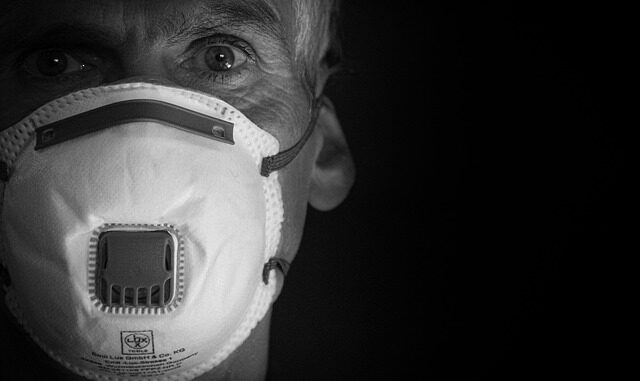
Abstract
Herd immunity, the indirect protection from infectious disease that arises when a sufficiently high proportion of a population is immune, either through prior infection or vaccination, remains a cornerstone of public health strategies. This report provides a comprehensive overview of herd immunity, extending beyond basic definitions to explore its nuanced theoretical underpinnings, the complexities of its practical application, and the ethical dilemmas it presents. We delve into the mathematical models used to estimate herd immunity thresholds, acknowledging their inherent limitations. Furthermore, we examine the dynamic interplay between disease characteristics, vaccination strategies, and societal behavior that influences the effectiveness of herd immunity. Finally, we address the contentious ethical considerations surrounding vaccination mandates and the challenges of balancing individual liberties with the collective good in the pursuit of herd immunity. This report aims to provide a critical perspective on herd immunity, relevant to experts in epidemiology, public health, and related fields.
Many thanks to our sponsor Esdebe who helped us prepare this research report.
1. Introduction: Defining and Contextualizing Herd Immunity
Herd immunity, also known as population immunity, is a phenomenon wherein the protection of susceptible individuals against infection arises indirectly from the presence of immune individuals within the population. It is not an individual-level property but rather an emergent population-level outcome. The underlying principle is that if a large enough proportion of the population is immune to a disease, the chain of transmission is disrupted, preventing widespread outbreaks and effectively shielding those who are not immune, whether due to age, underlying health conditions, or personal choice. This concept relies on the assumption that immune individuals are less likely to contract and transmit the disease. The immune individuals act as a barrier to the spread of the infection, effectively diluting the pool of susceptible hosts. Critically, herd immunity is not a perfect shield; breakthrough infections can and do occur, and pockets of susceptibility can persist, particularly in areas with low vaccination rates or among specific demographic groups.
The historical roots of herd immunity lie in the early observations of disease patterns. Edward Jenner’s pioneering work on vaccination against smallpox demonstrated that immunity could be artificially induced, and subsequent epidemiological studies revealed that vaccination campaigns could reduce the overall incidence of disease within a population. The concept was formalized mathematically in the 20th century, providing a framework for understanding the dynamics of infectious disease transmission and the potential for population-level protection. Modern research has expanded on these initial models, incorporating factors such as age structure, social mixing patterns, and the evolution of pathogens to provide a more realistic and nuanced picture of herd immunity dynamics.
The public health importance of herd immunity is undeniable. It serves as a crucial strategy for controlling and eliminating infectious diseases, particularly those for which vaccines are available. By achieving high levels of immunity within a population, it is possible to protect vulnerable individuals who cannot be vaccinated, such as infants, immunocompromised individuals, and those with allergies to vaccine components. Furthermore, herd immunity can reduce the overall burden of disease, alleviating strain on healthcare systems and minimizing the economic costs associated with outbreaks. However, the pursuit of herd immunity is not without its challenges, including vaccine hesitancy, logistical barriers to vaccination, and the ethical considerations surrounding vaccination mandates.
Many thanks to our sponsor Esdebe who helped us prepare this research report.
2. Mathematical Models of Herd Immunity: Thresholds and Limitations
The concept of a herd immunity threshold (HIT) is central to understanding the quantitative aspects of herd immunity. The HIT represents the minimum proportion of immune individuals required to prevent sustained transmission of a disease within a population. Below this threshold, each infected individual is expected to infect, on average, more than one other person, leading to continued spread. Above the HIT, each infected individual is expected to infect less than one other person, leading to a decline in the number of cases and eventually to elimination of the disease.
The HIT is typically calculated using the basic reproduction number (R0), which represents the average number of secondary infections caused by a single infected individual in a completely susceptible population. The HIT can be approximated by the formula: HIT = 1 – (1/R0). For example, if a disease has an R0 of 4, the HIT would be 75%, meaning that 75% of the population needs to be immune to achieve herd immunity. Different diseases have different R0 values, and thus different HITs. Measles, for instance, has a very high R0 (typically between 12 and 18), requiring a very high level of vaccination coverage to achieve herd immunity. In contrast, diseases with lower R0 values, such as influenza, require lower vaccination coverage.
While the HIT provides a useful theoretical framework, it is important to acknowledge its limitations. The formula relies on several simplifying assumptions, including:
- Homogeneous mixing: The model assumes that all individuals in the population have an equal chance of interacting with each other. In reality, populations are structured, with individuals tending to interact more frequently with others in their social networks, workplaces, or schools. This heterogeneity in contact patterns can significantly affect the effectiveness of herd immunity.
- Uniform immunity: The model assumes that immunity is uniformly distributed across the population. In reality, immunity levels may vary depending on age, geographic location, socioeconomic status, and access to healthcare. Pockets of susceptibility can persist even when overall vaccination coverage is high.
- Constant R0: The R0 is assumed to be constant over time. However, R0 can vary depending on factors such as seasonality, viral evolution, and changes in human behavior. For example, the R0 of influenza typically increases during the winter months.
- Perfect and lifelong immunity: The model often assumes that vaccination provides perfect and lifelong immunity. In reality, vaccines may not be 100% effective, and immunity may wane over time. This is particularly true for vaccines against rapidly evolving viruses, such as influenza.
More sophisticated mathematical models have been developed to address some of these limitations. These models incorporate factors such as age structure, social mixing patterns, spatial heterogeneity, and waning immunity. Agent-based models, for example, simulate the interactions of individual agents within a population, allowing for more realistic representation of contact patterns and transmission dynamics. These models can provide more accurate estimates of the HIT and can be used to evaluate the impact of different vaccination strategies.
Despite the advancements in mathematical modeling, it remains challenging to accurately predict the effectiveness of herd immunity in real-world settings. The complexity of human behavior and the unpredictable nature of viral evolution make it difficult to account for all the factors that influence disease transmission. Therefore, it is crucial to continuously monitor disease incidence and vaccination coverage to assess the effectiveness of herd immunity strategies and to adapt them as needed.
Many thanks to our sponsor Esdebe who helped us prepare this research report.
3. The Interplay of Disease Characteristics, Vaccination Strategies, and Societal Behavior
Herd immunity is not solely determined by the proportion of immune individuals in a population. It is a complex phenomenon that is influenced by a variety of factors, including the characteristics of the disease, the strategies used to implement vaccination programs, and the behaviors of individuals within the population.
The characteristics of the disease itself play a critical role. Diseases with high R0 values require higher levels of vaccination coverage to achieve herd immunity. The mode of transmission is also important. Diseases that are transmitted through the air, such as measles, are more difficult to control than diseases that are transmitted through direct contact. The severity of the disease also influences public perception and willingness to be vaccinated. Diseases that cause serious complications or death are more likely to motivate individuals to seek vaccination.
Vaccination strategies are also crucial. The type of vaccine used, the schedule of vaccination, and the delivery methods can all affect the effectiveness of vaccination programs. Vaccines that provide long-lasting immunity are more effective than vaccines that require frequent boosters. Vaccination schedules that are aligned with the age and risk factors of different populations can optimize the impact of vaccination programs. Delivery methods that are accessible and convenient can increase vaccination rates. Furthermore, the effectiveness of vaccination programs can be enhanced by public education campaigns that address vaccine hesitancy and promote the benefits of vaccination.
Societal behavior is perhaps the most challenging factor to address. Vaccine hesitancy, driven by misinformation, distrust of healthcare providers, or personal beliefs, can significantly reduce vaccination rates and undermine herd immunity. Complacency, arising from the perception that a disease is no longer a threat, can also lead to reduced vaccination rates. Cultural and social norms can also influence vaccination decisions. In some communities, vaccination may be viewed as a collective responsibility, while in others, it may be seen as a personal choice. Addressing vaccine hesitancy and promoting vaccination requires a multi-faceted approach that involves building trust, providing accurate information, and addressing the underlying concerns and beliefs of individuals.
The interaction between these factors is dynamic and complex. For example, a highly effective vaccine may not achieve its full potential if vaccination rates are low due to vaccine hesitancy. Similarly, a well-designed vaccination program may be undermined by the emergence of new viral strains that are resistant to the vaccine. Understanding these interactions is crucial for developing effective strategies to achieve and maintain herd immunity.
Many thanks to our sponsor Esdebe who helped us prepare this research report.
4. Ethical Considerations: Vaccination Mandates and the Balance of Individual Liberties
The pursuit of herd immunity often raises ethical questions, particularly concerning the use of vaccination mandates. Vaccination mandates require individuals to be vaccinated against certain diseases as a condition of enrollment in schools, employment, or access to public services. While mandates can be effective in increasing vaccination rates and achieving herd immunity, they also raise concerns about individual autonomy, freedom of choice, and bodily integrity.
The ethical justification for vaccination mandates is typically based on the principle of public health ethics known as the harm principle. This principle states that the government may restrict individual liberties if doing so is necessary to prevent harm to others. In the context of vaccination, the argument is that unvaccinated individuals pose a risk to others, particularly vulnerable populations who cannot be vaccinated or who are at higher risk of complications from infection. By requiring vaccination, the government can reduce the risk of disease transmission and protect the health of the community.
However, the harm principle is not without its limitations. Critics argue that it can be used to justify excessive restrictions on individual liberties. They argue that individuals have a right to make their own healthcare decisions, even if those decisions pose a risk to themselves or others. They also argue that the government should not force individuals to undergo medical procedures against their will.
In addition to the harm principle, other ethical considerations are relevant to the debate over vaccination mandates. These include:
- Beneficence: The principle of beneficence requires healthcare providers and public health officials to act in the best interests of their patients and the community. In the context of vaccination, beneficence supports the promotion of vaccination as a means of preventing disease and protecting health.
- Justice: The principle of justice requires that healthcare resources and benefits be distributed fairly across the population. In the context of vaccination, justice supports ensuring that all individuals have equal access to vaccines and that vaccination programs are designed to reduce health disparities.
- Respect for autonomy: The principle of respect for autonomy requires that individuals be allowed to make their own decisions about their healthcare. In the context of vaccination, respect for autonomy supports providing individuals with accurate information about the risks and benefits of vaccination and allowing them to make informed decisions.
Balancing these ethical principles is a complex task. Vaccination mandates can be justified when they are necessary to protect public health and when they are implemented in a fair and equitable manner. However, mandates should be carefully designed to minimize the infringement on individual liberties. Exemptions should be provided for individuals with legitimate medical contraindications to vaccination. Efforts should also be made to address vaccine hesitancy and to build trust in vaccination programs.
The ethical debate over vaccination mandates is ongoing and is likely to intensify as new vaccines are developed and as infectious diseases continue to pose a threat to public health. It is crucial to engage in open and respectful dialogue about these ethical issues and to find solutions that balance the rights and responsibilities of individuals with the need to protect the health of the community.
Many thanks to our sponsor Esdebe who helped us prepare this research report.
5. Modeling Scenarios: Disease Spread Under Different Vaccination Rates and Evolving Pathogens
Mathematical modeling plays a crucial role in understanding the potential impact of different vaccination rates on disease spread. Models can be used to simulate outbreaks under various scenarios, allowing public health officials to evaluate the effectiveness of different vaccination strategies and to predict the potential consequences of vaccine hesitancy or waning immunity.
One common modeling approach is to use compartmental models, such as the Susceptible-Infected-Recovered (SIR) model. In this model, the population is divided into three compartments: susceptible individuals, infected individuals, and recovered individuals. The model tracks the flow of individuals between these compartments over time, based on the rates of infection and recovery. By varying the vaccination rate in the model, it is possible to simulate the impact of different vaccination levels on the spread of the disease.
More sophisticated models can incorporate factors such as age structure, social mixing patterns, spatial heterogeneity, and waning immunity. These models can provide more realistic simulations of disease spread and can be used to evaluate the impact of different vaccination strategies on specific subpopulations.
Modeling can also be used to assess the potential impact of evolving pathogens on herd immunity. Viruses can evolve to become more transmissible, more virulent, or resistant to vaccines. These changes can alter the R0 of the disease and can undermine the effectiveness of herd immunity. Modeling can be used to predict how different viral mutations might affect disease spread and to evaluate the need for updated vaccines.
For example, models have been used to simulate the spread of influenza virus under different vaccination scenarios and with different levels of viral evolution. These models have shown that even with high vaccination rates, influenza can still cause significant outbreaks if the virus evolves to become resistant to the vaccine. This highlights the need for continuous monitoring of viral evolution and for the development of new vaccines that can keep pace with the evolving virus.
Similarly, models have been used to assess the potential impact of vaccine hesitancy on the spread of measles. These models have shown that even a small decline in vaccination rates can lead to a significant increase in the number of measles cases. This underscores the importance of maintaining high vaccination rates to prevent measles outbreaks.
The results of these modeling scenarios can inform public health policy and can help to guide vaccination strategies. By understanding the potential impact of different vaccination rates and the threat posed by evolving pathogens, public health officials can make informed decisions about how to best protect the health of the community.
Many thanks to our sponsor Esdebe who helped us prepare this research report.
6. Conclusion: Navigating the Future of Herd Immunity
Herd immunity remains a critical concept in public health, offering a pathway to control and eliminate infectious diseases. However, achieving and maintaining herd immunity is a complex and multifaceted challenge. It requires a deep understanding of disease dynamics, effective vaccination strategies, and a commitment to addressing vaccine hesitancy and promoting public trust.
The mathematical models used to estimate herd immunity thresholds provide a valuable framework for understanding the quantitative aspects of herd immunity. However, these models have limitations and should be used with caution. Real-world conditions are often more complex than the assumptions underlying these models.
The interplay of disease characteristics, vaccination strategies, and societal behavior is crucial in determining the effectiveness of herd immunity. Understanding these interactions is essential for developing effective strategies to achieve and maintain herd immunity.
The ethical considerations surrounding vaccination mandates must be carefully considered. Balancing individual liberties with the collective good is a complex and challenging task. Mandates should be used judiciously and should be implemented in a fair and equitable manner.
Modeling scenarios can provide valuable insights into the potential impact of different vaccination rates and the threat posed by evolving pathogens. These models can inform public health policy and can help to guide vaccination strategies.
Looking ahead, the future of herd immunity will depend on several factors, including:
- The development of new and improved vaccines: Vaccines that provide long-lasting immunity and that are effective against a broad range of viral strains will be essential for achieving and maintaining herd immunity.
- Improved understanding of vaccine hesitancy: Addressing vaccine hesitancy requires a multi-faceted approach that involves building trust, providing accurate information, and addressing the underlying concerns and beliefs of individuals.
- Enhanced surveillance of infectious diseases: Continuous monitoring of disease incidence and vaccination coverage is crucial for assessing the effectiveness of herd immunity strategies and for detecting emerging threats.
- Strengthened international collaboration: Infectious diseases do not respect national borders. International collaboration is essential for controlling and eliminating infectious diseases and for ensuring that vaccines are available to all who need them.
By addressing these challenges and by working together, we can harness the power of herd immunity to protect the health of our communities and to create a healthier future for all.
Many thanks to our sponsor Esdebe who helped us prepare this research report.
References
- Anderson, R. M., & May, R. M. (1991). Infectious diseases of humans: dynamics and control. Oxford University Press.
- Fine, P., Eames, K., & Heymann, D. L. (2011). “Herd immunity”: a rough guide. Clinical Infectious Diseases, 52(7), 911-916.
- Omer, S. B., Salmon, D. A., Orenstein, W. A., deHart, M. P., & Halsey, N. (2009). Vaccine refusal, mandatory immunization, and the risks of vaccine-preventable diseases. New England Journal of Medicine, 360(19), 1981-1988.
- Poland, G. A., & Jacobson, R. M. (2011). The age of reason: vaccine hesitancy and the mature parent. Pediatrics, 128(Supplement 3), S127-S134.
- Sirota, M., Juanchich, M., & Bonnefon, J. F. (2021). Herd immunity as a coordination game. Philosophical Transactions of the Royal Society B, 376(1829), 20200337.
- World Health Organization. (2024). Immunization coverage. Retrieved from https://www.who.int/news-room/fact-sheets/detail/immunization-coverage
- Doshi, P. (2020). Covid-19: Do many people have pre-existing immunity?. BMJ, 371, m4850.
- Lipsitch, M., & Goldstein, E. (2019). Herd immunity and its discontents. Vaccine, 37(48), 7115-7121.
- Keeling, M. J., & Rohani, P. (2008). Modeling infectious diseases in humans and animals. Princeton University Press.
Be the first to comment