. Several approaches can be used to protect ECs:
- Antioxidants: Oxidative stress plays a major role in endothelial dysfunction. Antioxidants, such as vitamin C, vitamin E, and N-acetylcysteine (NAC), can reduce oxidative stress and protect ECs from damage.
- Statins: Statins, commonly used to lower cholesterol, have also been shown to improve endothelial function by increasing NO production and reducing inflammation.
- ACE inhibitors and ARBs: Angiotensin-converting enzyme (ACE) inhibitors and angiotensin II receptor blockers (ARBs) can protect ECs by reducing oxidative stress and inflammation.
- Endothelial progenitor cells (EPCs): EPCs are bone marrow-derived cells that can differentiate into ECs and promote endothelial repair. EPC therapy has shown promise in preclinical studies for improving microvascular function in various diseases. However, clinical trials have yielded mixed results.
5.2 Inflammation Resolution
Resolving inflammation is crucial for preventing further microvascular damage. Several strategies can be used to resolve inflammation:
- Anti-inflammatory drugs: Nonsteroidal anti-inflammatory drugs (NSAIDs) and corticosteroids can reduce inflammation and protect the microvasculature. However, long-term use of these drugs can have significant side effects.
- Omega-3 fatty acids: Omega-3 fatty acids, such as EPA and DHA, have anti-inflammatory properties and can improve endothelial function.
- Resolvins and lipoxins: Resolvins and lipoxins are lipid mediators that promote inflammation resolution. They can reduce leukocyte adhesion and promote macrophage phagocytosis of apoptotic cells.
- Targeting inflammatory cytokines: Antibodies or inhibitors that target inflammatory cytokines, such as TNF-α, IL-1β, and IL-6, can reduce inflammation and protect the microvasculature. These therapies have shown promise in treating inflammatory diseases such as rheumatoid arthritis and inflammatory bowel disease.
5.3 Microvascular Regeneration
Promoting microvascular regeneration, the formation of new microvessels, can restore tissue perfusion and improve tissue function. Several approaches can be used to promote microvascular regeneration:
- Vascular endothelial growth factor (VEGF): VEGF is a potent angiogenic factor that stimulates EC proliferation and migration. VEGF therapy has shown promise in preclinical studies for promoting microvascular regeneration in ischemic tissues. However, clinical trials have yielded mixed results, potentially due to the complex effects of VEGF on vascular permeability and stability.
- Stem cell therapy: Stem cells, such as mesenchymal stem cells (MSCs) and induced pluripotent stem cells (iPSCs), can differentiate into ECs and promote angiogenesis. Stem cell therapy has shown promise in preclinical studies for promoting microvascular regeneration in ischemic tissues. Clinical trials are ongoing to evaluate the safety and efficacy of stem cell therapy for microvascular diseases.
- Gene therapy: Gene therapy can be used to deliver genes encoding angiogenic factors, such as VEGF, to promote microvascular regeneration.
5.4 Antithrombotic Therapies
Preventing microvascular thrombosis is crucial for maintaining microvascular perfusion. Several antithrombotic therapies are available:
- Antiplatelet drugs: Antiplatelet drugs, such as aspirin and clopidogrel, inhibit platelet aggregation and reduce the risk of thrombus formation.
- Anticoagulants: Anticoagulants, such as heparin and warfarin, inhibit the coagulation cascade and reduce the risk of thrombus formation. Direct oral anticoagulants (DOACs) are newer anticoagulants that are more convenient to use than warfarin.
- Thrombolytic drugs: Thrombolytic drugs, such as tissue plasminogen activator (tPA), can dissolve existing thrombi and restore microvascular perfusion. However, thrombolytic drugs are associated with a risk of bleeding.
5.5 Personalized Medicine Approaches
It is increasingly recognized that microvascular dysfunction is a heterogeneous condition, and treatment should be tailored to the individual patient. Personalized medicine approaches, based on genetic profiling, biomarker analysis, and imaging techniques, can help to identify patients who are most likely to benefit from specific therapies.
Many thanks to our sponsor Esdebe who helped us prepare this research report.
6. Conclusion
The microvasculature plays a crucial role in maintaining tissue homeostasis and responding to various stimuli. Microvascular damage is a prominent feature of many diseases, contributing significantly to their pathogenesis and progression. While significant progress has been made in understanding the mechanisms of microvascular damage and developing therapeutic strategies, several challenges remain. Emerging technologies, such as super-resolution microscopy, photoacoustic imaging, and AI, hold promise for improving the assessment of microvascular health. Personalized medicine approaches, based on genetic profiling, biomarker analysis, and imaging techniques, can help to tailor treatment to the individual patient. Future research should focus on developing novel therapeutic strategies that target specific aspects of microvascular dysfunction and on identifying biomarkers that can predict treatment response. By advancing our understanding of the microvasculature, we can develop more effective therapies to prevent and treat microvascular-related diseases and improve patient outcomes.
Many thanks to our sponsor Esdebe who helped us prepare this research report.
References
[1] Reitsma, S., et al. “The endothelial glycocalyx: Composition, functions, and visualization.” European Journal of Physiology 471.2 (2019): 233-246.
[2] Engelmann, B., et al. “Thromboinflammation in cardiovascular disease.” European Heart Journal 42.27 (2021): 2643-2652.
[3] Varga, Z., et al. “Endothelial cell infection and endotheliitis in COVID-19.” The Lancet 395.10234 (2020): 1417-1418.
The discussion of specific examples of microvascular damage in diseases is fascinating. Considering the role of SARS-CoV-2 in endothelial cell infection, how might pre-existing conditions impacting the microvasculature influence the severity and long-term outcomes of COVID-19?
That’s a great point! Pre-existing conditions, especially those affecting the microvasculature, could certainly exacerbate COVID-19’s severity. The virus’s impact on endothelial cells might be amplified in individuals with compromised microvascular function, potentially leading to more severe thromboinflammation and long-term complications. Further research is definitely needed in this area!
Editor: MedTechNews.Uk
Thank you to our Sponsor Esdebe
So, if microvascular occlusion is a critical consequence of damage, are we focusing enough on early detection methods to prevent the “point of no return” in tissue ischemia? Maybe some kind of micro-plumbing check-up?
That’s a fantastic analogy! A “micro-plumbing check-up” is exactly what we need. Early detection is key, and your comment highlights the need for more sensitive and readily available diagnostic tools. Perhaps advancements in non-invasive imaging will make this a reality soon, shifting from reaction to prevention. Thanks for sparking this thought!
Editor: MedTechNews.Uk
Thank you to our Sponsor Esdebe
Given the challenges in current microvascular imaging, what are the prospects for combining multiple modalities, such as OCT and AI-enhanced analysis, to provide a more comprehensive and clinically relevant assessment of microvascular health in various disease states?
That’s an excellent question! The future definitely looks promising for multi-modal approaches. Combining OCT’s high-resolution imaging with AI’s analytical power could revolutionize microvascular assessment. AI could help overcome limitations in current techniques by identifying subtle changes indicative of disease, leading to earlier and more accurate diagnoses. Imagine AI helping us create a risk profile based on multiple imaging modalities!
Editor: MedTechNews.Uk
Thank you to our Sponsor Esdebe