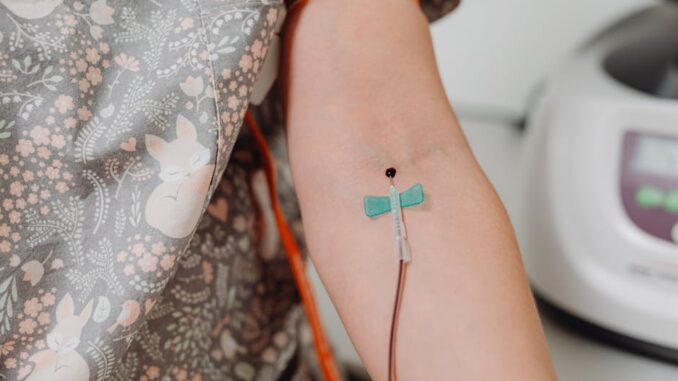
A Comprehensive Review of Catheter Technology: Materials, Failure Mechanisms, and Future Directions
Abstract
Catheters represent a ubiquitous class of medical devices critical for a wide range of diagnostic and therapeutic procedures. While their use is often routine, the potential for device failure, particularly leakage due to material fatigue, poses significant clinical risks. This research report provides a comprehensive overview of catheter technology, encompassing diverse catheter types, the materials employed in their construction, common failure modes, manufacturing processes impacting durability, and the regulatory landscape governing their safety and performance. Furthermore, the report explores recent advancements in catheter design, materials science, and manufacturing techniques, focusing on strategies to enhance reliability, minimize complications, and ultimately improve patient outcomes. Special attention is given to the interplay between material selection, device geometry, and applied stresses, with a focus on predicting and mitigating fatigue-related failures.
1. Introduction
Catheters are indispensable medical devices introduced into the body to deliver or remove fluids, provide access for diagnostic procedures, or perform therapeutic interventions. Their applications span nearly every medical specialty, including cardiology, urology, vascular surgery, anesthesiology, and oncology. Given their invasive nature and prolonged contact with bodily fluids and tissues, catheter safety and reliability are of paramount importance. A failure in catheter functionality can lead to serious complications, including infection, thrombosis, hemorrhage, and even death. One recurring concern is catheter leakage, often stemming from material fatigue, particularly in flexible catheters subjected to repetitive bending and flexing during insertion, dwell time, and removal. This report aims to provide a detailed examination of the multifaceted aspects of catheter technology, emphasizing the critical factors influencing device performance and longevity.
2. Catheter Types and Applications
Catheters are categorized based on their intended use, insertion route, and design features. Several key classifications include:
- Peripheral Intravenous Catheters (PIVCs): Used for short-term intravenous access for fluid and medication administration.
- Central Venous Catheters (CVCs): Inserted into large veins (e.g., subclavian, jugular, femoral) to provide long-term intravenous access for medication, fluid, and nutritional support. Subcategories include tunneled catheters (e.g., Hickman, Broviac), non-tunneled catheters, and peripherally inserted central catheters (PICCs).
- Urinary Catheters: Used to drain urine from the bladder. Types include indwelling catheters (e.g., Foley catheters) and intermittent catheters.
- Angiographic Catheters: Used to access blood vessels for angiography, angioplasty, and other interventional procedures.
- Cardiac Catheters: Used to access the heart chambers and coronary arteries for diagnostic and therapeutic procedures.
- Neurological Catheters: Used for cerebrospinal fluid drainage or intracranial pressure monitoring.
The specific material requirements and design considerations vary significantly depending on the catheter type and its intended application. For instance, cardiac catheters require excellent flexibility and trackability to navigate tortuous blood vessels, while urinary catheters must be biocompatible and resistant to encrustation.
3. Materials Used in Catheter Manufacturing
The selection of materials for catheter construction is a critical factor influencing device performance, biocompatibility, and durability. Common materials include:
- Polymers:
- Polyurethane (PU): Widely used due to its flexibility, biocompatibility, and resistance to degradation. However, some formulations are susceptible to hydrolysis and oxidation.
- Silicone: Known for its excellent biocompatibility and flexibility. However, silicone can be prone to kinking and may exhibit lower tensile strength than polyurethane.
- Polyethylene (PE): Used for stiff catheters or catheter components requiring high tensile strength.
- Polyvinyl Chloride (PVC): Historically used, but less common now due to concerns about phthalate plasticizers and environmental impact. Still used in some low-cost applications.
- Polytetrafluoroethylene (PTFE) (Teflon): Used for its low friction surface, making it ideal for coatings to improve catheter trackability.
- Nylon (Polyamide): Used for its high tensile strength and abrasion resistance.
- Metals:
- Stainless Steel: Used for guidewires and catheter shafts requiring high stiffness and radiopacity.
- Nitinol (Nickel-Titanium Alloy): Used for self-expanding stents and catheter components requiring shape memory properties and superelasticity.
- Other Materials:
- Radiopaque Fillers: Barium sulfate, tungsten, and bismuth trioxide are added to polymers to enhance radiopacity for visualization under fluoroscopy.
- Hydrophilic Coatings: Applied to the catheter surface to reduce friction and improve trackability. Examples include polyvinylpyrrolidone (PVP) and hyaluronic acid.
4. Common Catheter Failure Modes
Catheter failure can manifest in various ways, leading to significant patient complications. Common failure modes include:
- Leakage: Occurs due to cracks, punctures, or degradation of the catheter material. As previously mentioned, material fatigue is a major contributor to leakage, especially in flexible catheters subjected to repetitive bending and flexing.
- Kinking: Occurs when the catheter collapses or bends sharply, obstructing flow. Kinking is more common in flexible catheters with insufficient structural support.
- Fracture: Occurs when the catheter breaks into pieces, potentially requiring surgical removal.
- Thrombosis: Formation of blood clots within the catheter lumen or on the catheter surface, obstructing flow and potentially leading to thromboembolic events.
- Infection: Colonization of the catheter surface with microorganisms, leading to bloodstream infections (BSIs) or local infections.
- Material Degradation: Chemical or physical changes in the catheter material over time, leading to loss of mechanical strength or biocompatibility. Degradation can be caused by hydrolysis, oxidation, enzymatic attack, or exposure to radiation.
- Delamination: Separation of layers in multi-layered catheters.
Understanding the underlying mechanisms of these failure modes is crucial for developing strategies to improve catheter design and material selection.
5. Manufacturing Processes and Their Impact on Durability
The manufacturing processes employed in catheter production significantly influence the device’s durability and performance. Key processes include:
- Extrusion: Used to create catheter tubing with precise dimensions and consistent wall thickness. Extrusion parameters, such as temperature, pressure, and die design, must be carefully controlled to ensure material homogeneity and minimize defects.
- Injection Molding: Used to create catheter hubs, connectors, and other complex components. Injection molding allows for the production of intricate shapes with high precision.
- Bonding: Used to join different catheter components together. Bonding techniques include adhesive bonding, thermal bonding, and solvent bonding. The strength and durability of the bond are critical for preventing leakage and component separation.
- Coating: Used to apply hydrophilic coatings, antimicrobial coatings, or drug-eluting coatings to the catheter surface. Coating uniformity and adhesion are critical for ensuring consistent performance.
- Sterilization: Used to eliminate microorganisms from the catheter surface. Sterilization methods include ethylene oxide (EtO) sterilization, gamma irradiation, and autoclaving. The choice of sterilization method must be compatible with the catheter material and must not compromise its mechanical properties.
Quality control measures throughout the manufacturing process are essential for detecting and eliminating defects that could compromise catheter durability.
6. Regulatory Frameworks Governing Catheter Safety and Performance
Catheter safety and performance are regulated by government agencies worldwide. In the United States, the Food and Drug Administration (FDA) regulates catheters as medical devices. The FDA classifies catheters into different classes based on their risk profile. Class III devices, which pose the highest risk, require premarket approval (PMA) before they can be marketed. This process involves rigorous testing and clinical trials to demonstrate the device’s safety and effectiveness.
The European Union (EU) regulates catheters under the Medical Device Regulation (MDR). The MDR requires manufacturers to demonstrate conformity with essential requirements related to safety and performance. This is often achieved through certification by a notified body.
Other countries have their own regulatory frameworks for medical devices. The regulatory landscape is constantly evolving, and manufacturers must stay abreast of the latest requirements to ensure compliance.
7. Advancements in Catheter Design and Materials
Ongoing research and development efforts are focused on improving catheter design and materials to enhance reliability, minimize complications, and improve patient outcomes. Key advancements include:
- Next-Generation Polymers: Development of new polymers with improved biocompatibility, mechanical strength, and resistance to degradation. Examples include bioabsorbable polymers and polymers with antimicrobial properties.
- Shape Memory Alloys: Use of nitinol and other shape memory alloys to create catheters that can navigate complex anatomical structures with minimal trauma.
- Micro- and Nano-Structured Surfaces: Engineering of catheter surfaces with micro- or nano-scale features to reduce friction, prevent thrombosis, and inhibit bacterial adhesion.
- Drug-Eluting Catheters: Coating catheters with drugs to prevent restenosis, infection, or inflammation.
- 3D Printing: Use of 3D printing to create custom catheters tailored to individual patient anatomy.
- Advanced Manufacturing Techniques: Development of more precise and controlled manufacturing processes to minimize defects and improve catheter durability. Examples include laser micromachining and micro-injection molding.
- Biomimicry: Designing catheters that mimic biological systems to improve biocompatibility and reduce the risk of adverse reactions.
8. Addressing Material Fatigue and Leakage in BD PowerPICC Catheters
As highlighted in the prompt, BD PowerPICC catheters and related issues of material fatigue leading to leaks, serve as a specific example of a broader concern. To address these issues and similar problems in other catheter types, several strategies can be employed. Finite element analysis (FEA) can be used to model the stress distribution within the catheter during use, identifying areas of high stress concentration that are prone to fatigue failure. This allows for optimization of catheter geometry to reduce stress levels. Material testing, including accelerated aging studies and fatigue testing, can be used to evaluate the long-term durability of the catheter material under simulated use conditions. These tests should simulate the stresses and strains experienced by the catheter during insertion, dwell time, and removal. Furthermore, implementing robust quality control measures during manufacturing is paramount. This includes rigorous inspection of raw materials, monitoring of extrusion and molding processes, and performance testing of finished devices.
Addressing the specific issues related to BD PowerPICC catheters also necessitates a thorough investigation of reported failure modes. This would involve analyzing returned devices to determine the root cause of leakage, whether it is material fatigue, manufacturing defects, or improper handling. The data collected from these investigations can be used to inform design modifications and improve manufacturing processes.
9. Future Directions
The future of catheter technology lies in the development of more biocompatible, durable, and intelligent devices. Key areas of focus include:
- Personalized Catheters: Creating catheters that are tailored to individual patient needs based on their anatomy, medical history, and risk factors.
- Smart Catheters: Developing catheters with integrated sensors and actuators that can monitor physiological parameters, deliver drugs on demand, or perform minimally invasive procedures.
- Remote Monitoring: Implementing remote monitoring systems to track catheter performance and detect potential complications early on.
- AI-Driven Design: Using artificial intelligence and machine learning to optimize catheter design and material selection based on large datasets of clinical outcomes.
- Sustainability: Developing more sustainable catheter materials and manufacturing processes to reduce environmental impact.
10. Conclusion
Catheters are essential medical devices that play a critical role in modern healthcare. However, their safety and reliability are paramount. Material fatigue, leading to leakage, remains a significant concern. This report has provided a comprehensive overview of catheter technology, encompassing diverse catheter types, materials, failure modes, manufacturing processes, and regulatory frameworks. Furthermore, the report has explored recent advancements in catheter design and materials aimed at improving reliability and reducing risks. By addressing the challenges associated with catheter technology and embracing innovation, we can develop safer, more effective, and more sustainable devices that improve patient outcomes and enhance the quality of healthcare.
References
- Anderson, J. M., & Hanson, S. R. (2008). Advancing medical device biocompatibility. Science Translational Medicine, 1(1), 1ra1.
- Ratner, B. D., Bryant, S. J. (2004). Biomaterials: Where we have been and where we are going. Annual Review of Biomedical Engineering, 6, 41-75.
- Langer, R., & Tirrell, D. A. (2004). Designing materials for biology and medicine. Nature, 428(6982), 487-492.
- Medical Device Regulation (MDR) (EU) 2017/745.
- U.S. Food and Drug Administration (FDA) website: https://www.fda.gov/
- Dumoulin, C., & Vleugels, J. (2010). Central venous catheters: mechanical and infectious complications. Journal of Vascular Access, 11(3), 153-164.
- Mermel, L. A. (2000). Prevention of intravascular catheter-related infections. Annals of Internal Medicine, 132(5), 391-402.
- Pittiruti, M., Hamilton, H., Biffi, R., MacFarlane, C., Pertkiewicz, M. (2009). European recommendations on the use of central venous access devices (CVADs) in adult oncology patients: a DELPHI consensus survey. Supportive Care in Cancer, 17(6), 677-690.
- Webster, J., Osborne, S., Rickard, C. M., & Marsh, N. (2015). Clinically-indicated replacement versus routine replacement of peripheral intravenous catheters. Cochrane Database of Systematic Reviews, 8, CD007798.
- National Healthcare Safety Network (NHSN). (n.d.). Catheter-Associated Bloodstream Infection (CLABSI) Event. Centers for Disease Control and Prevention.
- Bryers, J. D. (2008). Medical device biofilms. Biomaterials, 29(34), 4281-4303.
This is a thorough review. Given the increasing use of catheters in outpatient settings, could remote monitoring technologies, as mentioned in the “Future Directions” section, significantly reduce complications by enabling earlier detection of issues like obstructions or infections?