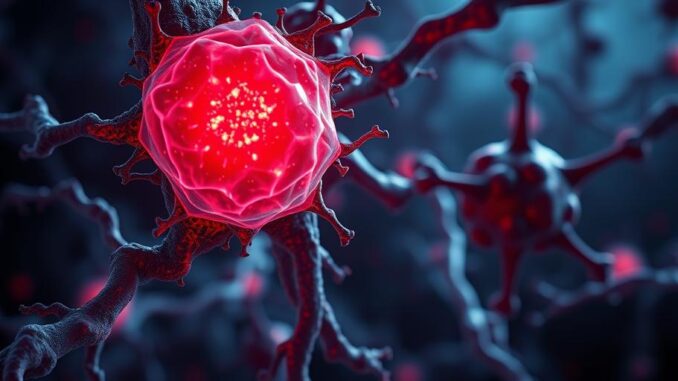
Abstract
Metastasis, the dissemination of cancer cells from a primary tumor to distant sites, remains the leading cause of cancer-related mortality. This intricate and multifaceted process involves a complex interplay of cellular and molecular events, including detachment from the primary tumor, invasion of surrounding tissues, intravasation into the circulatory system, survival in circulation, extravasation at distant sites, and colonization to form secondary tumors. This review delves into the molecular mechanisms governing each step of the metastatic cascade, examining the roles of epithelial-mesenchymal transition (EMT), cell adhesion molecules, proteases, and signaling pathways. We will also explore the crucial influence of the tumor microenvironment (TME) in modulating metastatic potential and therapeutic response. Furthermore, we discuss the diagnostic challenges associated with detecting early-stage metastasis and the prognostic implications of metastatic disease. Finally, we critically evaluate current therapeutic approaches targeting metastasis and highlight promising novel strategies, including immunotherapy, anti-angiogenic therapies, and agents targeting the TME, while also acknowledging the inherent challenges in translating preclinical findings into effective clinical outcomes.
Many thanks to our sponsor Esdebe who helped us prepare this research report.
1. Introduction
Metastasis, derived from the Greek word meaning “displacement,” is the process by which cancer cells spread from the primary tumor to establish secondary tumors in distant organs. While localized primary tumors can often be effectively treated with surgery, radiation, or chemotherapy, metastatic disease remains a formidable challenge in cancer management. Understanding the intricacies of metastasis is therefore paramount for improving patient outcomes and developing more effective therapies. The metastatic process is not a single event but a sequential series of steps, often referred to as the metastatic cascade, which involves a complex interplay between cancer cells and the surrounding microenvironment. Each step presents opportunities for therapeutic intervention, making a comprehensive understanding of the underlying mechanisms crucial.
Many thanks to our sponsor Esdebe who helped us prepare this research report.
2. The Metastatic Cascade: A Multi-Step Process
The metastatic cascade can be broadly divided into several distinct stages, each governed by specific molecular and cellular mechanisms.
2.1 Detachment and Local Invasion
The initial step in metastasis involves the detachment of cancer cells from the primary tumor mass. This process is often facilitated by the loss of cell-cell adhesion, particularly E-cadherin, a key component of adherens junctions. Downregulation of E-cadherin is frequently associated with the epithelial-mesenchymal transition (EMT), a cellular process in which epithelial cells lose their cell polarity and cell-cell adhesion and gain migratory and invasive properties. EMT is driven by transcription factors such as Snail, Slug, Twist, and Zeb1/2, which repress E-cadherin expression and induce the expression of mesenchymal markers like vimentin and N-cadherin. Notably, EMT is not an all-or-nothing phenomenon but rather a spectrum of states, with cells exhibiting varying degrees of epithelial and mesenchymal characteristics. This plasticity is crucial for cancer cell survival and adaptation during metastasis. Furthermore, dysregulation of tight junctions and desmosomes, other cell adhesion complexes, contributes to the loss of epithelial integrity.
Following detachment, cancer cells must invade the surrounding extracellular matrix (ECM) to gain access to blood or lymphatic vessels. This process involves the degradation of the ECM by secreted proteases, such as matrix metalloproteinases (MMPs), urokinase plasminogen activator (uPA), and cathepsins. MMPs, in particular, play a critical role in ECM remodeling by degrading collagen, laminin, and other ECM components. Cancer cells also utilize invadopodia, actin-rich protrusions that extend from the cell membrane and mediate ECM degradation at sites of cell-matrix contact. These invasive structures are crucial for breaching the basement membrane and invading adjacent tissues.
2.2 Intravasation and Survival in Circulation
Intravasation, the entry of cancer cells into blood or lymphatic vessels, is another critical step in metastasis. Cancer cells typically intravasate into pre-existing vessels or induce angiogenesis, the formation of new blood vessels, to facilitate their entry into the circulation. The tumor microenvironment plays a crucial role in promoting angiogenesis by secreting pro-angiogenic factors such as vascular endothelial growth factor (VEGF). VEGF binds to its receptor, VEGFR, on endothelial cells, stimulating their proliferation, migration, and tube formation. Cancer cells can also co-opt existing lymphatic vessels for lymphatic metastasis, a common route of dissemination for many solid tumors.
Once in the circulation, cancer cells face a hostile environment characterized by shear stress, immune surveillance, and lack of cell-matrix adhesion. To survive in circulation, cancer cells can form aggregates with platelets, immune cells, or other cancer cells, which protect them from mechanical stress and immune attack. These circulating tumor cell (CTC) clusters are thought to be more resistant to anoikis (apoptosis induced by loss of cell-matrix adhesion) and have a higher metastatic potential than single CTCs. Furthermore, cancer cells can express surface molecules that enable them to evade immune recognition and destruction. For example, some cancer cells express programmed death-ligand 1 (PD-L1), which binds to PD-1 on T cells and inhibits their cytotoxic activity.
2.3 Extravasation and Colonization
Extravasation, the exit of cancer cells from the circulation and into distant tissues, is a complex process that involves adhesion to the endothelium, transmigration through the endothelial barrier, and invasion of the target organ parenchyma. Cancer cells often adhere to the endothelium via interactions between cell adhesion molecules, such as selectins and integrins, and their respective ligands on endothelial cells. Selectins mediate initial tethering and rolling of cancer cells along the endothelium, while integrins mediate firm adhesion and transmigration. The specific adhesion molecules involved vary depending on the cancer type and the target organ. After adhering to the endothelium, cancer cells must cross the endothelial barrier to enter the surrounding tissue. This can occur via paracellular transmigration (migration between endothelial cells) or transcellular transmigration (migration through endothelial cells). In some cases, cancer cells can disrupt the endothelial barrier by secreting proteases or inducing endothelial cell retraction.
Colonization, the establishment of a secondary tumor at a distant site, is the final and often most inefficient step in the metastatic cascade. Many cancer cells that successfully extravasate into distant tissues fail to colonize and form macroscopic metastases. Colonization requires cancer cells to adapt to the new microenvironment, proliferate, and recruit stromal cells to support tumor growth. The pre-metastatic niche, a microenvironment prepared by the primary tumor to facilitate metastasis, plays a crucial role in colonization. Primary tumors can secrete factors that alter the microenvironment of distant organs, making them more receptive to metastatic cells. For example, primary tumors can secrete exosomes containing proteins and microRNAs that promote angiogenesis, immune suppression, and ECM remodeling in distant organs. Furthermore, the interactions between cancer cells and resident stromal cells, such as fibroblasts, immune cells, and endothelial cells, play a critical role in determining the fate of metastatic cells.
Many thanks to our sponsor Esdebe who helped us prepare this research report.
3. The Role of the Tumor Microenvironment in Metastasis
The tumor microenvironment (TME) is a complex and dynamic ecosystem surrounding cancer cells, consisting of various cellular and non-cellular components, including stromal cells (fibroblasts, immune cells, endothelial cells), blood vessels, lymphatic vessels, extracellular matrix (ECM), and soluble factors (growth factors, cytokines, chemokines). The TME plays a crucial role in modulating cancer cell behavior, including proliferation, survival, invasion, angiogenesis, and metastasis. It is now widely recognized that the TME is not merely a passive bystander but rather an active participant in cancer progression and metastasis.
3.1 Stromal Cells
Fibroblasts are one of the most abundant cell types in the TME and play a critical role in ECM remodeling and angiogenesis. Cancer-associated fibroblasts (CAFs) are activated fibroblasts that exhibit distinct characteristics compared to normal fibroblasts. CAFs secrete growth factors, cytokines, and ECM components that promote cancer cell proliferation, invasion, and angiogenesis. They also contribute to immune suppression by secreting factors that inhibit T cell activity. Immune cells, including macrophages, neutrophils, lymphocytes, and myeloid-derived suppressor cells (MDSCs), are also important components of the TME. The role of immune cells in cancer is complex and can be both tumor-promoting and tumor-suppressing. In some cases, immune cells can kill cancer cells and inhibit tumor growth. However, in other cases, immune cells can promote tumor growth and metastasis by secreting growth factors, cytokines, and proteases. Macrophages, in particular, can be polarized into different phenotypes, M1 and M2, with distinct functions. M1 macrophages are typically tumor-suppressing and promote inflammation, while M2 macrophages are typically tumor-promoting and suppress inflammation. MDSCs are a heterogeneous population of immature myeloid cells that suppress T cell activity and promote angiogenesis and metastasis. Endothelial cells form the lining of blood vessels and play a crucial role in angiogenesis. The TME contains pro-angiogenic factors, such as VEGF, that stimulate endothelial cell proliferation, migration, and tube formation, leading to the formation of new blood vessels that supply nutrients and oxygen to the tumor and facilitate metastasis.
3.2 Extracellular Matrix
The ECM is a complex network of proteins and polysaccharides that provides structural support to tissues and regulates cell behavior. The ECM in the TME is often altered compared to normal tissues, with increased deposition of collagen, fibronectin, and other ECM components. This altered ECM can promote cancer cell invasion and metastasis by providing a scaffold for cell migration and by activating signaling pathways that promote cell survival and proliferation. The ECM also contains growth factors and cytokines that can be released upon ECM degradation, further stimulating cancer cell growth and angiogenesis.
3.3 Soluble Factors
The TME is rich in soluble factors, including growth factors, cytokines, and chemokines, which play a crucial role in regulating cancer cell behavior and the interactions between cancer cells and stromal cells. Growth factors, such as epidermal growth factor (EGF), transforming growth factor-beta (TGF-β), and hepatocyte growth factor (HGF), stimulate cancer cell proliferation, survival, and migration. Cytokines, such as interleukin-6 (IL-6) and tumor necrosis factor-alpha (TNF-α), promote inflammation and angiogenesis. Chemokines, such as CXCL12 and CCL2, recruit immune cells and stromal cells to the TME. These soluble factors can act in a paracrine manner, affecting neighboring cells, or in an autocrine manner, affecting the cancer cells themselves. The specific combination of soluble factors present in the TME varies depending on the cancer type, the stage of the disease, and the host immune response.
Many thanks to our sponsor Esdebe who helped us prepare this research report.
4. Signaling Pathways Involved in Metastasis
Several signaling pathways are frequently dysregulated in cancer cells and contribute to the metastatic process. These pathways regulate various cellular processes, including cell proliferation, survival, invasion, angiogenesis, and immune evasion.
4.1 Receptor Tyrosine Kinase (RTK) Signaling
RTKs are transmembrane receptors that activate intracellular signaling cascades upon binding to their respective ligands. Several RTKs, including EGFR, HER2, MET, and VEGFR, are frequently overexpressed or activated in cancer cells and contribute to metastasis. EGFR signaling promotes cell proliferation, survival, and invasion. HER2 signaling is involved in cell proliferation and survival. MET signaling promotes cell invasion and angiogenesis. VEGFR signaling promotes angiogenesis. Inhibition of RTK signaling with specific inhibitors has shown some success in treating metastatic cancer, but resistance to these inhibitors is a common problem.
4.2 PI3K/AKT/mTOR Pathway
The PI3K/AKT/mTOR pathway is a central regulator of cell growth, survival, and metabolism. This pathway is frequently activated in cancer cells due to mutations or overexpression of PI3K, AKT, or mTOR. Activation of the PI3K/AKT/mTOR pathway promotes cell proliferation, survival, and angiogenesis. Inhibition of this pathway with specific inhibitors has shown promise in preclinical studies, but clinical trials have been less successful due to toxicity and resistance.
4.3 MAPK Pathway
The MAPK pathway is another key signaling pathway involved in cell proliferation, differentiation, and survival. This pathway is activated by various growth factors, cytokines, and stress stimuli. Activation of the MAPK pathway promotes cell proliferation, survival, and angiogenesis. Mutations in RAS and BRAF, key components of the MAPK pathway, are common in cancer cells and contribute to pathway activation. Inhibition of the MAPK pathway with specific inhibitors has shown some success in treating metastatic melanoma, but resistance to these inhibitors is also a problem.
4.4 TGF-β Signaling
The TGF-β signaling pathway plays a dual role in cancer, acting as a tumor suppressor in early stages and as a tumor promoter in later stages. In early stages, TGF-β inhibits cell proliferation and induces apoptosis. However, in later stages, TGF-β promotes cell invasion, angiogenesis, and immune suppression. TGF-β induces EMT by activating transcription factors such as Snail, Slug, Twist, and Zeb1/2. TGF-β also promotes angiogenesis by stimulating the production of VEGF. Inhibition of TGF-β signaling has shown promise in preclinical studies, but clinical trials have been less successful due to the complex role of TGF-β in cancer.
4.5 Wnt Signaling
The Wnt signaling pathway is involved in cell fate determination, proliferation, and differentiation. This pathway is frequently activated in cancer cells due to mutations in APC or β-catenin. Activation of the Wnt pathway promotes cell proliferation and survival. Inhibition of the Wnt pathway has shown promise in preclinical studies, but clinical trials are ongoing.
4.6 Hedgehog Signaling
The Hedgehog signaling pathway is involved in cell fate determination, proliferation, and differentiation. This pathway is frequently activated in cancer cells due to mutations in PTCH1 or SMO. Activation of the Hedgehog pathway promotes cell proliferation and survival. Inhibition of the Hedgehog pathway with specific inhibitors has shown some success in treating metastatic basal cell carcinoma, but resistance to these inhibitors is also a problem.
Many thanks to our sponsor Esdebe who helped us prepare this research report.
5. Diagnostic and Prognostic Implications of Metastasis
The detection and characterization of metastasis are crucial for cancer diagnosis, prognosis, and treatment planning. Several methods are used to detect metastasis, including imaging techniques, such as CT scans, MRI, PET scans, and bone scans, as well as biopsies and blood tests. However, detecting early-stage metastasis remains a challenge.
5.1 Imaging Techniques
CT scans and MRI are commonly used to detect macroscopic metastases in various organs. PET scans are more sensitive than CT scans and MRI for detecting small metastases. Bone scans are used to detect bone metastases. However, these imaging techniques have limitations in detecting micrometastases or circulating tumor cells.
5.2 Biopsies
Biopsies are used to confirm the presence of metastasis and to determine the histological type and molecular characteristics of the metastatic cells. Biopsies can be obtained from various sites, including lymph nodes, bone, liver, and lung. However, biopsies are invasive and can be associated with complications.
5.3 Liquid Biopsies
Liquid biopsies, which involve the analysis of blood or other bodily fluids, offer a non-invasive approach to detect and characterize metastasis. Liquid biopsies can be used to detect circulating tumor cells (CTCs), circulating tumor DNA (ctDNA), and exosomes. CTCs are cancer cells that have detached from the primary tumor and entered the circulation. ctDNA is DNA that has been released from cancer cells into the circulation. Exosomes are small vesicles that are secreted by cancer cells and contain proteins, RNA, and DNA. Liquid biopsies can provide valuable information about the molecular characteristics of metastatic cells and can be used to monitor treatment response and detect early signs of recurrence. However, the sensitivity of liquid biopsies is still limited, particularly for detecting early-stage metastasis.
The presence of metastasis is a major prognostic factor in cancer. Patients with metastatic disease have a significantly worse prognosis than patients with localized disease. The specific prognosis depends on the cancer type, the site of metastasis, and the extent of the disease. Several prognostic factors have been identified that can help predict the outcome of patients with metastatic disease. These factors include the number of metastatic sites, the size of the metastases, the presence of specific mutations, and the response to treatment.
Many thanks to our sponsor Esdebe who helped us prepare this research report.
6. Therapeutic Strategies Targeting Metastasis
Targeting metastasis is a major goal of cancer therapy. Several therapeutic strategies have been developed to prevent or inhibit metastasis, including surgery, radiation therapy, chemotherapy, targeted therapy, and immunotherapy.
6.1 Surgery and Radiation Therapy
Surgery can be used to remove localized metastases. Radiation therapy can be used to treat metastases in specific organs. However, surgery and radiation therapy are not effective for treating widespread metastasis.
6.2 Chemotherapy
Chemotherapy is a systemic treatment that uses cytotoxic drugs to kill cancer cells. Chemotherapy can be effective in treating metastatic cancer, but it is associated with significant side effects. Chemotherapy drugs target rapidly dividing cells, including cancer cells, but they also affect normal cells, leading to side effects such as nausea, vomiting, hair loss, and fatigue.
6.3 Targeted Therapy
Targeted therapy involves the use of drugs that specifically target molecular targets that are involved in cancer cell growth, survival, and metastasis. Several targeted therapies have been developed that have shown success in treating metastatic cancer. For example, EGFR inhibitors are used to treat metastatic non-small cell lung cancer with EGFR mutations. HER2 inhibitors are used to treat metastatic breast cancer with HER2 overexpression. BRAF inhibitors are used to treat metastatic melanoma with BRAF mutations. However, resistance to targeted therapies is a common problem.
6.4 Immunotherapy
Immunotherapy involves the use of drugs that stimulate the immune system to attack cancer cells. Several immunotherapies have been developed that have shown success in treating metastatic cancer. For example, immune checkpoint inhibitors, such as anti-PD-1 and anti-CTLA-4 antibodies, are used to treat metastatic melanoma, non-small cell lung cancer, and other cancers. These antibodies block the interaction between immune checkpoint proteins, such as PD-1 and CTLA-4, and their ligands, which allows T cells to kill cancer cells. Adoptive cell therapy, which involves the ex vivo expansion and activation of T cells, followed by infusion into the patient, has also shown success in treating metastatic cancer. However, immunotherapy is not effective for all patients, and it can be associated with immune-related adverse events.
6.5 Novel Therapeutic Strategies
Several novel therapeutic strategies are being developed to target metastasis, including anti-angiogenic therapies, matrix metalloproteinase inhibitors, and agents that target the tumor microenvironment. Anti-angiogenic therapies inhibit the formation of new blood vessels, which can prevent metastasis. Matrix metalloproteinase inhibitors inhibit the activity of matrix metalloproteinases, which can prevent cancer cell invasion. Agents that target the tumor microenvironment can disrupt the interactions between cancer cells and stromal cells, which can inhibit metastasis.
Many thanks to our sponsor Esdebe who helped us prepare this research report.
7. Challenges and Future Directions
Despite significant advances in our understanding of metastasis, several challenges remain in the prevention and treatment of metastatic disease. One major challenge is the heterogeneity of cancer cells and the tumor microenvironment. Cancer cells within a single tumor can exhibit significant genetic and epigenetic heterogeneity, which can lead to differences in their metastatic potential and response to therapy. The tumor microenvironment is also highly heterogeneous, with variations in the composition and organization of stromal cells, ECM, and soluble factors. This heterogeneity can affect cancer cell behavior and treatment response. Another challenge is the development of drug resistance. Cancer cells can develop resistance to chemotherapy, targeted therapy, and immunotherapy through various mechanisms, including mutations, epigenetic changes, and alterations in signaling pathways. Overcoming drug resistance is a major goal of cancer research.
Future research efforts should focus on developing more personalized approaches to cancer therapy that take into account the heterogeneity of cancer cells and the tumor microenvironment. This will require the development of new diagnostic tools that can accurately characterize the molecular profile of cancer cells and the TME. Furthermore, combination therapies that target multiple pathways and mechanisms may be more effective in preventing and treating metastasis. Finally, greater emphasis should be placed on developing strategies to prevent metastasis in the first place, such as early detection and intervention, and the development of vaccines that can prevent cancer.
Many thanks to our sponsor Esdebe who helped us prepare this research report.
8. Conclusion
Metastasis is a complex and multifaceted process that remains the leading cause of cancer-related mortality. Understanding the molecular mechanisms governing each step of the metastatic cascade, the role of the tumor microenvironment, and the signaling pathways involved is crucial for developing more effective therapies. While significant progress has been made in recent years, several challenges remain in the prevention and treatment of metastatic disease. Future research efforts should focus on developing more personalized approaches to cancer therapy that take into account the heterogeneity of cancer cells and the tumor microenvironment, as well as strategies to prevent metastasis in the first place.
Many thanks to our sponsor Esdebe who helped us prepare this research report.
References
- Hanahan, D., & Weinberg, R. A. (2011). Hallmarks of cancer: the next generation. Cell, 144(5), 646-674.
- Lambert, A. W., Pattabiraman, D. R., & Weinberg, R. A. (2017). EMT programs and cancer metastasis. Nature Reviews Clinical Oncology, 14(1), 8-29.
- Friedl, P., & Gilmour, D. (2009). Collective cell migration in morphogenesis, regeneration and cancer. Nature Reviews Molecular Cell Biology, 10(7), 445-457.
- Joyce, J. A., & Pollard, J. W. (2009). Microenvironmental regulation of metastasis. Nature Reviews Cancer, 9(4), 239-252.
- Quail, D. F., & Joyce, J. A. (2017). Microenvironmental regulation of tumor progression and metastasis. Nature Medicine, 23(1), 1-12.
- Fares, J., Fares, M. Y., Khachfe, H. H., Salhab, H. A., & Fares, Y. (2020). Molecular principles of metastasis. Signal Transduction and Targeted Therapy, 5(1), 28.
- Pantel, K., & Speicher, M. R. (2016). Cancer micrometastasis. Nature Reviews Cancer, 16(3), 121-134.
- Aktas, B., Kasimir-Bauer, S., Schwarzenbach, H., Ali, M., Speicher, M. R., & Pantel, K. (2009). Prognostic relevance of circulating tumor cells in metastatic breast cancer: a systematic review and meta-analysis. Breast Cancer Research, 11(5), R46.
- Wan, J. C. C., Massie, C., Garcia-Corbacho, J., Mouliere, F., Brenton, J. D., Caldas, C., … & Rosenfeld, N. (2017). Liquid biopsies come of age: towards implementation of circulating tumour DNA. Nature Reviews Cancer, 17(4), 223-238.
- Ribatti, D., Nico, B., & Crivellato, E. (2007). Angiogenesis and anti-angiogenesis: an historical overview. Leukemia, 21(1), 44-52.
- Coussens, L. M., Werb, Z. Inflammation and cancer. Nature 2002, 420, 860-867.
- Bissell, M. J., Hines, W. C. Why don’t we get more cancer? A proposed role of the microenvironment in restraining cancer progression. Nat. Med. 2011, 17, 320-329.
- Chiang, A. C., Massagué, J. Molecular basis of metastasis. N. Engl. J. Med. 2008, 359, 2814-2823.
- Van Meter, E. M., Kim, S. J., Weeraratna, A. T. The role of extracellular matrix in melanoma metastasis. Curr. Opin. Cell Biol. 2013, 25, 623-629.
- Yu, M., Bardia, A., Wittner, B. S., Stott, S. L., Smas, M. E., Ting, D. T., … Haber, D. A. Circulating breast cancer cells exhibit dynamic changes in epithelial and mesenchymal composition. Science 2013, 339, 580-584.
- Plaks, V., Koopman, C. D., Werb, Z. Cancer. Circulating tumor cells. Science 2013, 341, 1186-1188.
- Brabletz, S., Brabletz, T., Nieto, M. A. The EMT under attack: self-generated stress in cancer cells during epithelial-mesenchymal transition. Cancer Cell 2011, 19, 709-712.
- Giannelli, G., Koudelkova, P., Brezinova, J., Vetvicka, V. Matrix metalloproteinases (MMPs): important targets for cancer therapy? Biomed. Pap. Med. Fac. Univ. Palacky Olomouc Czech Repub. 2013, 157, 105-111.
- Fidler, I. J. The pathogenesis of cancer metastasis: the ‘seed and soil’ hypothesis revisited. Nat. Rev. Cancer 2003, 3, 453-458.
- Weinberg RA. The Biology of Cancer. 2nd ed. New York: Garland Science; 2014.
- Seyfried, T. N., Flores, R. E., Poff, A. M., & D’Agostino, D. P. (2014). Cancer as a metabolic disease: implications for novel therapeutics. Carcinogenesis, 36(1), 1-13.
Considering the role of the pre-metastatic niche, could interventions designed to alter the microenvironment of distant organs prove effective in preventing or delaying secondary tumor formation?
That’s a great question! Absolutely, targeting the pre-metastatic niche is showing real promise. Altering the microenvironment through interventions like immunomodulation or ECM remodeling could potentially disrupt the ‘soil’ and make it less receptive to arriving cancer cells. This could be a key strategy for preventing or delaying metastasis. What are your thoughts on the challenges of delivering these interventions specifically to the distant organs?
Editor: MedTechNews.Uk
Thank you to our Sponsor Esdebe
So, if cancer cells are playing musical chairs in the body, EMT is like their chaotic dance-off to new locations! Fascinating how these cells adapt and groove their way through the metastatic cascade. Wonder if offering them a less hospitable dance floor would change their tune?