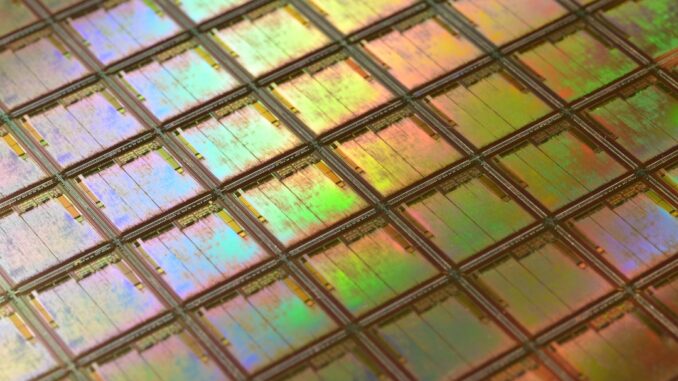
The Ever-Shrinking World: A Comprehensive Review of Miniaturization Across Disciplines
Abstract
Miniaturization, the relentless pursuit of creating smaller and more efficient devices and systems, has become a defining trend across a multitude of scientific and engineering disciplines. This report provides a comprehensive overview of the current state-of-the-art in miniaturization, spanning from microelectronics and micro-robotics to microfluidics and materials science. We delve into the fundamental principles that govern the behavior of systems at small scales, highlighting the challenges and opportunities that arise from scaling down. The report explores the diverse applications of miniaturized technologies in areas such as medicine, environmental monitoring, consumer electronics, and energy storage. Furthermore, we examine the manufacturing techniques employed to create these intricate devices, including microfabrication, 3D printing, and self-assembly. The ethical and societal implications of widespread miniaturization are also considered, concluding with a discussion of future trends and the potential for even smaller, nanoscale systems.
1. Introduction
Miniaturization, at its core, is the art and science of making things smaller. This simple goal has driven innovation across countless fields, leading to transformative advancements in technology and a profound impact on society. The underlying motivation behind miniaturization is multifaceted. Smaller devices often consume less power, operate faster, are more portable, and can be deployed in previously inaccessible locations. This drive for increased functionality within a reduced footprint has fueled a relentless pursuit of scaling down components, devices, and entire systems.
The benefits of miniaturization extend beyond simple size reduction. As systems become smaller, their surface area to volume ratio increases, leading to enhanced reactivity and sensitivity. This phenomenon is exploited in sensors, catalysts, and drug delivery systems. Furthermore, miniaturization often enables parallelization and automation, allowing for high-throughput screening, rapid prototyping, and efficient manufacturing. The development of microelectromechanical systems (MEMS) and microfluidic devices, for instance, has revolutionized fields such as biomedical diagnostics and chemical analysis.
This report provides a broad overview of miniaturization, examining its impact across several key disciplines. We will explore the fundamental principles that govern behavior at small scales, the materials and manufacturing techniques used to create miniature devices, and the diverse applications of these technologies. The report also considers the ethical and societal implications of miniaturization, concluding with a discussion of future trends and the potential for further advancements in the field. Miniaturization is not merely a scaling down of existing technologies; it necessitates a fundamental rethinking of design principles and manufacturing processes to fully realize the potential of small-scale systems.
2. Fundamental Principles and Challenges of Miniaturization
Scaling down a system is not a linear process; the physical laws that govern macroscopic behavior often differ significantly at smaller scales. Understanding these fundamental principles is crucial for designing and fabricating effective miniature devices. Several key phenomena become increasingly important as dimensions shrink:
- Surface Effects: As the surface area to volume ratio increases, surface phenomena such as surface tension, adhesion, and friction become dominant. These effects can significantly impact the performance of microfluidic devices, MEMS, and nanoscale materials. Controlling surface properties through surface modification and coatings is often essential for optimizing device performance.
- Quantum Mechanics: At the nanoscale, quantum mechanical effects such as quantum tunneling and quantum confinement become significant. These effects can be exploited to create novel electronic and optical devices, but they also pose challenges for device fabrication and reliability. For instance, quantum tunneling can lead to leakage currents in transistors, limiting their performance.
- Stochasticity: At small scales, thermal fluctuations and random events become more pronounced. This stochasticity can affect the precision and reliability of miniature devices, particularly in applications such as drug delivery and single-molecule detection. Statistical analysis and control strategies are often required to mitigate the effects of stochasticity.
- Fluid Dynamics: The behavior of fluids at small scales is governed by different principles than at macroscopic scales. Viscous forces become dominant over inertial forces, leading to laminar flow and high pressure drops in microfluidic channels. Understanding these effects is crucial for designing efficient microfluidic devices for chemical analysis and biomedical applications.
In addition to these fundamental principles, several technical challenges must be addressed to achieve successful miniaturization:
- Fabrication: Creating intricate structures with nanoscale precision requires sophisticated fabrication techniques such as photolithography, electron beam lithography, and focused ion beam milling. These techniques are often expensive and time-consuming, limiting the widespread adoption of nanoscale devices.
- Assembly: Assembling individual components into functional systems becomes increasingly difficult at smaller scales. Self-assembly techniques, which rely on the spontaneous organization of components based on their inherent properties, offer a promising solution to this challenge.
- Power Management: Supplying power to miniature devices without significantly increasing their size or weight is a major challenge. Wireless power transfer, energy harvesting, and micro-batteries are being developed to address this issue.
- Integration: Integrating different functional components into a single miniature device requires careful design and materials selection. Compatibility issues, such as thermal expansion mismatch and chemical incompatibility, must be addressed to ensure reliable device operation.
3. Materials and Manufacturing Techniques for Miniaturized Devices
The selection of appropriate materials and manufacturing techniques is critical for the successful fabrication of miniaturized devices. Traditional materials such as silicon, glass, and polymers are widely used in microfabrication, but new materials with tailored properties are constantly being developed to meet the demands of advanced applications.
- Silicon: Silicon is the workhorse of the microelectronics industry, and it is also widely used in MEMS and microfluidics. Its well-established fabrication processes, excellent mechanical properties, and biocompatibility make it an attractive material for a wide range of applications.
- Polymers: Polymers such as polydimethylsiloxane (PDMS), poly(methyl methacrylate) (PMMA), and SU-8 are commonly used in microfluidics due to their ease of fabrication, low cost, and biocompatibility. Polymers can be molded, etched, and patterned using a variety of techniques, allowing for the creation of complex microfluidic structures.
- Metals: Metals such as gold, platinum, and titanium are used in microelectronics and MEMS for their excellent electrical conductivity, chemical inertness, and mechanical strength. Thin films of metals can be deposited using sputtering, evaporation, and electroplating.
- Nanomaterials: Nanomaterials such as carbon nanotubes, graphene, and nanoparticles offer unique properties that can be exploited in miniature devices. Carbon nanotubes, for example, exhibit exceptional electrical conductivity and mechanical strength, making them ideal for use in sensors and transistors. Graphene, a two-dimensional sheet of carbon atoms, has attracted significant attention for its high electron mobility and potential applications in flexible electronics.
Several manufacturing techniques are used to create miniaturized devices, including:
- Photolithography: Photolithography is a widely used technique for patterning thin films on substrates. A photoresist layer is exposed to ultraviolet light through a mask, and the exposed regions are selectively removed, leaving behind a patterned film. This technique is used to create transistors, microfluidic channels, and other microstructures.
- Electron Beam Lithography: Electron beam lithography (EBL) offers higher resolution than photolithography, allowing for the fabrication of nanoscale structures. An electron beam is used to selectively expose a resist layer, which is then developed to create a patterned film. EBL is used to create high-resolution masks for photolithography and to fabricate nanoscale devices directly.
- Focused Ion Beam Milling: Focused ion beam (FIB) milling is a technique that uses a focused beam of ions to remove material from a substrate. FIB milling can be used to create complex three-dimensional structures with nanoscale precision. It is often used for prototyping and for modifying existing devices.
- Micro-molding: Micro-molding is a technique for fabricating microstructures by molding a polymer or other material into a mold. The mold can be created using photolithography, EBL, or FIB milling. Micro-molding is a cost-effective technique for mass-producing microfluidic devices and other microstructures.
- 3D Printing: Additive manufacturing techniques, commonly known as 3D printing, are increasingly used to create microscale structures. Techniques such as stereolithography, two-photon polymerization, and direct ink writing allow for the layer-by-layer fabrication of complex three-dimensional structures with high precision.
- Self-Assembly: Self-assembly is a process in which components spontaneously organize themselves into ordered structures based on their inherent properties. Self-assembly can be used to create nanoscale devices and materials with unique properties. This approach is particularly attractive for large-scale manufacturing of complex structures.
4. Applications of Miniaturization Across Disciplines
Miniaturization has revolutionized a wide range of disciplines, leading to transformative advancements in technology and a profound impact on society. Some key applications include:
- Medicine: Miniaturized medical devices such as micro-robots, micro-endoscopes, and lab-on-a-chip systems are transforming diagnostics, drug delivery, and surgery. Micro-robots can navigate through the body to deliver drugs directly to tumors or to perform minimally invasive surgery in hard-to-reach areas. Lab-on-a-chip systems can perform rapid and accurate diagnostics at the point of care, enabling early detection of diseases and personalized medicine.
- Environmental Monitoring: Miniaturized sensors can be deployed in the environment to monitor air and water quality, detect pollutants, and track climate change. These sensors can be integrated into wireless sensor networks, allowing for real-time monitoring of environmental conditions over large areas.
- Consumer Electronics: Miniaturization has enabled the development of smartphones, tablets, and other portable electronic devices with unprecedented functionality. Miniaturized sensors, microprocessors, and memory chips have allowed for the integration of a wide range of features into these devices, including cameras, GPS, and accelerometers.
- Energy Storage: Miniaturized batteries and fuel cells are being developed to power portable electronic devices and micro-robots. These devices require high energy density and long lifespans. Microfabrication techniques are used to create micro-batteries with high surface area and low internal resistance.
- Aerospace: Miniaturized sensors and actuators are used in aerospace applications for navigation, control, and communication. Miniaturized satellites, known as CubeSats, are being developed for Earth observation, scientific research, and telecommunications. These satellites are much smaller and cheaper than traditional satellites, allowing for more frequent and cost-effective space missions.
- Robotics: Microrobotics is a rapidly growing field that focuses on the development of robots with dimensions ranging from millimeters to micrometers. These tiny robots can be used for a variety of applications, including drug delivery, minimally invasive surgery, and environmental monitoring. The key challenge in microrobotics is to develop efficient and precise control mechanisms for navigating and manipulating objects at small scales.
5. Ethical and Societal Implications of Miniaturization
While miniaturization offers tremendous potential benefits, it also raises several ethical and societal concerns that must be carefully considered. These concerns include:
- Privacy: Miniaturized sensors and cameras can be easily concealed, raising concerns about privacy and surveillance. The widespread deployment of these devices could lead to a loss of privacy and the potential for misuse of personal information.
- Security: Miniaturized devices can be vulnerable to hacking and cyberattacks. A compromised miniature device could be used to steal data, disrupt critical infrastructure, or even cause physical harm.
- Environmental Impact: The production and disposal of miniaturized devices can have a significant environmental impact. The manufacturing processes often involve the use of hazardous chemicals and materials, and the disposal of electronic waste can lead to pollution and resource depletion.
- Job Displacement: The automation and increased efficiency enabled by miniaturized technologies could lead to job displacement in certain sectors. It is important to consider the social and economic consequences of these changes and to develop strategies for mitigating their impact.
- Dual Use: Many miniaturized technologies have both civilian and military applications. It is important to ensure that these technologies are not used for malicious purposes, such as the development of autonomous weapons or surveillance systems.
- Accessibility and Equity: The benefits of miniaturization should be accessible to all members of society, regardless of their income or location. It is important to ensure that the costs of these technologies do not create further inequalities. For example, if miniaturized diagnostics become commonplace but are not affordable to a significant portion of the population, this could exacerbate existing health disparities.
Addressing these ethical and societal concerns requires a multidisciplinary approach involving scientists, engineers, policymakers, and the public. It is important to develop ethical guidelines and regulations to ensure that miniaturized technologies are used responsibly and for the benefit of society.
6. Future Trends and the Nanoscale Frontier
The field of miniaturization is constantly evolving, driven by advances in materials science, manufacturing techniques, and computing power. Several key trends are shaping the future of miniaturization:
- Nanoscale Devices: The ultimate goal of miniaturization is to create devices at the nanoscale, where individual atoms and molecules are used as building blocks. Nanoscale devices offer the potential for unprecedented functionality and performance, but they also pose significant technical challenges. Research in areas such as molecular electronics, nano-robotics, and self-assembling nanostructures is paving the way for the development of functional nanoscale devices.
- Integration and System-on-a-Chip: Integrating multiple functional components into a single miniature device, known as system-on-a-chip (SoC), is a key trend in miniaturization. SoC devices offer improved performance, reduced power consumption, and lower cost compared to discrete components. This trend is particularly evident in the development of microprocessors, sensors, and biomedical devices.
- Artificial Intelligence and Machine Learning: Integrating artificial intelligence (AI) and machine learning (ML) into miniature devices is enabling new capabilities such as autonomous operation, adaptive behavior, and personalized diagnostics. AI-powered miniature robots can navigate complex environments and perform tasks without human intervention. ML algorithms can be used to analyze data from miniaturized sensors and to predict outcomes in areas such as healthcare and environmental monitoring.
- Biocompatible and Biodegradable Devices: Developing biocompatible and biodegradable miniature devices is essential for applications in medicine and environmental monitoring. These devices can be implanted in the body or deployed in the environment without causing harm, and they can eventually degrade and be absorbed by the body or the environment. This trend is particularly important for drug delivery, tissue engineering, and environmental remediation.
- Flexible and Stretchable Electronics: Flexible and stretchable electronics are enabling the development of wearable sensors, implantable devices, and flexible displays. These devices can conform to the shape of the body or other surfaces, making them more comfortable and versatile than rigid devices. This area relies heavily on the development of novel materials and fabrication techniques that can withstand bending and stretching.
The future of miniaturization holds immense promise for revolutionizing technology and improving the quality of life. By addressing the ethical and societal concerns and by continuing to push the boundaries of materials science, manufacturing techniques, and computing power, we can unlock the full potential of miniaturization and create a more sustainable and prosperous future.
7. Conclusion
Miniaturization stands as a testament to human ingenuity, a relentless pursuit of efficiency and capability within ever-shrinking dimensions. This report has highlighted the profound impact of miniaturization across diverse fields, from medicine and environmental monitoring to consumer electronics and aerospace. We have explored the fundamental principles governing behavior at small scales, the innovative materials and manufacturing techniques employed, and the wide-ranging applications that have emerged.
While the benefits of miniaturization are undeniable, we must also acknowledge and address the ethical and societal implications it presents. Privacy concerns, security vulnerabilities, environmental impact, and potential job displacement require careful consideration and proactive solutions. A multidisciplinary approach, involving scientists, engineers, policymakers, and the public, is crucial for ensuring the responsible development and deployment of miniaturized technologies.
The future of miniaturization points towards the nanoscale frontier, where individual atoms and molecules become the building blocks of functional devices. Integration with artificial intelligence, the development of biocompatible and biodegradable materials, and the emergence of flexible electronics are driving innovation and opening up new possibilities. As we continue to push the boundaries of what is possible, it is essential to remain mindful of the broader societal implications and to strive for a future where miniaturization contributes to a more sustainable, equitable, and prosperous world.
References
- Rogers, J. A., & Someya, T. (2010). Flexible electronics. Proceedings of the National Academy of Sciences, 107(23), 10272-10273.
- Whitesides, G. M. (2006). The origins and the future of microfluidics. Nature, 442(7101), 368-373.
- Li, J., Tang, B., & Cai, Q. (2017). Recent advances in micro/nanorobots: From fabrication to biomedical applications. Journal of Materials Chemistry B, 5(45), 8703-8732.
- Ratner, M. A., & Ratner, D. (2002). Nanotechnology: A gentle introduction to the next big idea. Pearson Education.
- Madou, M. J. (2018). Fundamentals of microfabrication and nanotechnology. CRC press.
- Bishop, K. J. M., Wilmer, C. E., Soh, S., & Grzybowski, B. A. (2009). Nanoscale materials and self-assembly. Small, 5(14), 1600-1624.
- El-Sayed, M. A. (2001). The chemistry and physics of nanoparticles. The Journal of Physical Chemistry B, 105(18), 3438-3448.
- Schmidt, O. G., & Eberbeck, D. (2010). Micro-and nanoscale fabrication for biomedical applications. Advanced Materials, 22(34), 3522-3536.
- Daniel, M. C., & Astruc, D. (2004). Gold nanoparticles: assembly, supramolecular chemistry, quantum-size-related properties, and applications toward biology, catalysis, and nanotechnology. Chemical Reviews, 104(1), 293-346.
- Ferrari, M. (2005). Cancer nanotechnology: opportunities and challenges. Nature Reviews Cancer, 5(3), 161-171.
So, if we shrink everything down enough, will my house cleaning chores also miniaturize? Asking for a friend… who is definitely me. Also, where can I invest in these tiny robot vacuums?
That’s a fantastic question! The idea of miniaturized chores is certainly appealing. While fully miniaturized cleaning might be a future dream, think about the advancements in micro-robotics. Perhaps, someday, we will have nanobots doing all our chores. Regarding tiny robot vacuums, keep an eye on companies developing MEMS-based devices!
Editor: MedTechNews.Uk
Thank you to our Sponsor Esdebe
This report highlights the exciting possibilities of miniaturization, particularly in medicine. The potential for targeted drug delivery using micro-robots could revolutionize treatments and improve patient outcomes. Further research in biocompatible materials will be crucial to realize these applications.
Thank you for your comment! I am glad you found the section on medicine interesting. I agree that targeted drug delivery is an exciting prospect! As biocompatible materials improve, do you think micro-robot surgery will become more mainstream?
Editor: MedTechNews.Uk
Thank you to our Sponsor Esdebe
Miniaturization in medicine – next thing you know, we’ll have nanobots performing internal audits of our vital organs and sending performance reviews directly to our insurance companies. The future is wild, and probably expensive.
That’s a great point! The cost-effectiveness of miniaturization is definitely something worth exploring. While the initial development might be expensive, do you think mass production and increased efficiency could eventually drive down the cost of these technologies, making them more accessible?
Editor: MedTechNews.Uk
Thank you to our Sponsor Esdebe