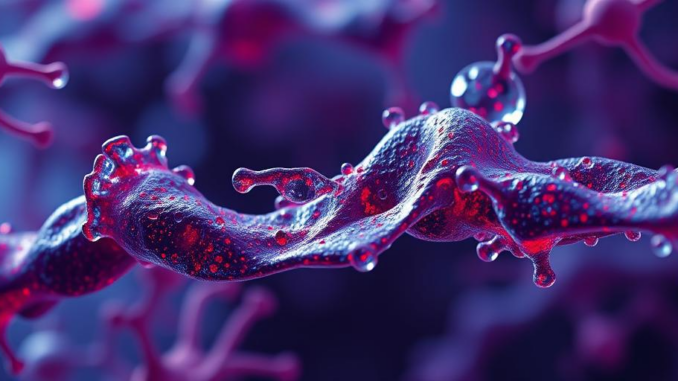
Amylin: A Comprehensive Review of its Physiological Roles, Therapeutic Potential, and the Evolving Landscape of Amylin-Based Therapeutics
Abstract
Amylin, also known as islet amyloid polypeptide (IAPP), is a 37-amino acid peptide hormone co-secreted with insulin from pancreatic β-cells. While historically recognized for its role in islet amyloid formation in type 2 diabetes (T2D), amylin’s physiological significance extends far beyond this association. This review provides a comprehensive overview of amylin, encompassing its diverse physiological roles in regulating appetite, gastric emptying, and glucose metabolism. It delves into the intricate mechanisms of action by which amylin exerts its effects, focusing on its interactions with specific brain regions and peripheral tissues. Furthermore, the therapeutic potential of amylin analogs, such as pramlintide (Symlin), in the management of diabetes and obesity is examined, along with a critical assessment of the challenges encountered in amylin research, including stability issues and the potential for amyloidogenesis. Finally, the review highlights recent advancements in amylin research, including the development of novel amylin analogs with improved pharmacological properties and their potential applications beyond glycemic control, particularly in the context of combination therapies like amycretin, paving the way for more effective and targeted therapeutic strategies.
1. Introduction
Amylin was first identified as the major component of amyloid deposits found in the islets of Langerhans of individuals with T2D [1]. Initially considered a pathological entity, research has progressively unveiled the critical physiological roles of amylin in glucose homeostasis and appetite regulation. Amylin is co-secreted with insulin in response to nutrient stimuli, and its actions complement those of insulin. Specifically, amylin contributes to postprandial glucose control by suppressing glucagon secretion, slowing gastric emptying, and promoting satiety [2].
The clinical relevance of amylin was established with the development and approval of pramlintide, a synthetic analog of human amylin, for the treatment of T2D [3]. Pramlintide represents the first in a new class of drugs designed to address the multifaceted pathophysiology of T2D by targeting postprandial glucose excursions. However, the limitations associated with pramlintide, primarily related to its propensity for aggregation and the need for multiple daily injections, have spurred ongoing research into novel amylin analogs with improved pharmacological profiles.
Recently, amylin has gained renewed attention due to its inclusion as a key component in the development of novel combination therapies such as amycretin. Amycretin combines an amylin receptor agonist with a GLP-1 receptor agonist, aiming to leverage the synergistic effects of these two hormones to achieve superior weight loss and glycemic control [4]. This resurgence of interest underscores the importance of a comprehensive understanding of amylin’s physiology, mechanisms of action, and the challenges and opportunities associated with amylin-based therapeutics. This review aims to provide such a comprehensive overview, with a focus on its relevance to emerging combination therapies and future directions in amylin research.
2. Physiological Roles of Amylin
Amylin exerts its physiological effects through a complex interplay of actions involving the central nervous system (CNS) and peripheral tissues. These actions collectively contribute to the regulation of glucose metabolism, appetite, and gastric emptying. A detailed examination of these roles is crucial for understanding the therapeutic potential and limitations of amylin-based therapies.
2.1. Glucose Metabolism
Amylin plays a significant role in postprandial glucose regulation. It does so primarily through three mechanisms:
- Suppression of Glucagon Secretion: Amylin directly inhibits glucagon secretion from pancreatic α-cells [5]. This is particularly important in preventing excessive hepatic glucose production following a meal. The exact mechanism of this inhibition is complex and involves both direct effects on α-cells and indirect effects mediated by the CNS.
- Slowing Gastric Emptying: Amylin slows the rate at which food empties from the stomach into the small intestine [6]. This reduces the rate of glucose absorption, thereby preventing postprandial glucose spikes. This action is mediated through vagal afferent nerve stimulation and subsequent effects on gastric motility.
- Indirect Effects on Insulin Secretion: While amylin doesn’t directly stimulate insulin secretion, it can indirectly modulate insulin release. By slowing gastric emptying and suppressing glucagon, amylin can reduce the demand for insulin, potentially preserving β-cell function over time. However, this effect is less pronounced than the direct effects of GLP-1 receptor agonists on insulin secretion.
2.2. Appetite Regulation and Satiety
Amylin is a potent satiety hormone. It acts on specific brain regions involved in appetite control, primarily the area postrema (AP) and the nucleus of the solitary tract (NTS) in the brainstem [7]. These regions lack a blood-brain barrier, allowing amylin to directly access these critical regulatory centers. Stimulation of these areas leads to:
- Reduced Food Intake: Amylin decreases food consumption by promoting feelings of fullness and reducing hunger. This effect is mediated by increasing the activity of anorexigenic (appetite-suppressing) pathways and decreasing the activity of orexigenic (appetite-stimulating) pathways in the brain.
- Increased Satiety: Amylin enhances the feeling of fullness after a meal, contributing to overall appetite control. This effect is likely mediated by both central and peripheral mechanisms, including the slowing of gastric emptying, which prolongs the feeling of satiety.
The contribution of amylin to satiety and appetite regulation is significant. While other hormones like leptin and ghrelin also play important roles, amylin’s rapid action and specific targeting of brain regions involved in satiety make it a valuable target for therapeutic interventions aimed at weight management.
2.3. Other Physiological Roles
Emerging evidence suggests that amylin may have other physiological roles beyond glucose and appetite regulation, including:
- Bone Metabolism: Some studies indicate that amylin may influence bone metabolism, potentially stimulating bone formation and inhibiting bone resorption [8]. The mechanisms underlying these effects are not fully understood but may involve direct effects on osteoblasts and osteoclasts or indirect effects mediated by other hormones.
- Renal Function: Amylin receptors have been identified in the kidneys, suggesting a potential role in renal function. However, the specific effects of amylin on renal physiology remain to be fully elucidated. Some studies suggest that amylin may influence sodium excretion and blood pressure regulation [9].
- Cardiovascular Function: The role of amylin in cardiovascular function is complex and not fully understood. Some studies have suggested a potential protective effect of amylin on the cardiovascular system, while others have raised concerns about potential adverse effects in certain contexts. Further research is needed to clarify the role of amylin in cardiovascular physiology.
3. Mechanisms of Action
Understanding the mechanisms by which amylin exerts its physiological effects is crucial for developing more effective and targeted amylin-based therapies. Amylin primarily acts through a receptor complex located in the brainstem, particularly in the AP and NTS. This receptor complex is distinct from the receptors for other related peptides, such as calcitonin gene-related peptide (CGRP) and calcitonin. The amylin receptor complex consists of the calcitonin receptor (CTR) and receptor activity-modifying proteins (RAMPs), specifically RAMP1, RAMP2, or RAMP3 [10]. The specific combination of RAMPs determines the binding affinity and signaling properties of the receptor complex.
3.1. Receptor Binding and Signaling
Amylin preferentially binds to the CTR in combination with RAMPs, particularly RAMP1 and RAMP3. This binding initiates a cascade of intracellular signaling events, including:
- Activation of Adenylyl Cyclase (AC) and Cyclic AMP (cAMP) Production: Amylin binding to its receptor stimulates AC, leading to an increase in intracellular cAMP levels. cAMP then activates protein kinase A (PKA), which phosphorylates various downstream targets, ultimately leading to changes in neuronal excitability and gene expression.
- Activation of Phospholipase C (PLC) and Inositol Trisphosphate (IP3) Production: Amylin can also activate PLC, leading to the production of IP3 and diacylglycerol (DAG). IP3 releases calcium from intracellular stores, while DAG activates protein kinase C (PKC). These pathways contribute to the overall signaling response to amylin.
- Activation of Mitogen-Activated Protein Kinase (MAPK) Pathways: Amylin can activate MAPK pathways, including ERK1/2 and p38 MAPK. These pathways are involved in regulating cell growth, differentiation, and survival, and they may contribute to the long-term effects of amylin on appetite regulation and metabolism.
The specific signaling pathways activated by amylin depend on the cell type and the receptor complex composition. The relative contribution of each pathway to the overall physiological effects of amylin is an area of ongoing research.
3.2. Neuronal Circuitry and Brain Regions
Amylin’s effects on appetite regulation are primarily mediated by its actions on specific neuronal circuits in the brainstem. The AP and NTS are critical relay stations for sensory information from the periphery, including signals related to gastric distension, nutrient availability, and hormone levels. Amylin activates neurons in these regions, which then project to other brain areas involved in appetite control, such as the hypothalamus and the amygdala [11].
The hypothalamus plays a central role in regulating energy balance. Amylin influences the activity of key hypothalamic neurons, including:
- Pro-opiomelanocortin (POMC) Neurons: Amylin activates POMC neurons, which produce α-melanocyte-stimulating hormone (α-MSH), a potent anorexigenic peptide. Activation of POMC neurons contributes to the appetite-suppressing effects of amylin.
- Agouti-Related Peptide (AgRP) Neurons: Amylin inhibits AgRP neurons, which produce AgRP, an orexigenic peptide. Inhibition of AgRP neurons further contributes to the appetite-suppressing effects of amylin.
The amygdala is involved in processing emotional and motivational aspects of eating. Amylin can modulate the activity of amygdala neurons, potentially influencing food preferences and reward-related eating behavior. The precise mechanisms by which amylin affects amygdala function are still under investigation.
3.3. Peripheral Mechanisms
While the central effects of amylin are well-established, amylin also exerts effects on peripheral tissues, including the stomach, pancreas, and kidneys. These peripheral actions contribute to the overall physiological effects of amylin.
- Gastric Emptying: Amylin slows gastric emptying by stimulating vagal afferent nerves in the stomach. This effect is mediated by the release of cholecystokinin (CCK) and other gastrointestinal hormones. The slowing of gastric emptying contributes to the postprandial glucose control and satiety-promoting effects of amylin.
- Pancreatic Glucagon Secretion: Amylin directly inhibits glucagon secretion from pancreatic α-cells. This effect is mediated by a direct action on α-cells, as well as indirect effects mediated by the CNS. The suppression of glucagon secretion contributes to the postprandial glucose control effects of amylin.
- Renal Function: Amylin receptors have been identified in the kidneys, suggesting a potential role in renal function. However, the specific effects of amylin on renal physiology remain to be fully elucidated. Some studies suggest that amylin may influence sodium excretion and blood pressure regulation.
4. Therapeutic Applications of Amylin
The therapeutic potential of amylin has been recognized since the development and approval of pramlintide for the treatment of T2D. However, ongoing research is exploring the potential of amylin analogs for a broader range of therapeutic applications, including obesity management and potentially other metabolic disorders.
4.1. Pramlintide (Symlin) in Type 2 Diabetes
Pramlintide is a synthetic analog of human amylin approved for use as an adjunct to insulin therapy in patients with T2D. Pramlintide improves glycemic control by:
- Reducing Postprandial Glucose Excursions: Pramlintide slows gastric emptying and suppresses glucagon secretion, thereby reducing postprandial glucose spikes. This effect complements the action of insulin and can significantly improve overall glycemic control.
- Promoting Weight Loss: Pramlintide promotes weight loss by reducing food intake and increasing satiety. This effect is particularly beneficial for patients with T2D who are overweight or obese.
- Reducing Insulin Dose Requirements: Pramlintide can reduce the amount of insulin needed to achieve glycemic control. This can help to reduce the risk of hypoglycemia, a common side effect of insulin therapy.
While pramlintide is effective in improving glycemic control and promoting weight loss, its use is limited by the need for multiple daily injections and the potential for nausea. These limitations have spurred the development of novel amylin analogs with improved pharmacological properties.
4.2. Amylin Analogs for Obesity Management
Given amylin’s potent effects on appetite regulation and satiety, amylin analogs are being investigated as potential therapies for obesity management. Clinical trials have shown that amylin analogs can produce significant weight loss in obese individuals, even in the absence of diabetes [12]. The mechanisms underlying this weight loss include:
- Reduced Food Intake: Amylin analogs decrease food consumption by promoting feelings of fullness and reducing hunger.
- Increased Satiety: Amylin analogs enhance the feeling of fullness after a meal, contributing to overall appetite control.
- Increased Energy Expenditure: Some studies suggest that amylin analogs may increase energy expenditure, potentially contributing to weight loss.
The development of long-acting amylin analogs with improved tolerability is a key focus of ongoing research in this area. Such analogs could provide a more convenient and effective treatment option for obesity.
4.3. Beyond Glycemic Control and Weight Loss
Emerging research suggests that amylin analogs may have therapeutic potential beyond glycemic control and weight loss, including:
- Neuroprotection: Some studies suggest that amylin may have neuroprotective effects, potentially protecting against neuronal damage in neurodegenerative diseases such as Alzheimer’s disease [13]. The mechanisms underlying these effects are not fully understood but may involve antioxidant and anti-inflammatory actions.
- Bone Health: As mentioned earlier, amylin may influence bone metabolism, potentially stimulating bone formation and inhibiting bone resorption. This suggests that amylin analogs may have therapeutic potential for osteoporosis and other bone disorders.
- Cardiovascular Disease: The role of amylin in cardiovascular disease is complex and not fully understood. However, some studies suggest that amylin may have protective effects on the cardiovascular system, potentially reducing the risk of heart disease and stroke. More research is needed to confirm these findings.
5. Challenges and Advancements in Amylin Research
Despite the promising therapeutic potential of amylin, several challenges have hampered its development and clinical application. These challenges include the propensity of amylin to aggregate and form amyloid fibrils, its short half-life in vivo, and the potential for nausea and other gastrointestinal side effects. However, significant advancements have been made in recent years to overcome these challenges.
5.1. Amyloidogenesis and Stability
A major challenge in amylin research is the propensity of native human amylin to aggregate and form amyloid fibrils. This aggregation can lead to the formation of amyloid deposits in the pancreatic islets, contributing to β-cell dysfunction and T2D. Furthermore, the aggregation of amylin in pharmaceutical formulations can reduce its efficacy and increase the risk of adverse effects. Therefore, significant efforts have been directed towards developing amylin analogs with improved stability and reduced amyloidogenic potential.
Pramlintide incorporates proline substitutions at positions 25, 28, and 29, which disrupt the β-sheet structure and reduce aggregation [14]. Other strategies for improving amylin stability include:
- Amino Acid Modifications: Introducing other amino acid substitutions or modifications can further enhance amylin stability and reduce its amyloidogenic potential.
- Chemical Modifications: Modifying amylin with polyethylene glycol (PEGylation) or other chemical groups can increase its solubility and reduce aggregation.
- Formulation Strategies: Developing novel formulation strategies, such as the use of excipients and stabilizers, can also improve amylin stability.
5.2. Pharmacokinetics and Delivery
Native human amylin has a short half-life in vivo, which necessitates frequent injections to maintain therapeutic levels. Pramlintide also has a relatively short half-life, requiring multiple daily injections. Therefore, the development of long-acting amylin analogs is a key goal of ongoing research.
Strategies for improving amylin pharmacokinetics include:
- Amino Acid Modifications: Introducing amino acid substitutions that reduce proteolytic degradation can prolong amylin’s half-life.
- PEGylation: Attaching polyethylene glycol (PEG) molecules to amylin can increase its size and reduce its rate of clearance from the circulation.
- Lipidation: Attaching lipid moieties to amylin can promote its association with albumin, prolonging its half-life.
- Controlled-Release Formulations: Developing controlled-release formulations, such as microspheres or nanoparticles, can provide sustained release of amylin over extended periods.
5.3. Tolerability and Side Effects
The most common side effect associated with pramlintide is nausea, which can limit its use in some patients. Other gastrointestinal side effects, such as vomiting and anorexia, may also occur. These side effects are thought to be mediated by the activation of the area postrema in the brainstem, which is involved in regulating nausea and vomiting [15].
Strategies for improving amylin tolerability include:
- Dose Titration: Starting with a low dose of amylin and gradually increasing it can help to reduce the risk of nausea.
- Analog Design: Developing amylin analogs with reduced affinity for the area postrema can minimize the risk of nausea.
- Combination Therapies: Combining amylin analogs with other anti-nausea medications can help to improve tolerability.
5.4. Advancements in Amylin Research
Despite the challenges, significant advancements have been made in amylin research in recent years, including:
- Development of Novel Amylin Analogs: Researchers have developed numerous novel amylin analogs with improved stability, pharmacokinetics, and tolerability. These analogs are being evaluated in preclinical and clinical studies for the treatment of diabetes and obesity.
- Understanding Amylin Receptor Structure and Function: Advances in structural biology and molecular biology have provided new insights into the structure and function of the amylin receptor. This knowledge is being used to design more potent and selective amylin receptor agonists.
- Combination Therapies: The development of combination therapies, such as amycretin (amylin receptor agonist + GLP-1 receptor agonist), represents a promising approach to leveraging the synergistic effects of multiple hormones to achieve superior weight loss and glycemic control. This reflects a trend toward multi-agonist strategies to treat metabolic diseases.
- Targeting Specific Brain Regions: Researchers are exploring strategies for targeting amylin analogs to specific brain regions involved in appetite regulation. This approach could potentially minimize side effects and enhance efficacy.
6. Amylin and Amycretin: A Synergistic Approach
The recent development of amycretin, a combination therapy comprising an amylin receptor agonist and a GLP-1 receptor agonist, represents a significant advancement in the treatment of obesity and T2D [4]. This synergistic approach leverages the complementary actions of these two hormones to achieve superior weight loss and glycemic control compared to either agent alone.
The rationale for combining amylin and GLP-1 receptor agonists is based on their distinct but complementary mechanisms of action:
- Amylin: Primarily acts to slow gastric emptying, suppress glucagon secretion, and promote satiety.
- GLP-1 Receptor Agonists: Primarily act to stimulate insulin secretion, suppress glucagon secretion, and promote satiety.
By combining these two agents, amycretin can effectively address multiple aspects of glucose metabolism and appetite regulation, leading to improved glycemic control, weight loss, and potentially other metabolic benefits.
The clinical trials of amycretin have demonstrated remarkable weight loss results, exceeding those typically observed with either amylin analogs or GLP-1 receptor agonists alone. These findings suggest that the synergistic combination of amylin and GLP-1 receptor agonists represents a powerful new approach to the treatment of obesity and T2D.
7. Future Directions
Amylin research is a dynamic and rapidly evolving field. Future research directions include:
- Development of Novel Amylin Analogs: Continued development of novel amylin analogs with improved stability, pharmacokinetics, and tolerability.
- Personalized Medicine: Identifying biomarkers that can predict individual responses to amylin-based therapies.
- Long-Term Safety and Efficacy Studies: Conducting long-term studies to evaluate the safety and efficacy of amylin analogs in preventing the progression of T2D and obesity-related complications.
- Exploring Novel Therapeutic Applications: Investigating the potential of amylin analogs for other therapeutic applications, such as neuroprotection and bone health.
- Understanding the Role of Amylin in Other Metabolic Disorders: Investigating the role of amylin in the pathogenesis and treatment of other metabolic disorders, such as non-alcoholic fatty liver disease (NAFLD) and polycystic ovary syndrome (PCOS).
8. Conclusion
Amylin is a key hormone involved in glucose homeostasis and appetite regulation. Its therapeutic potential has been demonstrated by the approval of pramlintide for the treatment of T2D. The development of novel amylin analogs with improved pharmacological properties and the emergence of combination therapies like amycretin hold promise for the treatment of obesity and other metabolic disorders. Ongoing research is focused on overcoming the challenges associated with amylin-based therapies and exploring its potential for a broader range of therapeutic applications. As our understanding of amylin’s physiology and mechanisms of action continues to grow, it is likely that amylin-based therapies will play an increasingly important role in the management of metabolic diseases in the future.
References
[1] Cooper, G. J. S., et al. (1987). Purification and characterization of a peptide from amyloid-rich pancreatic extracts of diabetic patients. Proceedings of the National Academy of Sciences, 84(23), 8628-8632.
[2] Young, A. A. (2006). Amylin physiology and its role in glucose control. American Journal of Medicine, 119(6 Suppl 1), S27-S36.
[3] Kolterman, O. G., et al. (2006). Pramlintide, the synthetic amylin analog: A review of the physiology, pharmacology, and clinical data. Diabetes Technology & Therapeutics, 8(5), 573-588.
[4] Structurally Modified Amylin-Analog/GLP-1R Co-Agonists and Methods of Use. WO2024051567A1. Published March 14, 2024.
[5] D’Alessio, D. A., et al. (2004). Amylin inhibits glucagon secretion in humans. American Journal of Physiology-Endocrinology and Metabolism, 286(1), E108-E114.
[6] Edelbroek, M. A. L., et al. (1999). Amylin decreases gastric emptying in healthy humans. American Journal of Physiology-Gastrointestinal and Liver Physiology, 277(3), G309-G313.
[7] Lutz, T. A., & Meyer-Gerspach, A. C. (2007). The role of amylin in the control of energy balance. American Journal of Physiology-Regulatory, Integrative and Comparative Physiology, 292(3), R1083-R1095.
[8] Cornish, J., et al. (2001). Amylin stimulates osteoblast proliferation and inhibits osteoclast formation in vitro. Endocrinology, 142(11), 4615-4623.
[9] Stevenson, R. W., et al. (2010). Amylin stimulates renal sodium excretion via a central nervous system mechanism in rats. American Journal of Physiology-Regulatory, Integrative and Comparative Physiology, 298(4), R1009-R1017.
[10] Christopoulos, A., et al. (1999). The calcitonin receptor-like receptor and receptor activity-modifying proteins. Molecular Pharmacology, 56(5), 1011-1020.
[11] Riediger, T., et al. (2003). Amylin acts in the area postrema to reduce food intake. American Journal of Physiology-Regulatory, Integrative and Comparative Physiology, 284(1), R39-R43.
[12] Aronne, L. J., et al. (2003). Pramlintide and sibutramine for weight loss in obese patients: A randomized controlled trial. Obesity Research, 11(2), 255-265.
[13] Jhamandas, J. H., et al. (2011). Amylin protects hippocampal neurons against extracellular beta-amyloid-induced toxicity. Journal of Neuroscience, 31(32), 11233-11239.
[14] Young, A. A., et al. (2014). Design strategy for human amylin analogue pramlintide to reduce self-aggregation and fibrillation. Peptides, 58, 1–11.
[15] Roth, J. D., et al. (2007). Activation of area postrema neurons by amylin: Role in satiety. American Journal of Physiology-Regulatory, Integrative and Comparative Physiology, 293(1), R194-R202.
So, basically, amylin is the body’s way of saying, “Hold on, liver, not so fast with that glucose dump!”? Suddenly, my pancreas feels like the responsible adult in the room. Wonder if it accepts performance bonuses?
That’s a fantastic analogy! And yes, it seems your pancreas deserves some recognition. Maybe a healthy, balanced meal as a reward? It’s amazing how amylin helps coordinate glucose control, and these insights are driving exciting advancements like amycretin. Perhaps more bonuses are coming!
Editor: MedTechNews.Uk
Thank you to our Sponsor Esdebe
So, amylin is basically the unsung hero of metabolic regulation? Does this mean we can expect amylin-based supplements at the local health store, promising to curb cravings and magically manage our waistlines? Asking for a friend, of course.
That’s a great question! While amylin itself isn’t available as an over-the-counter supplement due to its peptide nature, research into amylin analogs is definitely heating up! Amycretin combines an amylin receptor agonist with a GLP-1 receptor agonist. Keep an eye on clinical trials and future developments in this area!
Editor: MedTechNews.Uk
Thank you to our Sponsor Esdebe