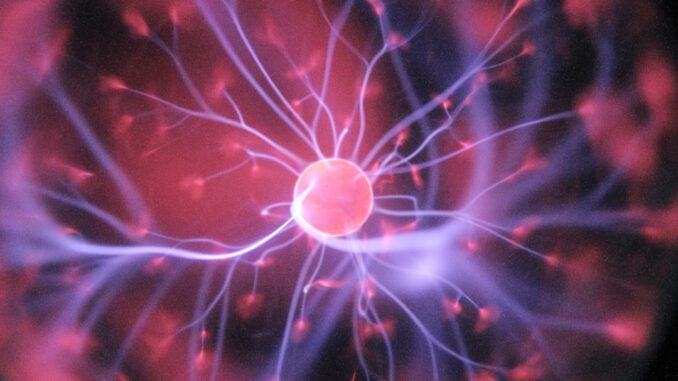
Abstract
Neurodegenerative diseases, encompassing conditions such as Alzheimer’s disease (AD), Parkinson’s disease (PD), Huntington’s disease (HD), and amyotrophic lateral sclerosis (ALS), pose a significant and growing global health challenge. These disorders, characterized by the progressive dysfunction and death of neurons, lead to debilitating cognitive and motor impairments, ultimately impacting quality of life and lifespan. Despite decades of research, effective disease-modifying therapies remain elusive. This report provides a comprehensive overview of neurodegenerative diseases, delving into their underlying molecular mechanisms, genetic and environmental risk factors, and current diagnostic and therapeutic strategies. We critically evaluate the limitations of traditional research approaches and highlight the transformative potential of advanced in vitro models, including three-dimensional (3D) brain organoids (mini-brains), for recapitulating disease-relevant phenotypes and accelerating therapeutic discovery. Furthermore, we explore emerging therapeutic avenues, such as gene therapy, immunotherapy, and small molecule drug development, with a particular emphasis on personalized medicine approaches tailored to individual patient profiles. Finally, we discuss the ethical considerations surrounding the use of advanced in vitro models and the translation of research findings to clinical practice.
Many thanks to our sponsor Esdebe who helped us prepare this research report.
1. Introduction
Neurodegenerative diseases are a heterogeneous group of disorders characterized by the progressive loss of neuronal structure and function, leading to irreversible neurological deficits. The prevalence of these diseases is increasing dramatically with the aging global population, placing a substantial burden on healthcare systems and society as a whole. The etiology of neurodegenerative diseases is complex and multifaceted, involving a complex interplay of genetic predisposition, environmental factors, and aging-related cellular processes. While significant progress has been made in identifying disease-associated genes and pathological hallmarks, the precise mechanisms underlying neuronal dysfunction and death remain incompletely understood.
Traditional research approaches, including in vitro cell culture models and in vivo animal models, have provided valuable insights into neurodegenerative disease pathogenesis. However, these models often fail to fully recapitulate the complexity of the human brain and the chronic nature of neurodegeneration. Two-dimensional (2D) cell cultures lack the intricate cellular organization, cell-cell interactions, and extracellular matrix (ECM) components present in the brain. Animal models, while providing a more complex in vivo environment, may exhibit species-specific differences in disease mechanisms and drug responses, limiting their translational relevance. The advent of advanced in vitro models, such as 3D brain organoids, offers a promising alternative for studying neurodegenerative diseases in a more physiologically relevant context.
Many thanks to our sponsor Esdebe who helped us prepare this research report.
2. Types of Neurodegenerative Diseases and Their Pathological Hallmarks
Neurodegenerative diseases are classified based on their clinical presentation, affected brain regions, and underlying pathological hallmarks. Several of the most prevalent and well-studied neurodegenerative diseases are summarized below:
-
Alzheimer’s Disease (AD): AD is the most common cause of dementia, characterized by progressive memory loss, cognitive decline, and behavioral changes. The hallmark pathologies of AD include extracellular amyloid plaques composed of amyloid-beta (Aβ) peptides and intracellular neurofibrillary tangles composed of hyperphosphorylated tau protein. The “amyloid cascade hypothesis” proposes that Aβ accumulation triggers a cascade of events leading to tau pathology, neuronal dysfunction, and ultimately, neuronal death. [1] However, this hypothesis is constantly being refined as evidence suggests the intricate connection between Aβ and tau is much more complex. Inflammation and vascular dysfunction also play important roles.
-
Parkinson’s Disease (PD): PD is a movement disorder characterized by tremor, rigidity, bradykinesia (slowness of movement), and postural instability. The primary pathological hallmark of PD is the loss of dopaminergic neurons in the substantia nigra pars compacta, a brain region involved in motor control. Intracellular inclusions called Lewy bodies, composed of aggregated α-synuclein protein, are also characteristic of PD. [2] The causes of PD are complex, involving mitochondrial dysfunction, oxidative stress, and protein aggregation. The presence of non-motor symptoms, such as sleep disorders and olfactory dysfunction, suggest the spread of the disease from the gut to the brain.
-
Huntington’s Disease (HD): HD is a genetic disorder caused by an expansion of a CAG repeat in the huntingtin (HTT) gene, resulting in a mutant HTT protein with an elongated polyglutamine tract. The mutant HTT protein aggregates and disrupts neuronal function, leading to progressive motor, cognitive, and psychiatric symptoms. [3] HD primarily affects the striatum and cortex, brain regions involved in motor control and cognitive function. The disease is autosomal dominant and symptoms typically manifest in mid-life, causing progressive motor, cognitive, and psychiatric disturbances.
-
Amyotrophic Lateral Sclerosis (ALS): ALS is a progressive neurodegenerative disease that affects motor neurons in the brain and spinal cord, leading to muscle weakness, paralysis, and ultimately, respiratory failure. [4] The etiology of ALS is heterogeneous, with both genetic and environmental factors contributing to disease risk. Mutations in genes such as SOD1, TARDBP, and FUS have been implicated in familial ALS. Pathological hallmarks of ALS include cytoplasmic aggregates of TDP-43 protein and motor neuron degeneration. Non-cell autonomous mechanisms, involving glial cells, are also thought to play a role.
-
Frontotemporal Lobar Degeneration (FTLD): FTLD is a group of neurodegenerative disorders characterized by progressive changes in behavior, personality, and language due to the degeneration of the frontal and temporal lobes of the brain. [5] The underlying pathology of FTLD is diverse, with the most common subtypes being FTLD-tau (characterized by tau protein aggregates) and FTLD-TDP (characterized by TDP-43 protein aggregates). Mutations in genes such as MAPT, GRN, and C9orf72 have been linked to FTLD.
Many thanks to our sponsor Esdebe who helped us prepare this research report.
3. Molecular Mechanisms Underlying Neurodegeneration
The molecular mechanisms underlying neurodegeneration are complex and vary depending on the specific disease. However, several common pathways and processes are implicated in the pathogenesis of multiple neurodegenerative diseases:
-
Protein Misfolding and Aggregation: The accumulation of misfolded and aggregated proteins is a common hallmark of many neurodegenerative diseases. Misfolded proteins can form toxic oligomers and insoluble aggregates, disrupting cellular function and triggering neuronal death. The ubiquitin-proteasome system (UPS) and autophagy are cellular mechanisms responsible for clearing misfolded proteins. Impairments in these pathways can contribute to protein aggregation and neurodegeneration. The prion-like spread of misfolded proteins between cells may also contribute to disease progression.
-
Mitochondrial Dysfunction: Mitochondria are essential organelles responsible for cellular energy production. Mitochondrial dysfunction, including impaired oxidative phosphorylation, increased oxidative stress, and altered mitochondrial dynamics, is implicated in the pathogenesis of many neurodegenerative diseases. Damaged mitochondria can release pro-apoptotic factors, leading to neuronal death. The removal of damaged mitochondria by mitophagy is critical for maintaining cellular health. Impairments in mitophagy can contribute to mitochondrial dysfunction and neurodegeneration.
-
Oxidative Stress: Oxidative stress, an imbalance between the production of reactive oxygen species (ROS) and antioxidant defense mechanisms, can damage cellular components, including DNA, proteins, and lipids. Increased oxidative stress is implicated in the pathogenesis of many neurodegenerative diseases. Antioxidant enzymes, such as superoxide dismutase (SOD) and catalase, play a critical role in neutralizing ROS. Impairments in antioxidant defense mechanisms can contribute to oxidative stress and neurodegeneration.
-
Inflammation: Chronic inflammation is increasingly recognized as a key contributor to neurodegeneration. Activated microglia and astrocytes release inflammatory cytokines and chemokines, which can exacerbate neuronal damage and dysfunction. The inflammasome, a multi-protein complex involved in the activation of inflammatory responses, is implicated in the pathogenesis of several neurodegenerative diseases. Resolving inflammation is essential for promoting neuronal survival and tissue repair. While inflammation is largely seen as detrimental, in the early stages, it may actually be neuroprotective.
-
Excitotoxicity: Excitotoxicity, the excessive stimulation of glutamate receptors, can lead to neuronal damage and death. Overactivation of glutamate receptors, particularly NMDA receptors, can cause an influx of calcium ions into neurons, triggering a cascade of events leading to apoptosis or necrosis. Excitotoxicity is implicated in the pathogenesis of several neurodegenerative diseases, including AD, PD, and ALS.
Many thanks to our sponsor Esdebe who helped us prepare this research report.
4. Current Diagnostic and Therapeutic Strategies
Currently, there are no curative treatments for most neurodegenerative diseases. Available therapies primarily focus on managing symptoms and improving quality of life.
-
Alzheimer’s Disease: Cholinesterase inhibitors (e.g., donepezil, rivastigmine, galantamine) and memantine are commonly used to treat cognitive symptoms in AD. These medications can provide temporary symptomatic relief but do not halt or reverse disease progression. Aducanumab, an anti-amyloid antibody, was recently approved by the FDA, but its clinical efficacy and potential side effects remain controversial. [6] Other Aβ-targeting therapies and tau-targeting therapies are under development. Lifestyle modifications, such as regular exercise, a healthy diet, and cognitive stimulation, may also help to slow cognitive decline.
-
Parkinson’s Disease: Levodopa, a precursor to dopamine, is the most effective medication for treating motor symptoms in PD. However, long-term levodopa use can lead to motor complications, such as dyskinesias. Dopamine agonists (e.g., pramipexole, ropinirole) can also be used to treat motor symptoms. Deep brain stimulation (DBS) is a surgical procedure that can alleviate motor symptoms in patients with advanced PD. Gene therapy approaches are also being explored.
-
Huntington’s Disease: Tetrabenazine and deutetrabenazine are used to treat chorea (involuntary movements) in HD. There are no disease-modifying therapies for HD. Supportive care, including physical therapy, occupational therapy, and speech therapy, can help to manage symptoms and improve quality of life. Several huntingtin-lowering therapies are in clinical development.
-
Amyotrophic Lateral Sclerosis: Riluzole and edaravone are the only FDA-approved medications that have been shown to modestly slow disease progression in ALS. Supportive care, including respiratory support, nutritional support, and physical therapy, is essential for managing symptoms and improving quality of life.
-
Frontotemporal Lobar Degeneration: There are no FDA-approved medications specifically for FTLD. Selective serotonin reuptake inhibitors (SSRIs) may be used to treat behavioral symptoms. Supportive care is essential for managing symptoms and improving quality of life.
Early diagnosis is crucial for maximizing the effectiveness of available therapies and providing patients with the opportunity to participate in clinical trials. Biomarkers, such as Aβ and tau levels in cerebrospinal fluid (CSF) and amyloid PET imaging, are used to diagnose AD in its early stages. DaTscan imaging is used to diagnose PD. Genetic testing is used to diagnose HD and certain forms of FTLD and ALS. However, more sensitive and specific biomarkers are needed for earlier and more accurate diagnosis of neurodegenerative diseases.
Many thanks to our sponsor Esdebe who helped us prepare this research report.
5. The Role of Mini-Brains in Neurodegeneration Research
Three-dimensional (3D) brain organoids, or “mini-brains,” are in vitro models that recapitulate many aspects of human brain development and structure. Brain organoids are typically generated from human pluripotent stem cells (hPSCs), which can be differentiated into various neural cell types, including neurons, astrocytes, and oligodendrocytes. These cells self-organize into complex 3D structures that resemble the developing brain. [7] Brain organoids offer several advantages over traditional 2D cell culture models and animal models for studying neurodegenerative diseases.
-
Improved Physiological Relevance: Brain organoids provide a more physiologically relevant environment for studying neurodegenerative diseases compared to 2D cell cultures. They contain multiple cell types, cell-cell interactions, and ECM components that are absent in 2D cultures. Brain organoids also exhibit more complex gene expression patterns and signaling pathways that are similar to those observed in the human brain.
-
Human-Specific Disease Modeling: Brain organoids can be generated from hPSCs derived from patients with neurodegenerative diseases, allowing for the study of human-specific disease mechanisms. Patient-derived brain organoids can recapitulate disease-relevant phenotypes, such as Aβ plaques, tau tangles, α-synuclein aggregates, and TDP-43 aggregates. This allows researchers to investigate the effects of genetic mutations and environmental factors on disease pathogenesis in a human context.
-
Drug Discovery and Screening: Brain organoids can be used as a platform for drug discovery and screening. They can be used to test the efficacy and toxicity of potential therapeutic compounds in a human-relevant model. Brain organoids can also be used to identify novel drug targets and develop personalized medicine approaches.
-
Understanding Disease Mechanisms: Brain organoids can be used to investigate the molecular mechanisms underlying neurodegenerative diseases. They can be used to study the effects of disease-associated proteins on neuronal function, synaptic transmission, and cell survival. Brain organoids can also be used to investigate the role of inflammation, oxidative stress, and mitochondrial dysfunction in neurodegeneration.
However, brain organoids also have some limitations. They lack a fully developed vascular system, which can limit nutrient and oxygen supply to the core of the organoid. Brain organoids also lack the complexity of the adult human brain, including the presence of specialized brain regions and long-range neuronal connections. In addition, the reproducibility and standardization of brain organoid protocols can be challenging. Despite these limitations, brain organoids are a valuable tool for studying neurodegenerative diseases and accelerating therapeutic discovery. There is a growing need for greater standardization of organoid protocols and reporting of results to enable easier comparison between studies.
Many thanks to our sponsor Esdebe who helped us prepare this research report.
6. Emerging Therapeutic Avenues
Several emerging therapeutic avenues hold promise for treating neurodegenerative diseases:
-
Gene Therapy: Gene therapy involves delivering therapeutic genes into cells to correct genetic defects or enhance cellular function. Gene therapy approaches are being developed to treat several neurodegenerative diseases, including PD, HD, and ALS. For example, gene therapy can be used to deliver genes encoding dopamine-synthesizing enzymes into the brains of patients with PD to restore dopamine levels. Gene therapy can also be used to deliver huntingtin-lowering genes into the brains of patients with HD to reduce the levels of mutant HTT protein.
-
Immunotherapy: Immunotherapy involves harnessing the power of the immune system to fight disease. Immunotherapy approaches are being developed to treat AD and other neurodegenerative diseases. For example, anti-Aβ antibodies can be used to clear Aβ plaques from the brain in patients with AD. Immunotherapy can also be used to modulate the inflammatory response in the brain.
-
Small Molecule Drug Development: Small molecule drugs are chemical compounds that can be designed to target specific proteins or pathways involved in neurodegeneration. Small molecule drugs are being developed to inhibit protein aggregation, reduce oxidative stress, improve mitochondrial function, and modulate inflammation.
-
Personalized Medicine: Personalized medicine involves tailoring treatment to the individual patient based on their genetic profile, disease stage, and other factors. Personalized medicine approaches are being developed to treat neurodegenerative diseases. For example, patients with specific genetic mutations may benefit from targeted therapies that are designed to correct the underlying genetic defect. The heterogeneity of neurodegenerative diseases makes personalized medicine essential for effective treatment.
-
Targeting Non-neuronal Cells: Traditionally, neurodegenerative disease therapies have focused on protecting neurons. However, accumulating evidence highlights the crucial role of non-neuronal cells, such as astrocytes, microglia, and oligodendrocytes, in disease pathogenesis. Therapies targeting these cell types, to reduce inflammation or improve trophic support, for example, are gaining increasing attention.
Many thanks to our sponsor Esdebe who helped us prepare this research report.
7. Ethical Considerations
The use of advanced in vitro models, such as brain organoids, raises several ethical considerations:
-
Moral Status of Brain Organoids: As brain organoids become more complex and begin to exhibit more brain-like features, questions arise about their moral status. Some argue that brain organoids should be treated with the same respect as human beings. Others argue that brain organoids do not have the same moral status as human beings because they lack consciousness and self-awareness. Further research is needed to understand the potential for brain organoids to develop consciousness and self-awareness.
-
Informed Consent: When generating brain organoids from patient-derived hPSCs, it is essential to obtain informed consent from the patients. Patients should be informed about the purpose of the research, the potential risks and benefits, and their right to withdraw from the study. The use of patient-derived tissues and data must be carefully managed to protect patient privacy and confidentiality.
-
Commercialization: The commercialization of brain organoids raises concerns about access and affordability. It is important to ensure that brain organoid technology is accessible to researchers and patients from all backgrounds. Intellectual property rights should be managed in a way that promotes innovation and benefits the public.
-
Animal Welfare: While brain organoids can reduce the reliance on animal models in research, it is important to ensure that animal welfare is protected. Animal models should only be used when necessary and in accordance with ethical guidelines. The “3Rs” principles of replacement, reduction, and refinement should be applied to minimize the use of animals in research.
Many thanks to our sponsor Esdebe who helped us prepare this research report.
8. Conclusion
Neurodegenerative diseases pose a significant and growing global health challenge. Despite decades of research, effective disease-modifying therapies remain elusive. Traditional research approaches have limitations in recapitulating the complexity of the human brain and the chronic nature of neurodegeneration. Advanced in vitro models, such as 3D brain organoids, offer a promising alternative for studying neurodegenerative diseases in a more physiologically relevant context. These models can be used to investigate disease mechanisms, identify novel drug targets, and develop personalized medicine approaches. Emerging therapeutic avenues, such as gene therapy, immunotherapy, and small molecule drug development, hold promise for treating neurodegenerative diseases. However, ethical considerations surrounding the use of advanced in vitro models must be carefully addressed. Future research should focus on developing more complex and physiologically relevant in vitro models, validating therapeutic targets, and translating research findings to clinical practice. A holistic approach, combining advanced in vitro models with traditional research methods, is essential for accelerating the development of effective therapies for neurodegenerative diseases. The development of robust biomarkers for early diagnosis and disease progression monitoring remains a critical unmet need. The future of neurodegeneration research hinges on collaborative efforts across disciplines, including neuroscience, genetics, immunology, and bioengineering.
Many thanks to our sponsor Esdebe who helped us prepare this research report.
References
[1] Hardy, J., & Selkoe, D. J. (2002). The amyloid hypothesis of Alzheimer’s disease: progress and problems on the road to therapeutics. Science, 297(5580), 353-356.
[2] Spillantini, M. G., Schmidt, M. L., Lee, V. M. Y., Trojanowski, J. Q., Jakes, R., & Goedert, M. (1997). α-Synuclein in Lewy bodies. Nature, 388(6645), 839-840.
[3] Bates, G. P., Dorsey, R., Gusella, J. F., Hayden, M. R., Lawlor, B., Nance, M., … & Tabrizi, S. J. (2015). Huntington disease. Nature Reviews Disease Primers, 1(1), 1-21.
[4] Hardiman, O., Al-Chalabi, A., Chio, A., Corr, E. M., Logroscino, G., Robberecht, W., … & van den Berg, L. H. (2017). Amyotrophic lateral sclerosis. Nature Reviews Disease Primers, 3(1), 1-19.
[5] Bang, J., Spina, S., & Miller, B. L. (2015). Frontotemporal dementia. The Lancet, 386(9991), 167-180.
[6] Mullard, A. (2021). Controversial Alzheimer’s drug approved by FDA. Nature Reviews Drug Discovery, 20(7), 491-492.
[7] Lancaster, M. A., Renner, M., Martin, C. A., Wenzel, D., Bicknell, L. S., Collin, J., … & Huttner, W. B. (2013). Cerebral organoids model human brain development and microcephaly. Nature, 501(7467), 373-379.
The report mentions the potential of immunotherapy in treating Alzheimer’s by clearing Aβ plaques. Given the complex and often detrimental role of inflammation in neurodegeneration, how are researchers ensuring that immunotherapy strategies don’t inadvertently exacerbate harmful inflammatory responses in the brain?
That’s a crucial point! The interplay between Aβ clearance and inflammation is delicate. Researchers are exploring strategies like using modified antibodies that minimize inflammatory signaling and focusing on early-stage intervention to prevent the immune system from overreacting. It’s an active area of investigation!
Editor: MedTechNews.Uk
Thank you to our Sponsor Esdebe
The report highlights the potential of targeting non-neuronal cells. Could you elaborate on specific mechanisms by which astrocytes or microglia, for instance, contribute to the initiation or progression of these diseases, and how these mechanisms might be targeted therapeutically?