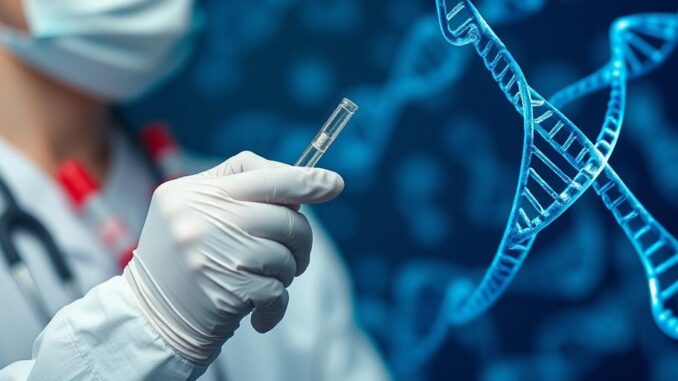
The Expanding Landscape of Genetic Testing: Applications, Challenges, and Future Directions
Many thanks to our sponsor Esdebe who helped us prepare this research report.
Abstract
Genetic testing has revolutionized medical practice, moving from a niche diagnostic tool to an increasingly integral component of personalized medicine. This report provides a comprehensive overview of the current state of genetic testing, encompassing its diverse applications, underlying technologies, analytical and clinical validity and utility, ethical and societal implications, and the challenges associated with data interpretation and implementation. We explore the evolution of genetic testing methodologies, from single-gene assays to comprehensive genomic sequencing, and their impact across various medical specialties, with particular emphasis on applications beyond inherited aortopathies to include pharmacogenomics, cancer diagnostics and prognostics, and personalized risk assessment. Furthermore, we delve into the complexities of variant interpretation, the importance of robust quality control measures, the evolving regulatory landscape, and the critical need for enhanced patient education and equitable access. Finally, we discuss emerging trends and future directions, including the integration of artificial intelligence, the use of polygenic risk scores, and the potential for proactive genomic screening, highlighting the transformative potential of genetic testing while acknowledging the need for careful consideration of its limitations and ethical implications.
Many thanks to our sponsor Esdebe who helped us prepare this research report.
1. Introduction
The human genome project, completed in 2003, marked a paradigm shift in biomedical research and clinical medicine. The ability to decipher the complete human genetic code has paved the way for the development and implementation of genetic testing across a wide range of clinical settings. Genetic testing encompasses a diverse array of methodologies designed to analyze an individual’s DNA, RNA, or chromosomes to identify alterations that may predispose them to disease, inform treatment decisions, or predict responses to medication. The scope of genetic testing has expanded exponentially in recent years, driven by advancements in sequencing technologies, bioinformatics, and our understanding of the genetic basis of human diseases. This report aims to provide a comprehensive overview of the current landscape of genetic testing, highlighting its applications, challenges, and future directions, with the goal of informing researchers, clinicians, and policymakers alike.
Many thanks to our sponsor Esdebe who helped us prepare this research report.
2. Types of Genetic Tests and Technologies
Genetic testing encompasses a wide range of methodologies, each with its own strengths and limitations. The choice of testing method depends on the clinical question being addressed, the suspected underlying genetic mechanism, and the availability of resources. Below are some of the most commonly used types of genetic tests:
-
2.1 Single-Gene Testing: This involves analyzing a specific gene known to be associated with a particular disease or trait. It is often used when there is a strong clinical suspicion of a specific genetic disorder or when family history suggests a specific gene mutation is responsible.
-
2.2 Gene Panels: Gene panels analyze multiple genes simultaneously, typically selected based on their known association with a specific clinical phenotype or disease category. These panels are more efficient and cost-effective than single-gene testing when multiple genes could be responsible for the condition being investigated.
-
2.3 Exome Sequencing (ES): ES targets the protein-coding regions of the genome (the exome), which constitute approximately 1-2% of the entire genome but contain approximately 85% of disease-causing variants. ES is a powerful tool for identifying novel disease genes and diagnosing rare and undiagnosed genetic disorders.
-
2.4 Whole-Genome Sequencing (WGS): WGS sequences the entire genome, including both coding and non-coding regions. WGS offers the most comprehensive view of an individual’s genetic makeup and can identify variants in regulatory regions, introns, and other non-coding sequences that may contribute to disease. While initially more expensive and complex to analyze than ES, WGS is becoming increasingly affordable and accessible.
-
2.5 Chromosomal Microarray Analysis (CMA): CMA detects copy number variations (CNVs), which are gains or losses of large segments of DNA. CNVs can cause a variety of developmental and intellectual disabilities, as well as certain types of cancer.
-
2.6 Non-Invasive Prenatal Testing (NIPT): NIPT analyzes cell-free fetal DNA circulating in the maternal bloodstream to screen for common chromosomal aneuploidies, such as Down syndrome (trisomy 21), Edwards syndrome (trisomy 18), and Patau syndrome (trisomy 13).
-
2.7 Pharmacogenomic Testing: Pharmacogenomic testing analyzes genes that influence drug metabolism, transport, and receptor binding to predict an individual’s response to specific medications. This can help clinicians optimize drug selection and dosage to improve treatment efficacy and reduce the risk of adverse drug reactions.
The technological advancements driving these tests are significant. Next-generation sequencing (NGS) has become the workhorse of modern genetic testing, enabling high-throughput, cost-effective sequencing of DNA and RNA. NGS technologies such as Illumina, Ion Torrent, and PacBio have revolutionized the field, allowing for the rapid and comprehensive analysis of genetic information. PCR-based methods remain crucial for targeted sequencing and validation. Furthermore, advances in bioinformatics tools and databases are essential for analyzing and interpreting the vast amounts of data generated by these technologies.
Many thanks to our sponsor Esdebe who helped us prepare this research report.
3. Applications of Genetic Testing in Medicine
Genetic testing has found broad applications across nearly all medical specialties. Its use extends beyond the diagnosis of rare inherited disorders, increasingly playing a role in personalized medicine and risk assessment.
-
3.1 Cardiology: Beyond aortopathies, genetic testing is instrumental in identifying individuals at risk for hypertrophic cardiomyopathy, long QT syndrome, and other inherited cardiac conditions. Early diagnosis and management can prevent sudden cardiac death and improve patient outcomes.
-
3.2 Oncology: Genetic testing is increasingly used to identify individuals at high risk for developing cancer (e.g., BRCA1/2 mutations in breast and ovarian cancer), guide treatment decisions (e.g., EGFR mutations in lung cancer), and monitor treatment response (e.g., circulating tumor DNA analysis). Liquid biopsies are transforming cancer diagnostics by enabling non-invasive monitoring of tumor evolution.
-
3.3 Neurology: Genetic testing is used to diagnose and manage neurological disorders such as Huntington’s disease, spinal muscular atrophy, and Duchenne muscular dystrophy. It is also playing an increasingly important role in the diagnosis of epilepsy and autism spectrum disorder.
-
3.4 Pediatrics: Genetic testing is essential for diagnosing and managing a wide range of pediatric disorders, including congenital anomalies, intellectual disability, and metabolic disorders. Newborn screening programs use genetic testing to identify infants at risk for certain treatable conditions.
-
3.5 Obstetrics and Gynecology: Genetic testing is used for prenatal screening and diagnosis, carrier screening for recessive genetic disorders, and preimplantation genetic diagnosis (PGD) to select embryos free from specific genetic mutations.
-
3.6 Pharmacogenomics: As mentioned earlier, pharmacogenomic testing helps clinicians tailor medication choices and dosages based on an individual’s genetic profile. This is particularly relevant in fields such as psychiatry, cardiology, and oncology, where drug response can vary significantly among individuals.
-
3.7 Infectious Disease: Genetic testing is becoming important in infectious disease for identifying pathogens, understanding resistance to antiviral medications, and tracing outbreaks of bacterial and viral diseases.
-
3.8 Direct-to-Consumer Genetic Testing (DTC-GT): DTC-GT is offered directly to consumers without the involvement of a healthcare provider. While DTC-GT can provide individuals with information about their ancestry, traits, and disease risks, the clinical validity and utility of these tests can vary considerably. Concerns have been raised about the accuracy of DTC-GT results, the potential for misinterpretation, and the lack of adequate genetic counseling.
Many thanks to our sponsor Esdebe who helped us prepare this research report.
4. Analytical and Clinical Validity and Utility
Assessing the validity and utility of genetic tests is crucial to ensure that they provide accurate and meaningful information that can be used to improve patient outcomes.
-
4.1 Analytical Validity: Analytical validity refers to the ability of a genetic test to accurately and reliably detect the presence or absence of a specific genetic variant. Analytical validity is typically assessed by measuring the sensitivity, specificity, accuracy, and reproducibility of the test. Rigorous quality control measures, including the use of positive and negative controls, are essential to ensure analytical validity.
-
4.2 Clinical Validity: Clinical validity refers to the ability of a genetic test to accurately predict the presence or absence of a specific disease or trait. Clinical validity is typically assessed by measuring the sensitivity, specificity, positive predictive value (PPV), and negative predictive value (NPV) of the test. Clinical validity can be influenced by factors such as the prevalence of the disease in the population being tested and the penetrance of the genetic variant.
-
4.3 Clinical Utility: Clinical utility refers to the extent to which a genetic test improves patient outcomes. Clinical utility is assessed by determining whether the test results lead to changes in clinical management that result in improved health outcomes, such as reduced morbidity, mortality, or healthcare costs. Demonstrating clinical utility can be challenging, particularly for genetic tests that are used to predict disease risk rather than diagnose existing conditions.
-
4.4 Regulatory Oversight: The regulation of genetic testing varies across different countries. In the United States, genetic tests are regulated by the Food and Drug Administration (FDA) and the Centers for Medicare & Medicaid Services (CMS). The FDA regulates the analytical validity of genetic tests, while CMS regulates the clinical validity and utility of genetic tests. The European Union (EU) regulates genetic testing through the In Vitro Diagnostic Regulation (IVDR), which sets standards for the quality, safety, and performance of in vitro diagnostic devices.
Many thanks to our sponsor Esdebe who helped us prepare this research report.
5. Ethical Considerations
The increasing use of genetic testing raises a number of ethical considerations that must be addressed to ensure that genetic testing is used responsibly and ethically.
-
5.1 Privacy: Genetic information is highly personal and sensitive, and individuals have a right to privacy regarding their genetic information. Genetic testing raises concerns about the potential for unauthorized access to and misuse of genetic information. Strong privacy protections are needed to prevent genetic discrimination in employment, insurance, and other areas of life. The Genetic Information Nondiscrimination Act (GINA) in the United States provides some protection against genetic discrimination, but gaps remain.
-
5.2 Informed Consent: Individuals should be fully informed about the risks and benefits of genetic testing before deciding whether to undergo testing. Informed consent should include information about the purpose of the test, the potential results, the limitations of the test, and the implications of the results for the individual and their family members. It should also include details regarding how the data will be used and stored, and who will have access to it. This necessitates clear and accessible communication, tailored to the individual’s level of understanding.
-
5.3 Genetic Counseling: Genetic counseling plays a crucial role in helping individuals understand the implications of genetic testing results and make informed decisions about their healthcare. Genetic counselors can provide information about the inheritance patterns of genetic disorders, the risks of passing on genetic mutations to future generations, and the available options for managing genetic conditions. Genetic counselors should be an integral part of the genetic testing process.
-
5.4 Equity and Access: Genetic testing should be accessible to all individuals, regardless of their socioeconomic status or geographic location. However, genetic testing is often expensive and may not be covered by insurance, limiting access for many individuals. Efforts are needed to ensure that genetic testing is affordable and accessible to all who could benefit from it.
-
5.5 Incidental Findings: Genetic testing can sometimes reveal incidental findings, which are genetic variants that are not related to the primary reason for testing but may have implications for an individual’s health. The American College of Medical Genetics and Genomics (ACMG) recommends that laboratories actively search for and report certain incidental findings, such as mutations in genes associated with cancer predisposition. However, the reporting of incidental findings raises ethical questions about the duty to warn and the potential for psychological distress.
-
5.6 Germline Editing: CRISPR-Cas9 and other gene-editing technologies have the potential to correct disease-causing mutations in germ cells (sperm and eggs) or embryos. However, germline editing raises significant ethical concerns, including the potential for unintended consequences, the risk of off-target effects, and the potential for misuse. While somatic cell gene therapy is generally considered acceptable, germline editing remains highly controversial.
Many thanks to our sponsor Esdebe who helped us prepare this research report.
6. Challenges in Data Interpretation and Variant Classification
One of the most significant challenges in genetic testing is the interpretation of genetic variants. The human genome is highly variable, and many genetic variants have no known effect on health. Distinguishing between pathogenic variants (those that cause disease) and benign variants (those that do not cause disease) can be challenging, particularly for rare and novel variants.
The ACMG and the Association for Molecular Pathology (AMP) have developed guidelines for the interpretation of sequence variants. These guidelines classify variants into five categories: pathogenic, likely pathogenic, uncertain significance, likely benign, and benign. However, the application of these guidelines can be subjective, and different laboratories may classify the same variant differently. This highlights the need for standardized variant classification criteria and collaborative efforts to share variant data.
Furthermore, the interpretation of genetic variants can be influenced by factors such as ethnicity and ancestry. Some genetic variants are more common in certain populations than others, and the clinical significance of a variant may differ depending on the individual’s genetic background. Large-scale population studies are needed to better understand the distribution and clinical significance of genetic variants in diverse populations.
Many thanks to our sponsor Esdebe who helped us prepare this research report.
7. Cost-Effectiveness Analyses
The cost-effectiveness of genetic testing is an important consideration for healthcare policymakers and payers. Cost-effectiveness analyses compare the costs and benefits of genetic testing to other diagnostic or treatment strategies. The results of cost-effectiveness analyses can inform decisions about whether to reimburse genetic testing and which types of genetic tests to prioritize.
The cost-effectiveness of genetic testing can vary depending on the clinical context, the type of genetic test, and the prevalence of the disease in the population being tested. In some cases, genetic testing can be highly cost-effective, particularly when it leads to earlier diagnosis, more effective treatment, or prevention of disease. For example, genetic testing for BRCA1/2 mutations in women at high risk for breast and ovarian cancer has been shown to be cost-effective because it can lead to earlier detection and prevention of cancer. However, in other cases, genetic testing may not be cost-effective, particularly when it is used for screening low-risk individuals or when it does not lead to changes in clinical management.
The declining cost of sequencing technologies has made genetic testing more affordable, but the cost of data analysis and interpretation remains a significant barrier. Further research is needed to develop more efficient and cost-effective methods for analyzing and interpreting genetic data.
Many thanks to our sponsor Esdebe who helped us prepare this research report.
8. Impact of Genetic Testing on Patient Outcomes and Healthcare Decision-Making
Genetic testing has the potential to improve patient outcomes by enabling earlier diagnosis, more effective treatment, and personalized risk assessment. Genetic testing can also inform healthcare decision-making by providing information about the likelihood of developing a disease, the potential response to treatment, and the risk of passing on genetic mutations to future generations.
Studies have shown that genetic testing can lead to changes in clinical management in a significant proportion of patients. These changes can include earlier initiation of treatment, modification of treatment regimens, and implementation of preventive measures. For example, genetic testing for Lynch syndrome, a hereditary cancer syndrome, can lead to increased surveillance for colon cancer and other cancers, which can improve survival rates.
However, the impact of genetic testing on patient outcomes can be complex and may vary depending on the individual’s psychological makeup, social support, and access to healthcare. Some individuals may experience anxiety or distress as a result of receiving genetic testing results, particularly if the results indicate an increased risk for disease. Comprehensive genetic counseling and psychological support are essential to help individuals cope with the emotional impact of genetic testing.
Furthermore, the integration of genetic information into clinical practice requires healthcare providers to have adequate knowledge and training in genetics. Many healthcare providers lack the necessary training to interpret genetic testing results and apply them to patient care. Efforts are needed to improve genetics education for healthcare providers at all levels.
Many thanks to our sponsor Esdebe who helped us prepare this research report.
9. Future Directions
The field of genetic testing is rapidly evolving, driven by technological advancements, increasing knowledge of the human genome, and growing demand for personalized medicine. Several emerging trends are poised to shape the future of genetic testing.
-
9.1 Artificial Intelligence (AI): AI is increasingly being used to analyze and interpret genetic data, predict disease risk, and identify potential drug targets. Machine learning algorithms can be trained to recognize patterns in genetic data that are associated with disease, even when the underlying mechanisms are not fully understood. AI has the potential to accelerate the development of new diagnostic and therapeutic strategies.
-
9.2 Polygenic Risk Scores (PRS): PRS combine the effects of multiple genetic variants to estimate an individual’s risk for a complex disease. PRS are based on the concept that many common diseases are influenced by a large number of genetic variants, each with a small effect. PRS have the potential to improve risk prediction for common diseases such as heart disease, diabetes, and Alzheimer’s disease. However, the accuracy of PRS can vary depending on the disease, the population being tested, and the number of genetic variants included in the score. Careful validation is needed before PRS can be widely implemented in clinical practice.
-
9.3 Proactive Genomic Screening: Proactive genomic screening involves offering genetic testing to individuals regardless of their personal or family history of disease. This approach aims to identify individuals at high risk for preventable or treatable conditions before they develop symptoms. Proactive genomic screening has the potential to improve health outcomes by enabling early intervention and personalized prevention strategies. However, proactive genomic screening raises ethical questions about the duty to warn, the potential for psychological distress, and the cost-effectiveness of screening large populations. Careful consideration is needed before proactive genomic screening is widely implemented.
-
9.4 Integration with Electronic Health Records (EHRs): The seamless integration of genetic information into EHRs is essential for enabling personalized medicine. This requires standardized data formats, interoperable systems, and secure data sharing protocols. EHR-integrated genetic information can facilitate clinical decision support, pharmacogenomic testing, and precision oncology. However, the integration of genetic information into EHRs also raises privacy and security concerns that must be addressed.
Many thanks to our sponsor Esdebe who helped us prepare this research report.
10. Conclusion
Genetic testing has transformed medical practice, providing unprecedented insights into the genetic basis of human diseases and enabling personalized approaches to diagnosis, treatment, and prevention. The field is rapidly evolving, driven by technological advancements, increasing knowledge of the human genome, and growing demand for personalized medicine. While genetic testing holds tremendous promise for improving patient outcomes, it also raises significant ethical, social, and economic challenges. Careful consideration of these challenges is needed to ensure that genetic testing is used responsibly and equitably to benefit all individuals.
Many thanks to our sponsor Esdebe who helped us prepare this research report.
References
- Landrum, M. J., Lee, J. M., Riley, G. R., et al. (2016). ClinVar: public archive of interpretations of clinically relevant variants. Nucleic Acids Research, 44(D1), D862–D868.
- Richards, S., Aziz, N., Bale, S., et al. (2015). Standards and guidelines for the interpretation of sequence variants: a joint consensus recommendation of the American College of Medical Genetics and Genomics and the Association for Molecular Pathology. Genetics in Medicine, 17(5), 405–424.
- Manolio, T. A., Chisholm, R. L., Ozenberger, B., et al. (2009). Genome-wide association studies and assessment of the risk of disease. New England Journal of Medicine, 361(7), 666–678.
- Berg, J. S., Khoury, M. J., & Evans, J. P. (2011). Deploying whole genome sequencing in clinical practice and public health: meeting the challenge one bin at a time. Genetics in Medicine, 13(6), 499–504.
- Khoury, M. J., Janssens, A. C. J. W., & Ransohoff, D. F. (2013). How can genomics improve population health?. American Journal of Preventive Medicine, 44(1 Suppl 1), S5–S14.
- Evans, J. P., Green, R. C., & McCabe, E. R. B. (2013). Predictive genetic testing–how good is good enough?. New England Journal of Medicine, 369(25), 2373–2375.
- National Human Genome Research Institute. (n.d.). Regulation of Genetic Tests. Retrieved from https://www.genome.gov/about-genomics/policy-issues/Regulation-Genetic-Tests
- The Genetic Information Nondiscrimination Act of 2008 (GINA). Public Law 110-233.
- ACMG Board of Directors. (2021). ACMG policy statement: updated recommendations for reporting of secondary findings in clinical exome and genome sequencing. Genetics in Medicine, 23(5), 814–824.
- Torkamani, A., Wineinger, N. E., & Topol, E. J. (2018). The personal and clinical utility of polygenic risk scores. Nature Reviews Genetics, 19(9), 581–590.
- Hogarth, S., Javitt, G., & Melzer, D. (2008). The current landscape for direct-to-consumer genetic testing: a systematic review. Genetics in Medicine, 10(10), 701–710.
- Phillips, K. A., Annas, G. J., & Elias, S. (2013). DNA banking for research and clinical care: policy guidance and recommendations. Genetics in Medicine, 15(1), 1–15.
- Caulfield, T., & Condit, C. M. (2012). Science and the sources of hype: why some areas of research generate more media enthusiasm than others. Public Understanding of Science, 21(3), 347-357.
The point about ethical considerations is critical, particularly concerning data privacy and informed consent. With the rise of DTC-GT, how do we ensure individuals fully understand the implications of sharing their genetic information and the potential for its use by third parties?