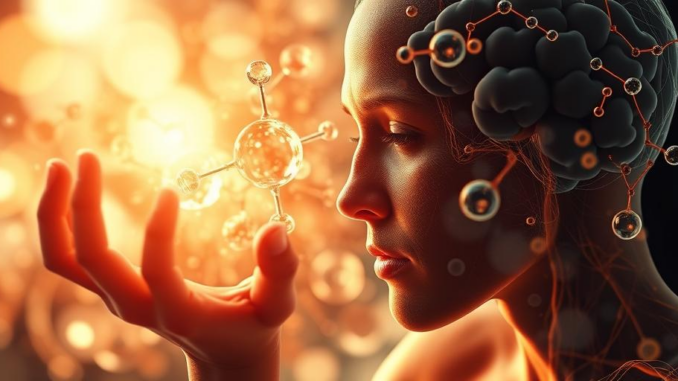
Abstract
Oxidative stress, characterized by an imbalance between the production of reactive oxygen and nitrogen species (RONS) and antioxidant defenses, has long been implicated in the pathogenesis of a wide array of neurological disorders, from Alzheimer’s disease (AD) and Parkinson’s disease (PD) to amyotrophic lateral sclerosis (ALS) and stroke. While the detrimental effects of excessive oxidative damage are well-documented, emerging evidence highlights the complex and often dual-edged nature of RONS, suggesting their involvement in crucial cellular signaling pathways and adaptive responses. This review aims to provide a comprehensive overview of the current understanding of oxidative stress in neurodegeneration, encompassing its underlying biological mechanisms, diagnostic approaches beyond traditional PET imaging, the potential of lifestyle and dietary interventions, and the latest advancements in antioxidant therapies. Furthermore, we will explore the challenges associated with modulating RONS for therapeutic benefit, considering the importance of maintaining redox homeostasis and the potential off-target effects of antioxidant interventions. Finally, we will present an opinion as to what we consider the most promising areas of research in this complex field of oxidative stress in neurodegeneration.
Many thanks to our sponsor Esdebe who helped us prepare this research report.
1. Introduction
The central nervous system (CNS), with its high metabolic rate, abundant lipid content, and relatively weak antioxidant defenses, is particularly vulnerable to oxidative stress. This vulnerability is further exacerbated by the presence of redox-active metals like iron and copper, which can catalyze the formation of highly reactive hydroxyl radicals via the Fenton and Haber-Weiss reactions [1]. Under physiological conditions, a delicate balance exists between the generation and scavenging of RONS, ensuring proper cellular function and signaling. However, in neurodegenerative diseases, this balance is disrupted, leading to a chronic state of oxidative stress that contributes to neuronal dysfunction and cell death [2]. The damaging effects of RONS include lipid peroxidation, protein oxidation, DNA damage, and mitochondrial dysfunction, all of which have been implicated in the pathogenesis of various neurological disorders [3].
While the detrimental effects of oxidative stress are widely recognized, it is becoming increasingly clear that RONS also play important roles in cell signaling, immune responses, and adaptive mechanisms [4]. For example, superoxide radicals can activate signaling pathways involved in neuronal survival and plasticity [5]. Moreover, low levels of RONS can induce the expression of antioxidant enzymes, thereby enhancing the cell’s ability to cope with oxidative stress [6]. Therefore, a nuanced understanding of the complex interplay between RONS and cellular function is crucial for developing effective therapeutic strategies that target oxidative stress in neurodegenerative diseases.
Many thanks to our sponsor Esdebe who helped us prepare this research report.
2. Biological Mechanisms of Oxidative Stress in Neurodegeneration
The mechanisms by which oxidative stress contributes to neurodegeneration are multifaceted and involve a complex interplay of cellular and molecular processes.
2.1 Mitochondrial Dysfunction
Mitochondria are the primary source of cellular energy and also a major site of RONS production. In neurodegenerative diseases, mitochondrial dysfunction is a common feature, leading to increased RONS generation and decreased ATP production [7]. This vicious cycle of oxidative stress and mitochondrial damage perpetuates neuronal dysfunction and cell death. Specifically, defects in the electron transport chain (ETC) can lead to the leakage of electrons, resulting in the formation of superoxide radicals [8]. Furthermore, oxidative damage to mitochondrial DNA (mtDNA) can impair mitochondrial function and further exacerbate RONS production [9].
2.2 Protein Aggregation
Oxidative stress can promote protein aggregation, a hallmark of many neurodegenerative diseases. RONS can modify proteins, leading to misfolding and aggregation [10]. These aggregates can impair cellular function and trigger inflammatory responses, further contributing to neuronal damage. For example, in AD, oxidative stress promotes the aggregation of amyloid-beta (Aβ) peptides and tau protein, leading to the formation of plaques and tangles, respectively [11]. Similarly, in PD, oxidative stress contributes to the aggregation of α-synuclein, resulting in the formation of Lewy bodies [12].
2.3 Lipid Peroxidation
The brain is rich in polyunsaturated fatty acids (PUFAs), which are particularly susceptible to lipid peroxidation. Lipid peroxidation is a chain reaction initiated by RONS that results in the formation of highly reactive aldehydes, such as malondialdehyde (MDA) and 4-hydroxynonenal (4-HNE) [13]. These aldehydes can modify proteins and DNA, leading to cellular dysfunction and cell death. Lipid peroxidation has been implicated in the pathogenesis of AD, PD, and ALS [14].
2.4 DNA Damage
RONS can directly damage DNA, leading to mutations, strand breaks, and base modifications. DNA damage can impair DNA replication and transcription, leading to cellular dysfunction and cell death [15]. Furthermore, DNA damage can activate DNA repair pathways, which can consume cellular energy and further contribute to oxidative stress. Oxidative DNA damage has been implicated in the pathogenesis of AD, PD, and ALS [16].
2.5 Neuroinflammation
Oxidative stress can trigger neuroinflammation, a chronic inflammatory response in the brain that contributes to neurodegeneration. RONS can activate microglia and astrocytes, the resident immune cells of the brain, leading to the release of pro-inflammatory cytokines and chemokines [17]. These inflammatory mediators can further exacerbate oxidative stress and neuronal damage. Neuroinflammation has been implicated in the pathogenesis of AD, PD, and ALS [18].
Many thanks to our sponsor Esdebe who helped us prepare this research report.
3. Diagnostic Methods Beyond PET Imaging
Positron emission tomography (PET) imaging using radiolabeled tracers that bind to oxidative stress markers has been used to detect oxidative stress in vivo. However, PET imaging has limitations, including limited spatial resolution, radiation exposure, and high cost. Therefore, there is a need for alternative diagnostic methods that can provide more detailed and accessible information about oxidative stress in neurodegenerative diseases.
3.1 Measurement of Oxidative Stress Biomarkers in Biological Fluids
Several oxidative stress biomarkers can be measured in biological fluids, such as blood, cerebrospinal fluid (CSF), and urine. These biomarkers include MDA, 4-HNE, 8-hydroxydeoxyguanosine (8-OHdG), and protein carbonyls [19]. Measurement of these biomarkers can provide an indication of the overall level of oxidative stress in the body. However, it is important to note that these biomarkers are not specific to the brain and may reflect oxidative stress in other tissues. Furthermore, the levels of these biomarkers can be influenced by various factors, such as age, diet, and lifestyle [20]. Despite these limitations, measurement of oxidative stress biomarkers in biological fluids can be a useful tool for monitoring oxidative stress in neurodegenerative diseases.
3.2 Detection of Oxidative Stress Markers in Postmortem Brain Tissue
Postmortem brain tissue can be used to detect oxidative stress markers at the cellular and molecular level. Immunohistochemistry (IHC) and immunofluorescence (IF) can be used to detect oxidized proteins, lipids, and DNA in specific brain regions [21]. Furthermore, mass spectrometry can be used to identify specific protein modifications caused by oxidative stress [22]. These techniques can provide valuable information about the spatial distribution and molecular mechanisms of oxidative stress in neurodegenerative diseases. However, it is important to note that postmortem brain tissue may be subject to artifactual changes, such as autolysis and fixation artifacts. Therefore, it is important to use appropriate controls and validation methods when analyzing postmortem brain tissue.
3.3 Novel Imaging Techniques
Several novel imaging techniques are being developed to detect oxidative stress in vivo. These techniques include magnetic resonance spectroscopy (MRS), which can be used to measure levels of glutathione (GSH), a major antioxidant in the brain [23]. Electron paramagnetic resonance (EPR) spectroscopy can detect free radicals directly [24]. These techniques have the potential to provide more detailed and specific information about oxidative stress in neurodegenerative diseases. However, these techniques are still under development and may not be widely available.
3.4 Omics Technologies
Genomics, transcriptomics, proteomics, and metabolomics are useful to understand the complex molecular interactions leading to neuronal damage and oxidative stress responses. These techniques enable us to identify molecular signatures associated with disease progression, potential therapeutic targets and discover the relationship between genetic risk factors and oxidative stress levels in neurodegenerative disorders [25].
Many thanks to our sponsor Esdebe who helped us prepare this research report.
4. Lifestyle and Dietary Interventions to Mitigate Oxidative Stress
Lifestyle and dietary interventions can play a significant role in mitigating oxidative stress and promoting brain health. Several studies have shown that regular exercise, a healthy diet, and stress management techniques can reduce oxidative stress and improve cognitive function [26].
4.1 Exercise
Regular exercise has been shown to increase antioxidant defenses and reduce oxidative stress in the brain. Exercise can stimulate the production of antioxidant enzymes, such as superoxide dismutase (SOD) and glutathione peroxidase (GPx) [27]. Furthermore, exercise can improve mitochondrial function and reduce RONS production. However, it is important to note that excessive exercise can also lead to increased oxidative stress. Therefore, it is important to find a balance between exercise and rest [28].
4.2 Diet
A healthy diet rich in fruits, vegetables, and whole grains can provide antioxidants that can neutralize RONS. Fruits and vegetables are rich in vitamins C and E, carotenoids, and polyphenols, which are all potent antioxidants [29]. Furthermore, a diet low in processed foods, saturated fats, and sugar can reduce inflammation and oxidative stress. The Mediterranean diet, which is rich in fruits, vegetables, whole grains, olive oil, and fish, has been shown to be beneficial for brain health [30]. Specific dietary components, such as omega-3 fatty acids and curcumin, have also been shown to have antioxidant and anti-inflammatory properties [31].
4.3 Stress Management
Chronic stress can lead to increased oxidative stress in the brain. Stress hormones, such as cortisol, can stimulate RONS production and impair antioxidant defenses [32]. Therefore, stress management techniques, such as meditation, yoga, and deep breathing exercises, can help to reduce oxidative stress and promote brain health [33].
Many thanks to our sponsor Esdebe who helped us prepare this research report.
5. Antioxidant Therapies: Latest Research and Challenges
Antioxidant therapies have been investigated as a potential treatment strategy for neurodegenerative diseases. However, the results of clinical trials have been mixed, and there are several challenges associated with antioxidant therapies [34].
5.1 Types of Antioxidant Therapies
Several types of antioxidant therapies have been investigated, including:
- Vitamin E: A fat-soluble antioxidant that protects against lipid peroxidation [35].
- Vitamin C: A water-soluble antioxidant that scavenges free radicals [36].
- N-acetylcysteine (NAC): A precursor to glutathione that enhances antioxidant defenses [37].
- Coenzyme Q10 (CoQ10): An electron carrier in the ETC that also acts as an antioxidant [38].
- Resveratrol: A polyphenol found in grapes and red wine that has antioxidant and anti-inflammatory properties [39].
- Mitochondria-targeted antioxidants: Antioxidants that are specifically targeted to mitochondria to reduce oxidative stress in this organelle [40].
5.2 Challenges of Antioxidant Therapies
Several challenges are associated with antioxidant therapies, including:
- Bioavailability: Many antioxidants have poor bioavailability, meaning that they are not effectively absorbed into the body and do not reach the brain in sufficient concentrations [41].
- Blood-brain barrier (BBB): The BBB restricts the passage of many antioxidants into the brain [42].
- Dosage: The optimal dosage of antioxidants is not well-established. High doses of some antioxidants can have pro-oxidant effects [43].
- Specificity: Many antioxidants are not specific for the brain and may have off-target effects in other tissues [44].
- Timing: The timing of antioxidant therapy may be critical. Antioxidants may be more effective if administered early in the disease process, before significant neuronal damage has occurred [45].
5.3 The Dual-Edged Nature of RONS
As mentioned earlier, RONS can act as signaling molecules and play a role in adaptive responses. Therefore, complete elimination of RONS may not be beneficial and could even be harmful. Indeed, some studies have suggested that antioxidant therapies can interfere with cellular signaling pathways and impair immune function [46]. Therefore, it is important to modulate RONS levels in a controlled manner, rather than completely eliminating them.
5.4 Novel Antioxidant Strategies
Several novel antioxidant strategies are being developed to overcome the limitations of traditional antioxidant therapies. These strategies include:
- Nanoparticle-based delivery: Nanoparticles can be used to deliver antioxidants directly to the brain, bypassing the BBB [47].
- Enzyme-based antioxidants: Enzymes, such as SOD and catalase, can be used to scavenge RONS in a more efficient and specific manner [48].
- Redox modulators: Redox modulators are compounds that can shift the redox balance in cells, either by increasing or decreasing RONS levels [49].
- Gene therapy: Gene therapy can be used to increase the expression of antioxidant enzymes in the brain [50].
Many thanks to our sponsor Esdebe who helped us prepare this research report.
6. Conclusion and Future Directions
Oxidative stress plays a complex and multifaceted role in neurodegeneration. While the detrimental effects of excessive oxidative damage are well-documented, it is becoming increasingly clear that RONS also play important roles in cellular signaling, immune responses, and adaptive mechanisms. Therefore, a nuanced understanding of the complex interplay between RONS and cellular function is crucial for developing effective therapeutic strategies that target oxidative stress in neurodegenerative diseases.
Future research should focus on:
- Developing more sensitive and specific diagnostic methods for detecting oxidative stress in vivo.
- Investigating the mechanisms by which lifestyle and dietary interventions mitigate oxidative stress and promote brain health.
- Developing novel antioxidant therapies that can overcome the limitations of traditional antioxidants.
- Identifying the optimal timing and dosage of antioxidant therapies.
- Better defining the dual-edged nature of RONS and developing strategies for modulating them in a controlled manner.
- Investigating how cellular responses to oxidative stress differ with age and genetic background.
Specifically, in the opinion of this review, the most promising area for future research lies in the development of redox modulators. These compounds offer the potential to fine-tune RONS levels, rather than simply eliminating them. By carefully modulating the redox balance in cells, it may be possible to harness the beneficial effects of RONS while minimizing their damaging effects. Another area of promise is the use of targeted antioxidant delivery systems, such as nanoparticles, which can overcome the limitations of the BBB and deliver antioxidants directly to the brain. Combining these strategies with a deeper understanding of individual genetic risk factors may lead to more effective and personalized therapies for neurodegenerative diseases. Furthermore, there should be more studies regarding the gut-brain axis and how gut microbiota-related oxidative stress impacts neurodegeneration. The interplay between gut dysbiosis, inflammation and the release of RONS is an interesting area for future studies.
Many thanks to our sponsor Esdebe who helped us prepare this research report.
References
[1] Halliwell B, Gutteridge JM. Free radicals in biology and medicine. Oxford university press; 2015.
[2] Uttara B, Singh AV, Zamboni P, Mahajan RT. Oxidative stress and neurodegenerative diseases: a review. Immun Ageing. 2009;6:5.
[3] Butterfield DA, Di Domenico F, Barone E. Elevated risk of Alzheimer’s disease: the central role of increased oxidative stress. Dev Neurosci. 2014;36(6):417-28.
[4] Mittler R. ROS are good. Trends Plant Sci. 2017;22(1):11-19.
[5] Hurd TR, Murphy MP, Skehel PA. Production, compartmentalization and function of superoxide in neurons. Trends Neurosci. 2022;45(8):588-601.
[6] Lushchak VI. Adaptive response to oxidative stress: bacteria, fungi, plants and animals. Comp Biochem Physiol C Toxicol Pharmacol. 2011;153(2):175-90.
[7] Swerdlow RH. Mitochondria and mitochondrial dysfunction in Alzheimer’s disease. Exp Neurol. 2018;300:160-72.
[8] Brand MD. The contribution of mitochondrial proton leak to metabolic rate. Am J Clin Nutr. 2000;71(2):565-9.
[9] Chaturvedi RK, Flint Beal M. Mitochondrial dysfunction in neurodegenerative diseases. J Neurochem. 2013;126 Suppl 1:71-81.
[10] Dalle-Donne I, Rossi R, Colombo R, Giustarini D, Milzani A. Biomarkers of oxidative damage in human disease. Clin Chem. 2006;52(4):601-23.
[11] Butterfield DA, Swomley WR, Sultana R. Amyloid β-peptide(1-42)-induced oxidative stress in Alzheimer disease: importance in disease pathogenesis and promise in therapeutic intervention. Antioxid Redox Signal. 2013;19(8):823-35.
[12] Vila M, Przedborski S. Targeting oxidative stress in neurodegenerative diseases. Nat Rev Neurosci. 2003;4(5):365-76.
[13] Ayala A, Muñoz MF, Argüelles S. Lipid peroxidation: production, metabolism, and signaling mechanisms of malondialdehyde and 4-hydroxy-2-nonenal. Oxid Med Cell Longev. 2014;2014:360438.
[14] Barnham KJ, Masters CL, Bush AI. Neurodegenerative diseases and oxidative stress. Nat Rev Drug Discov. 2004;3(3):205-14.
[15] Cadet J, Douki T, Gasparutto D, Ravanat JL. Oxidative damage to DNA: formation, measurement and biochemical features. Mutat Res. 2003;531(1-2):5-23.
[16] Weissman L, Milkovic L, Gilgun-Sherki Y, Offen D. DNA damage and repair in neurodegenerative diseases. CNS Neurosci Ther. 2012;18(9):739-44.
[17] Glass CK, Saijo K, Winner B, Marchetto MC, Gage FH. Mechanisms underlying inflammation in neurodegeneration. Cell. 2010;140(6):918-34.
[18] Amor S, Peferoen L, Vogel DY, Breur M, van der Valk P, Baker D, et al. The immune response in different stages of multiple sclerosis: a simple model. Mult Scler. 2010;16(11):1284-91.
[19] Lyras M, Cairns BJ, Jenner A, Jenner P, Halliwell B. An assessment of the direct detection of oxidative damage to nucleic acids, lipids and proteins in brain tissue from patients with Parkinson’s disease. J Neurochem. 1997;68(5):2074-82.
[20] Ghezzi P, Bonetto V, Iannone A, Antico A, Paglia G, Conte C, et al. Oxidative stress in human diseases: from a comprehensive review to a practical paradigm shift in personalized medicine. Redox Biol. 2023;64:102783.
[21] Castellani RJ, Smith MA, Richey PL, Perry G. Oxidative stress and its role in Alzheimer disease. Am J Alzheimers Dis Other Demen. 2001;16(4):215-22.
[22] Butterfield DA, Lange ML. Mass spectrometry-based proteomics to identify brain proteins selectively modified by free radical-induced oxidative stress in neurodegenerative diseases: Alzheimer’s disease and amyotrophic lateral sclerosis. Mass Spectrom Rev. 2009;28(4):645-67.
[23] Mandal PK, Tripathi M, Sugunan S, Vairamani M, Bhatia R, Sharma R, et al. Brain glutathione levels in multiple system atrophy: a proton magnetic resonance spectroscopic study at 3 Tesla. J Neurol Sci. 2015;348(1-2):239-45.
[24] Kuppusamy P, Li H, Halpern HJ. In vivo electron paramagnetic resonance imaging of tissue redox status and metabolism. Antioxid Redox Signal. 2013;18(17):2364-76.
[25] Lunnon K, Keohane A, Pidsley R, Newhouse S, Shine J, Griffin J, et al. Integrative methylomics of late-onset Alzheimer’s disease. PLoS Genet. 2014;10(10):e1004726.
[26] Morris MC. Nutritional interventions for the prevention of Alzheimer’s disease. Ann N Y Acad Sci. 2016;1367(1):25-34.
[27] Radak Z, Chung HY, Goto S. Exercise and hormesis: oxidative stress-related adaptation for successful aging. Biogerontology. 2008;9(1):71-6.
[28] Powers SK, Jackson MJ. Exercise-induced oxidative stress: cellular mechanisms and impact on aging. Physiol Rev. 2008;88(4):1243-76.
[29] Lobo V, Patil A, Phatak A, Chandra N. Free radicals, antioxidants and functional foods: Impact on human health. Pharmacogn Rev. 2010;4(8):118-26.
[30] Davis A. The Mediterranean diet and Alzheimer’s disease. Am J Alzheimers Dis Other Demen. 2013;28(5):469-78.
[31] Calabrese V, Bates TE, Stella AM. Curcumin and the cellular stress response: implications for neurodegenerative diseases. Mol Neurobiol. 2008;37(1):44-63.
[32] Sapolsky RM. Stress, the aging brain, and the mechanisms of neuron death. MIT Press; 1992.
[33] McEwen BS. Protection and damage from acute and chronic stress: allostasis and allostatic overload and relevance to the pathophysiology of psychiatric disorders. Ann N Y Acad Sci. 2004;1032:1-7.
[34] Sano M, Ernesto C, Thomas RG, Klauber MR, Schafer K, Grundman M, et al. A controlled trial of selegiline, alpha-tocopherol, or both as treatment for Alzheimer’s disease. The Alzheimer’s Disease Cooperative Study. N Engl J Med. 1997;336(17):1216-22.
[35] Lloret A, Badia MC, Mora NJ, Cervera-Ferri A, Olivas S, Monllor P, et al. Vitamin E: a master antioxidant in neurodegenerative diseases. Front Neurosci. 2019;13:203.
[36] Harrison FE. A critical review of vitamin C for the prevention of age-related cognitive decline and Alzheimer’s disease. J Alzheimers Dis. 2012;29(4):711-26.
[37] Mokhtari V, Afshari P, Shahhoseini M, Kalantar SM, Moini A. A review on uses of N-acetylcysteine in neurological disorders. Cell Mol Neurobiol. 2019;39(4):487-508.
[38] Littarru GP, Tiano L. Bioenergetic and antioxidant properties of coenzyme Q10: recent developments. Mol Biotechnol. 2007;37(1):31-7.
[39] Baur JA, Sinclair DA. Therapeutic potential of resveratrol: the in vivo evidence. Nat Rev Drug Discov. 2006;5(6):493-506.
[40] Smith RA, Murphy MP. Selective targeting of mitochondria with antioxidants and their therapeutic potential. Ann N Y Acad Sci. 2010;1201:75-9.
[41] Daugherty A, Rateri DL. Concerns with antioxidant trials. Atherosclerosis. 2015;243(2):521-4.
[42] Abbott NJ, Patabendige AA, Dolman DE, Yusof SR, Begley DJ. Structure and function of the blood-brain barrier. Neurobiol Dis. 2010;37(1):13-25.
[43] Halliwell B. Are polyphenols good for us or bad? Clearing up the contradictions. Arch Biochem Biophys. 2008;476(2):107-12.
[44] Bast A, Haenen GR, Doelman CJ. Oxidants and antioxidants: state of the art. Am J Med. 1991;91(3C):2S-13S.
[45] Christen Y. Oxidative stress and Alzheimer’s disease. Am J Alzheimers Dis Other Demen. 2000;15(3):157-75.
[46] Rajasekaran NS, Venkatesan R, Song IS, Kim SY, Choi DY, Lee J, et al. Reactive oxygen species in apoptosis resistance. Antioxid Redox Signal. 2013;18(8):979-99.
[47] Allen TM, Cullis PR. Liposomal drug delivery systems: from concept to clinical applications. Adv Drug Deliv Rev. 2013;65(1):36-48.
[48] Crapo JD, Oury TD, Rabouille A, Slot JW. Copper-zinc superoxide dismutase is primarily a cytosolic protein in human cells. Proc Natl Acad Sci U S A. 1992;89(21):10405-9.
[49] Forman HJ, Maiorino M, Ursini F. Signalling functions of reactive oxygen species. Biochemistry. 2010;49(25):529-41.
[50] Manfredsson FP, Mandel RJ. Gene therapy approaches for Parkinson’s disease. Mov Disord. 2010;25 Suppl 1:S116-24.
Redox modulators, you say? Sounds promising, but I’m bracing myself for the inevitable headlines: “Miracle pill reverses aging… in yeast!” Still, if it keeps me from misplacing my keys (or my mind), I’m tentatively on board. Maybe hold the press releases until after human trials? Just a thought.
I appreciate your cautious optimism! You’re right, the path from promising research to proven treatment is a long one. Definitely agree that human trials are crucial before any talk of “miracle cures.” Focusing on robust, well-designed studies is key to advancing the science. Thanks for your comment!
Editor: MedTechNews.Uk
Thank you to our Sponsor Esdebe