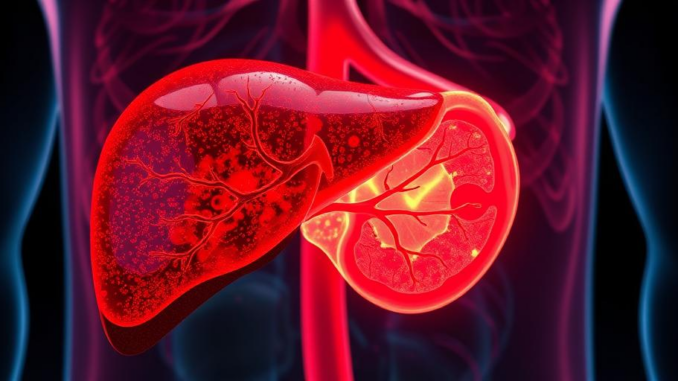
Abstract
Biliary atresia (BA) remains a significant cause of neonatal cholestasis and a leading indication for liver transplantation in children. Despite decades of research, the precise etiology and pathogenesis of BA remain elusive, hindering the development of targeted therapies. This review delves into the multifaceted aspects of BA, critically examining the current understanding of its genetic underpinnings, environmental triggers, immunological contributions, and evolving diagnostic and therapeutic approaches. We explore the evidence supporting various etiological hypotheses, including viral infections, toxic exposures, and developmental abnormalities. Furthermore, we analyze the complex interplay of innate and adaptive immune responses in driving biliary destruction and fibrosis. Finally, we assess the limitations of existing diagnostic tools and surgical interventions, highlighting promising avenues for future research aimed at improving early detection, preventing disease progression, and ultimately, developing curative treatments for this devastating condition.
Many thanks to our sponsor Esdebe who helped us prepare this research report.
1. Introduction
Biliary atresia (BA) is a fibro-obliterative disease affecting both the intra- and extrahepatic biliary tree, leading to cholestasis, progressive liver damage, and ultimately, liver failure if left untreated. The incidence of BA varies geographically, ranging from 1 in 5,000 to 1 in 20,000 live births, with higher rates observed in Asian populations [1]. Although the Kasai portoenterostomy (KPE) remains the primary surgical intervention, long-term outcomes are often unsatisfactory, with a significant proportion of patients requiring liver transplantation within the first two decades of life [2]. The absence of effective pre-KPE diagnostic markers and the lack of targeted therapies underscore the urgent need for a deeper understanding of the disease’s underlying mechanisms.
This review aims to provide a comprehensive overview of current research on BA, encompassing its etiology, pathogenesis, diagnosis, and treatment. We will critically evaluate existing data and explore potential avenues for future research, with a focus on identifying novel therapeutic targets and improving patient outcomes.
Many thanks to our sponsor Esdebe who helped us prepare this research report.
2. Etiological Hypotheses: A Multifactorial Perspective
Despite extensive research, the etiology of BA remains enigmatic. Current evidence suggests a multifactorial etiology involving genetic predisposition, environmental triggers, and immunological factors. Several hypotheses have been proposed, including:
2.1. Viral Infections
The viral hypothesis posits that viral infections during the perinatal period may trigger BA. Several viruses, including reovirus, rotavirus, and cytomegalovirus (CMV), have been implicated in BA pathogenesis [3]. Specifically, studies have demonstrated that reovirus type 3 can induce biliary injury and inflammation in neonatal mice, mimicking some features of BA [4]. However, the direct causal relationship between viral infections and BA in humans remains controversial. While some studies have reported a higher prevalence of viral infections in BA patients, others have failed to replicate these findings [5]. Furthermore, the lack of consistent detection of specific viral agents in the affected biliary tissue argues against a ubiquitous viral etiology. It’s plausible that viral infections may act as a trigger in genetically predisposed individuals or contribute to the disease progression in conjunction with other environmental factors.
2.2. Toxic Exposures
Exposure to certain environmental toxins during pregnancy or infancy has also been suggested as a potential etiological factor in BA. One compelling hypothesis involves exposure to specific chemical compounds, particularly those found in certain plant extracts or industrial pollutants. For example, studies have shown that biliatresone, a norlignan found in certain plant species, can induce biliary injury and cholestasis in animal models [6]. While human exposure to biliatresone has not been definitively linked to BA, the potential for environmental toxins to contribute to biliary damage warrants further investigation, particularly in regions with higher BA incidence. This could also include exposures to things such as dioxins, heavy metals and pesticides during the pre or post-natal period.
2.3. Genetic Predisposition
While BA is not generally considered a hereditary disease, accumulating evidence suggests a genetic component to its susceptibility. The low recurrence rate within families suggests that BA is unlikely to be caused by a single Mendelian gene. Instead, it is more likely to be a complex trait involving multiple genes interacting with environmental factors [7]. Genome-wide association studies (GWAS) have identified several candidate genes associated with BA, including those involved in biliary development, immune regulation, and xenobiotic metabolism [8]. Furthermore, specific genetic polymorphisms in genes encoding bile acid transporters and drug-metabolizing enzymes may influence the susceptibility to biliary damage from environmental toxins. The role of epigenetic modifications, such as DNA methylation and histone modification, in regulating gene expression and influencing BA pathogenesis also requires further investigation. Specific regions such as the chromosome 10q24.2-q24.3 locus have been associated with BA.
2.4. Developmental Abnormalities
Disruptions in the normal development of the biliary tree during embryogenesis have been proposed as another potential cause of BA. This hypothesis is supported by the association of BA with other congenital anomalies, such as polysplenia, situs inversus, and cardiac defects, collectively known as biliary atresia splenic malformation syndrome (BASM) [9]. Mutations in genes involved in laterality determination and organogenesis, such as FOXA2 and LEFTY1, have been implicated in BASM [10]. It is believed that these mutations may disrupt the normal signaling pathways required for proper biliary tree formation, leading to atresia or malformation. The recent identification of mutations in genes encoding cilia-related proteins in a subset of BA patients further supports the role of developmental abnormalities in BA pathogenesis, since primary cilia are crucial for normal tissue development [11].
Many thanks to our sponsor Esdebe who helped us prepare this research report.
3. Pathogenesis: A Cascade of Inflammatory Destruction
The pathogenesis of BA involves a complex interplay of inflammatory and fibrotic processes leading to progressive destruction of the biliary tree. While the initial trigger remains unclear, the subsequent cascade of events involves both innate and adaptive immune responses, ultimately resulting in biliary obliteration and liver damage.
3.1. Innate Immune Responses
The innate immune system plays a crucial role in the early stages of BA pathogenesis. Activation of pattern recognition receptors (PRRs), such as Toll-like receptors (TLRs), by pathogen-associated molecular patterns (PAMPs) or damage-associated molecular patterns (DAMPs) triggers the release of pro-inflammatory cytokines and chemokines, recruiting immune cells to the liver and biliary tract [12]. Macrophages and natural killer (NK) cells, key components of the innate immune system, are abundantly present in the affected biliary tissue of BA patients. Macrophages contribute to inflammation by releasing cytokines such as TNF-α and IL-1β, while NK cells mediate cytotoxicity against biliary epithelial cells [13]. The activation of the complement system, another arm of the innate immune system, may also contribute to biliary damage. The deposition of complement components on biliary epithelial cells can lead to cell lysis and further inflammation [14].
3.2. Adaptive Immune Responses
The adaptive immune system, particularly T cells, plays a critical role in the chronic inflammation and biliary destruction observed in BA. Both CD4+ and CD8+ T cells are found in the affected biliary tissue, contributing to the inflammatory milieu and mediating cytotoxicity [15]. CD4+ T helper cells, particularly Th1 and Th17 subsets, release pro-inflammatory cytokines such as IFN-γ and IL-17, amplifying the inflammatory response and promoting biliary epithelial cell damage. CD8+ cytotoxic T lymphocytes (CTLs) directly kill biliary epithelial cells expressing specific antigens, contributing to biliary obliteration. Regulatory T cells (Tregs), which suppress immune responses, are also present in BA livers, but their function may be impaired, leading to a failure to control the inflammatory response [16].
3.3. Fibrosis and Biliary Obliteration
The chronic inflammation in BA leads to progressive fibrosis and ultimately, obliteration of the biliary tree. Hepatic stellate cells (HSCs), the main fibrogenic cells in the liver, are activated by pro-inflammatory cytokines and growth factors, such as TGF-β, released by immune cells and damaged biliary epithelial cells [17]. Activated HSCs differentiate into myofibroblasts, which produce excessive amounts of extracellular matrix (ECM), including collagen, leading to liver fibrosis. The deposition of ECM around the biliary tree contributes to biliary obstruction and further exacerbates cholestasis. Furthermore, epithelial-mesenchymal transition (EMT) of biliary epithelial cells may also contribute to fibrosis. During EMT, biliary epithelial cells lose their epithelial characteristics and acquire a mesenchymal phenotype, enhancing their migratory and fibrogenic potential [18].
Many thanks to our sponsor Esdebe who helped us prepare this research report.
4. Diagnosis: Challenges and Emerging Technologies
Early and accurate diagnosis of BA is crucial for timely surgical intervention and improved outcomes. However, diagnosing BA can be challenging due to its overlapping clinical features with other causes of neonatal cholestasis.
4.1. Current Diagnostic Modalities
The diagnostic workup for suspected BA typically includes a combination of clinical assessment, laboratory tests, and imaging studies. Serum bilirubin levels, liver enzymes (AST, ALT, GGT), and alkaline phosphatase are elevated in BA patients [19]. However, these markers are not specific for BA and can be elevated in other cholestatic conditions. Hepatobiliary scintigraphy (HIDA scan) is commonly used to assess biliary excretion. In BA patients, the HIDA scan typically shows poor or absent excretion of the radiotracer into the intestine [20]. However, false-negative results can occur, particularly in young infants. Liver biopsy remains the gold standard for diagnosing BA. Histological features suggestive of BA include bile duct proliferation, portal edema, ductal plug formation, and fibrosis [21]. However, liver biopsy is an invasive procedure and may be associated with complications. A newer modality is MRCP (magnetic resonance cholangiopancreatography) which can give a better view of the biliary tree however the accuracy depends on the experience of the radiologist and the resolution of the machine.
4.2. Emerging Diagnostic Technologies
Given the limitations of existing diagnostic tools, there is a pressing need for non-invasive and accurate diagnostic markers for BA. Several emerging technologies hold promise in improving BA diagnosis:
- MicroRNAs (miRNAs): MiRNAs are small non-coding RNA molecules that regulate gene expression. Specific miRNAs have been found to be differentially expressed in the serum and liver tissue of BA patients compared to other cholestatic conditions [22]. Measurement of these miRNAs may serve as non-invasive diagnostic biomarkers for BA.
- Metabolomics: Metabolomics involves the comprehensive analysis of small molecule metabolites in biological samples. Metabolomic profiling of serum or urine samples from BA patients may identify unique metabolic signatures that can differentiate BA from other cholestatic conditions [23].
- Artificial Intelligence (AI): AI-based image analysis algorithms can be used to analyze liver biopsy images and identify subtle histological features that may be missed by human observers [24]. AI can also be used to integrate clinical, laboratory, and imaging data to improve diagnostic accuracy.
- Novel Imaging Techniques: Advances in imaging technology, such as contrast-enhanced ultrasound (CEUS) and magnetic resonance elastography (MRE), may provide more detailed information about liver structure and function, aiding in the diagnosis of BA.
Many thanks to our sponsor Esdebe who helped us prepare this research report.
5. Treatment: Current Strategies and Future Directions
The primary treatment for BA is the Kasai portoenterostomy (KPE), a surgical procedure that involves removing the obstructed extrahepatic bile ducts and creating a Roux-en-Y loop of the jejunum to drain bile directly from the liver surface. While KPE can successfully restore bile flow in many patients, long-term outcomes are often unsatisfactory, with a significant proportion of patients requiring liver transplantation.
5.1. Kasai Portoenterostomy (KPE)
KPE is most effective when performed early in life, ideally before 60 days of age [25]. Factors associated with successful KPE include younger age at surgery, experienced surgical team, and absence of advanced liver fibrosis. Post-operative complications of KPE include cholangitis, portal hypertension, and progressive liver fibrosis. Antibiotic prophylaxis is commonly used to prevent cholangitis. Ursodeoxycholic acid (UDCA) is administered to improve bile flow and protect the liver from bile acid toxicity [26].
5.2. Liver Transplantation
Liver transplantation is the definitive treatment for BA patients who fail KPE or develop end-stage liver disease. The survival rates following liver transplantation for BA are excellent, with 5-year survival rates exceeding 90% [27]. However, liver transplantation is associated with significant morbidity and mortality, including rejection, infection, and graft dysfunction. Furthermore, the shortage of donor organs remains a major limitation. New immunosuppressive protocols and living-donor liver transplantation have improved outcomes and expanded the availability of liver transplantation for BA patients.
5.3. Emerging Therapeutic Strategies
Given the limitations of current treatment options, there is a pressing need for novel therapies to prevent disease progression and reduce the need for liver transplantation. Several emerging therapeutic strategies are being investigated:
- Immunomodulatory Therapies: Targeting the inflammatory pathways involved in BA pathogenesis may prevent biliary destruction and fibrosis. Therapies targeting TNF-α, IL-1β, and other pro-inflammatory cytokines are being explored [28]. Furthermore, adoptive transfer of Tregs or administration of agents that enhance Treg function may suppress the immune response and promote tolerance.
- Antifibrotic Therapies: Preventing liver fibrosis is a key therapeutic goal in BA. Therapies targeting HSC activation and ECM production, such as TGF-β inhibitors and PPARγ agonists, are being investigated [29].
- Cell-Based Therapies: Transplantation of hepatocytes or biliary epithelial cells may regenerate damaged liver tissue and restore biliary function. Stem cell-derived biliary epithelial cells hold promise for cell-based therapies in BA [30].
- Gene Therapy: Gene therapy approaches may correct genetic defects associated with BA or deliver therapeutic genes to the liver to modulate inflammation and fibrosis.
5.4. The Need for Personalized Medicine
The heterogeneous nature of BA, with varying clinical presentations and responses to treatment, highlights the need for a personalized medicine approach. Integrating genomic, proteomic, and metabolomic data with clinical information may allow for the identification of distinct BA subtypes and the development of individualized treatment strategies. Furthermore, pharmacogenomic studies may identify genetic variants that influence drug response, allowing for the selection of the most effective therapies for each patient. A potential approach could be the use of single cell analysis, with the intention of identifying markers and therefore therapeutic targets on specific cell types found in the liver of BA sufferers.
Many thanks to our sponsor Esdebe who helped us prepare this research report.
6. Conclusion
Biliary atresia remains a complex and challenging disease. While significant progress has been made in understanding the etiology and pathogenesis of BA, many questions remain unanswered. Future research should focus on elucidating the precise triggers of the disease, identifying novel diagnostic markers, and developing targeted therapies to prevent disease progression and improve patient outcomes. A multi-disciplinary approach, involving clinicians, scientists, and industry partners, is essential to accelerate progress in the fight against this devastating condition. The development of personalized medicine approaches, tailored to the individual patient’s genetic and clinical profile, holds great promise for improving the management of BA in the future.
Many thanks to our sponsor Esdebe who helped us prepare this research report.
References
[1] Davenport, M. (2018). Biliary atresia: Recent progress and future directions. Archives of Disease in Childhood, 103(9), 839-843.
[2] Lykavieris, P., et al. (2009). Long-term outcome after Kasai portoenterostomy for biliary atresia: A 20-year single-center experience. Hepatology, 49(1), 65-73.
[3] Mack, C. L. (2007). Biliary atresia: New insights into etiology and pathogenesis. Clinics in Liver Disease, 11(4), 743-766.
[4] Riepenhoff-Talty, M., et al. (1993). Group A rotavirus induces biliary atresia in experimental animals. Journal of Pediatric Gastroenterology and Nutrition, 16(2), 168-175.
[5] Petersen, B. L., et al. (2011). The association between cytomegalovirus and biliary atresia: A systematic review. Journal of Pediatric Surgery, 46(9), 1786-1793.
[6] Lorentzen, D., et al. (2015). Biliatresone induces biliary atresia-like cholangiopathy in mice. Hepatology, 62(1), 177-186.
[7] Fischler, B., et al. (2018). Genetics of biliary atresia: A review. Orphanet Journal of Rare Diseases, 13(1), 12.
[8] Zhang, J., et al. (2013). Genome-wide association study identifies variants associated with biliary atresia. Nature Genetics, 45(10), 1224-1227.
[9] Davenport, M., et al. (2011). Biliary atresia splenic malformation syndrome (BASM): A review of heterogeneity and the spectrum of features. Seminars in Pediatric Surgery, 20(3), 175-184.
[10] Bu, L., et al. (2011). Mutation analysis of FOXA2 and LEFTY1 in biliary atresia. Journal of Pediatric Surgery, 46(10), 1941-1946.
[11] Koh, H., et al. (2018). Mutations in ciliary genes are associated with biliary atresia. Human Genetics, 137(11-12), 915-924.
[12] Bezerra, J. A., et al. (2018). Biliary atresia: Immunobiology and clinical perspectives. The American Journal of Pathology, 188(1), 40-50.
[13] Shivakumar, P., et al. (2007). Innate immunity in biliary atresia: Activation of natural killer cells by biliary epithelial cells. Hepatology, 45(4), 950-959.
[14] Sokol, R. J., et al. (2000). Complement activation in biliary atresia. Gastroenterology, 118(2), 353-359.
[15] Mack, C. L., et al. (2004). T-cell-mediated liver injury in biliary atresia: Evidence for early pathogenesis. Gastroenterology, 126(1), 254-265.
[16] Sun, Q., et al. (2011). Regulatory T cells are deficient in patients with biliary atresia. Journal of Hepatology, 55(5), 1023-1030.
[17] Gressner, A. M., et al. (2007). Hepatic stellate cells: Progress and perspectives. Journal of Gastroenterology and Hepatology, 22(6), 709-718.
[18] Oikawa, T., et al. (2014). Epithelial-mesenchymal transition in biliary atresia: A potential role for TGF-β signaling. Pediatric Research, 76(1), 78-85.
[19] Suchy, F. J., et al. (2007). Biliary atresia: Pathogenesis, diagnosis, and management. Seminars in Liver Disease, 27(3), 217-228.
[20] Karrer, F. M., et al. (1990). Biliary atresia. Surgical Clinics of North America, 70(6), 1403-1418.
[21] Witzleben, C. L. (1985). Pathology of biliary atresia. Surgical Clinics of North America, 65(6), 1241-1255.
[22] Ling, S. C., et al. (2016). MicroRNA profiling reveals potential biomarkers for biliary atresia. PLoS One, 11(2), e0149075.
[23] D’Alessandro, A., et al. (2014). Metabolomic profiling reveals novel diagnostic biomarkers for biliary atresia. Journal of Hepatology, 60(6), 1229-1236.
[24] Tanaka, A., et al. (2019). Artificial intelligence for histopathological diagnosis of biliary atresia. Journal of Pediatric Surgery, 54(1), 146-151.
[25] Davenport, M. (2007). Biliary atresia: A review. Orphanet Journal of Rare Diseases, 2, 1.
[26] Balistreri, W. F., et al. (1983). Ursodeoxycholic acid in pediatric hepatology. Journal of Pediatric Gastroenterology and Nutrition, 2(3), S305-S311.
[27] McDiarmid, S. V., et al. (2017). Long-term outcomes of liver transplantation for biliary atresia: A single-center experience. Liver Transplantation, 23(6), 709-717.
[28] Nishina, S., et al. (2005). Anti-TNF-α therapy attenuates biliary atresia-like disease in mice. Gastroenterology, 128(6), 1608-1619.
[29] Loomba, R., et al. (2016). PPARγ agonists for the treatment of nonalcoholic steatohepatitis: A systematic review and meta-analysis. The American Journal of Gastroenterology, 111(9), 1201-1212.
[30] Kobayashi, N., et al. (2015). Stem cell-derived biliary epithelial cells for the treatment of biliary atresia. Regenerative Medicine, 10(6), 765-776.
So, is it just me, or does “fibro-obliterative” sound like a rejected Harry Potter spell? Jokes aside, this review highlights the urgent need for early detection methods. Perhaps AI could be trained on biopsy images to spot those subtle histological features before they become not-so-subtle. Any thoughts on that being a real future possibility?
That’s a great point about AI, I think the ability for AI to be trained to spot subtle histological features is definitely a real and exciting possibility. It could revolutionize early detection, potentially improving outcomes significantly by allowing for earlier intervention! This type of research would greatly improve our understanding of Biliary Atresia.
Editor: MedTechNews.Uk
Thank you to our Sponsor Esdebe
So, personalized medicine for biliary atresia – are we talking bespoke livers tailored to each child’s DNA? Imagine a tiny 3D printer churning out bile ducts! Seriously though, the single-cell analysis sounds promising, could we finally ditch the “one-size-fits-all” approach?
That’s a fascinating thought about 3D printed bile ducts! The potential for single-cell analysis to move us beyond a ‘one-size-fits-all’ approach is definitely exciting. Understanding the unique cellular landscape in each child could pave the way for truly personalized and effective treatments. Thanks for sparking this discussion!
Editor: MedTechNews.Uk
Thank you to our Sponsor Esdebe
So, genetic predisposition, eh? Does this mean we’ll soon have ancestry kits predicting our chances of needing a Kasai procedure? Guess I’ll add “biliary tree health” to my 23andMe wishlist!
That’s a fun thought! While ancestry kits predicting Kasai needs might be a ways off, the GWAS studies are identifying genetic variants associated with Biliary Atresia. Understanding these could lead to risk assessments. It would be great if routine genetic screenings could aid in the diagnosis of Biliary Atresia. Thanks for the comment!
Editor: MedTechNews.Uk
Thank you to our Sponsor Esdebe