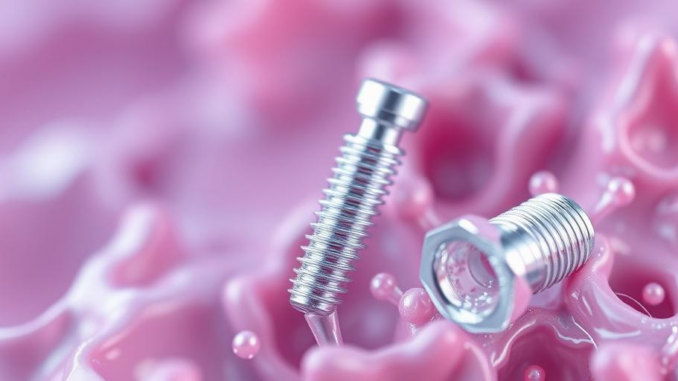
A Comprehensive Review of Medical Implants: Materials, Failure Modes, Regulation, and Future Directions
Abstract
Medical implants have revolutionized healthcare, offering solutions for a wide range of conditions, from cardiovascular diseases to orthopedic injuries and neurological disorders. This review provides a comprehensive overview of medical implants, covering various types, the materials used in their construction, common failure modes, regulatory oversight mechanisms, and recent advancements aimed at enhancing biocompatibility and longevity. We explore the critical role of materials science in implant development, examining the properties of metals, polymers, ceramics, and composites used in different implant applications. A detailed analysis of failure modes, including mechanical wear, corrosion, infection, and biocompatibility issues, is presented, emphasizing the importance of robust design and manufacturing processes. Furthermore, we delve into the complex regulatory landscape governing medical implants, focusing on the roles of agencies like the FDA and EMA in ensuring patient safety and device efficacy. Finally, we discuss emerging technologies and research directions, such as advanced biomaterials, 3D printing, and smart implants, which hold promise for improving implant performance and patient outcomes. This review aims to provide a valuable resource for researchers, clinicians, and industry professionals involved in the design, development, and clinical application of medical implants.
1. Introduction
Medical implants are devices or tissues that are placed inside or on the surface of the body. They are designed to replace, support, or enhance biological functions. The history of medical implants dates back centuries, with early examples including wooden prosthetics and crude dental implants. However, the modern era of medical implants began in the 20th century with the development of biocompatible materials and advanced surgical techniques. Today, a vast array of medical implants is available, addressing diverse medical needs and improving the quality of life for millions of patients worldwide.
The scope of medical implants is remarkably broad, encompassing devices used in cardiology (e.g., pacemakers, stents, artificial heart valves), orthopedics (e.g., hip and knee replacements, spinal implants), neurology (e.g., deep brain stimulators, vagus nerve stimulators), ophthalmology (e.g., intraocular lenses), and numerous other specialties. These devices can be made from a variety of materials, including metals (e.g., titanium, stainless steel, cobalt-chromium alloys), polymers (e.g., polyethylene, silicone), ceramics (e.g., alumina, zirconia), and composites. The selection of materials depends on the specific application, required mechanical properties, biocompatibility considerations, and manufacturing feasibility.
While medical implants offer significant benefits, they are also associated with potential risks and complications. Implant failure, infection, biocompatibility issues, and regulatory challenges are major concerns. Recent events, such as recalls of implantable ports and concerns surrounding breast implants, have highlighted the importance of rigorous testing, quality control, and post-market surveillance. Therefore, a comprehensive understanding of medical implants, including their design, materials, failure modes, regulatory aspects, and future directions, is essential for ensuring their safe and effective use.
2. Types of Medical Implants
Medical implants can be classified based on their function, location within the body, and the materials used in their construction. This section provides an overview of several major categories of medical implants.
2.1 Cardiovascular Implants
Cardiovascular implants are designed to treat diseases and conditions affecting the heart and blood vessels. Common types of cardiovascular implants include:
-
Pacemakers: These devices regulate heart rhythm in patients with bradycardia (slow heart rate) or arrhythmias. Modern pacemakers are sophisticated electronic devices that can sense the heart’s electrical activity and deliver precisely timed electrical impulses to stimulate contraction. Improvements in battery life and miniaturization have significantly enhanced the performance and longevity of pacemakers.
-
Implantable Cardioverter-Defibrillators (ICDs): ICDs are used to treat life-threatening ventricular arrhythmias, such as ventricular tachycardia and ventricular fibrillation. They can deliver high-energy shocks to restore normal heart rhythm. ICDs are often combined with pacemakers to provide comprehensive cardiac rhythm management.
-
Stents: Stents are small, expandable tubes that are inserted into narrowed or blocked arteries to restore blood flow. They are commonly used to treat coronary artery disease. Drug-eluting stents release medication to prevent restenosis (re-narrowing of the artery). Advancements in stent design and drug delivery systems have significantly improved their effectiveness and safety.
-
Artificial Heart Valves: Artificial heart valves are used to replace diseased or damaged heart valves. They can be mechanical or bioprosthetic (made from animal tissue). Mechanical valves are durable but require lifelong anticoagulation therapy, while bioprosthetic valves have a limited lifespan but do not usually require anticoagulation.
-
Ventricular Assist Devices (VADs): VADs are mechanical pumps that assist the heart in pumping blood. They are used as a bridge to transplant or as a destination therapy for patients with severe heart failure. VADs have significantly improved survival rates for patients with end-stage heart failure.
2.2 Orthopedic Implants
Orthopedic implants are used to treat fractures, joint diseases, and other musculoskeletal conditions. Common types of orthopedic implants include:
-
Hip and Knee Replacements: These implants replace damaged hip or knee joints with artificial components. They are used to relieve pain and improve mobility in patients with osteoarthritis, rheumatoid arthritis, or traumatic injuries. Modern hip and knee replacements are designed for long-term durability and functionality.
-
Spinal Implants: Spinal implants are used to stabilize the spine after fractures, spinal fusions, or other surgical procedures. They can include screws, rods, plates, and interbody cages. Advancements in spinal implant design have led to improved fusion rates and reduced complications.
-
Fracture Fixation Devices: These implants are used to stabilize broken bones during the healing process. They can include plates, screws, rods, and external fixators. The choice of fixation device depends on the type and location of the fracture.
-
Bone Graft Substitutes: Bone graft substitutes are used to promote bone healing in fractures or spinal fusions. They can be made from synthetic materials or processed bone tissue. These substitutes provide a scaffold for new bone formation and can accelerate the healing process.
2.3 Neurological Implants
Neurological implants are used to treat a variety of neurological disorders, including Parkinson’s disease, epilepsy, and chronic pain. Common types of neurological implants include:
-
Deep Brain Stimulators (DBS): DBS devices deliver electrical stimulation to specific areas of the brain to alleviate symptoms of Parkinson’s disease, essential tremor, and dystonia. DBS has revolutionized the treatment of these movement disorders.
-
Vagus Nerve Stimulators (VNS): VNS devices stimulate the vagus nerve to reduce the frequency of seizures in patients with epilepsy and to treat depression. VNS is a non-pharmacological alternative for patients who do not respond to medication.
-
Spinal Cord Stimulators (SCS): SCS devices deliver electrical stimulation to the spinal cord to relieve chronic pain. SCS is used to treat neuropathic pain, back pain, and complex regional pain syndrome.
-
Intrathecal Drug Delivery Systems: These systems deliver medication directly to the spinal cord to manage chronic pain or spasticity. They can provide targeted drug delivery with minimal systemic side effects.
2.4 Other Types of Implants
In addition to the major categories listed above, there are numerous other types of medical implants used in various specialties, including:
-
Dental Implants: These implants replace missing teeth and provide a stable foundation for dentures or crowns.
-
Breast Implants: These implants are used for breast augmentation or reconstruction after mastectomy.
-
Ocular Implants: These implants are used to treat glaucoma, cataracts, and other eye conditions.
-
Auditory Implants: These implants, such as cochlear implants, restore hearing in patients with severe hearing loss.
-
Drug Delivery Implants: These implants release medication at a controlled rate over an extended period of time.
3. Materials Used in Medical Implants
The selection of materials is crucial in the design and development of medical implants. The materials must be biocompatible, mechanically strong, corrosion-resistant, and able to withstand the harsh environment of the human body. This section discusses the major classes of materials used in medical implants.
3.1 Metals and Alloys
Metals and alloys are widely used in medical implants due to their high strength, durability, and biocompatibility. Common metallic implant materials include:
-
Titanium and Titanium Alloys: Titanium and its alloys, such as Ti-6Al-4V, are highly biocompatible and corrosion-resistant. They are used in orthopedic implants, dental implants, and cardiovascular implants.
-
Stainless Steel: Stainless steel is a widely used metallic implant material due to its high strength and corrosion resistance. It is used in orthopedic implants, fracture fixation devices, and cardiovascular implants.
-
Cobalt-Chromium Alloys: Cobalt-chromium alloys are known for their high wear resistance and biocompatibility. They are used in hip and knee replacements, dental implants, and cardiovascular stents.
-
Shape Memory Alloys (e.g., Nitinol): Shape memory alloys, such as Nitinol (nickel-titanium alloy), can return to a pre-defined shape after being deformed. They are used in cardiovascular stents and orthopedic implants.
3.2 Polymers
Polymers are used in medical implants due to their versatility, biocompatibility, and ease of processing. Common polymeric implant materials include:
-
Polyethylene (PE): Polyethylene is a widely used polymer in orthopedic implants, particularly in hip and knee replacements. High-density polyethylene (HDPE) and ultra-high molecular weight polyethylene (UHMWPE) are commonly used.
-
Polymethylmethacrylate (PMMA): PMMA is used as bone cement to fix orthopedic implants in place. It is also used in intraocular lenses and dental implants.
-
Silicone: Silicone is a biocompatible polymer used in breast implants, catheters, and other medical devices. It is known for its flexibility and chemical inertness.
-
Polyurethane (PU): Polyurethane is used in cardiovascular implants, such as heart valves and VADs. It is known for its flexibility and blood compatibility.
-
Biodegradable Polymers: Biodegradable polymers, such as polylactic acid (PLA) and polyglycolic acid (PGA), are used in sutures, drug delivery systems, and tissue engineering scaffolds. They degrade over time, eliminating the need for removal.
3.3 Ceramics
Ceramics are used in medical implants due to their high biocompatibility, wear resistance, and compressive strength. Common ceramic implant materials include:
-
Alumina (Aluminum Oxide): Alumina is used in hip replacements and dental implants due to its high wear resistance and biocompatibility.
-
Zirconia (Zirconium Oxide): Zirconia is used in hip replacements and dental implants due to its high strength and fracture toughness.
-
Hydroxyapatite (HA): Hydroxyapatite is a calcium phosphate ceramic that is similar to the mineral component of bone. It is used as a coating for orthopedic implants to promote bone ingrowth.
-
Bioactive Glasses: Bioactive glasses are silicate-based ceramics that can bond directly to bone tissue. They are used in bone grafts and dental implants.
3.4 Composites
Composites are materials made from two or more components with different physical and chemical properties. They are used in medical implants to combine the desirable properties of different materials. Common composite implant materials include:
-
Carbon Fiber Reinforced Polymers (CFRP): CFRP is used in orthopedic implants and spinal implants due to its high strength-to-weight ratio and biocompatibility.
-
Polymer-Ceramic Composites: These composites combine the strength and biocompatibility of polymers and ceramics. They are used in bone grafts and dental implants.
4. Common Failure Modes of Medical Implants
Medical implants, despite being designed and manufactured to high standards, are susceptible to failure. Understanding the common failure modes is crucial for improving implant design, material selection, and surgical techniques. This section discusses the major failure modes of medical implants.
4.1 Mechanical Wear
Mechanical wear is a common failure mode in orthopedic implants, particularly in hip and knee replacements. It occurs when the articulating surfaces of the implant rub against each other, leading to the generation of wear particles. These wear particles can cause inflammation and osteolysis (bone resorption), leading to implant loosening and failure. Factors that contribute to mechanical wear include implant design, material properties, surgical technique, and patient activity level.
4.2 Corrosion
Corrosion is a major concern for metallic implants. The body’s physiological environment is highly corrosive, with the presence of electrolytes, proteins, and immune cells that can accelerate the corrosion process. Corrosion can lead to the release of metal ions into the surrounding tissues, causing inflammation, allergic reactions, and even systemic toxicity. Factors that influence corrosion include the type of metal or alloy, surface finish, and the presence of stress or defects in the implant.
4.3 Infection
Implant-related infections are a serious complication that can lead to implant failure and significant morbidity. Bacteria can adhere to the implant surface and form biofilms, which are resistant to antibiotics and immune cells. Implant infections are often difficult to treat and may require surgical removal of the implant. Factors that increase the risk of infection include surgical technique, patient health, and the presence of foreign bodies.
4.4 Biocompatibility Issues
Biocompatibility refers to the ability of a material to interact with the body without causing adverse reactions. Biocompatibility issues can arise from the release of toxic substances from the implant material, inflammation, allergic reactions, or the formation of scar tissue around the implant. Factors that influence biocompatibility include the chemical composition of the material, surface properties, and the presence of contaminants.
4.5 Fracture
Implant fracture is a catastrophic failure mode that can occur due to excessive loading, material defects, or corrosion. Implant fractures can lead to pain, instability, and the need for revision surgery. Factors that increase the risk of fracture include implant design, material properties, surgical technique, and patient activity level.
4.6 Loosening
Implant loosening is a common failure mode in orthopedic and dental implants. It occurs when the implant loses its fixation to the surrounding bone. Loosening can be caused by mechanical wear, corrosion, infection, or biocompatibility issues. Implant loosening can lead to pain, instability, and the need for revision surgery.
5. Regulatory Oversight
Medical implants are subject to stringent regulatory oversight to ensure their safety and efficacy. The regulatory framework varies by country, but the general principles are similar. This section discusses the regulatory oversight of medical implants in the United States and Europe.
5.1 United States (FDA)
In the United States, medical implants are regulated by the Food and Drug Administration (FDA). The FDA classifies medical devices into three classes based on the level of risk they pose to patients:
-
Class I: Low-risk devices, such as bandages and examination gloves, are subject to general controls, such as registration and labeling requirements.
-
Class II: Moderate-risk devices, such as surgical instruments and powered wheelchairs, are subject to special controls, such as performance standards and premarket notification (510(k)).
-
Class III: High-risk devices, such as implantable pacemakers and heart valves, are subject to premarket approval (PMA), which requires extensive clinical data to demonstrate safety and efficacy.
The FDA also has post-market surveillance programs to monitor the performance of medical devices after they have been approved for sale. These programs include adverse event reporting, device tracking, and recalls. The FDA has the authority to recall medical devices that are found to be unsafe or ineffective.
5.2 Europe (EMA)
In Europe, medical implants are regulated by the European Medicines Agency (EMA) and national regulatory authorities. The European Union Medical Device Regulation (MDR) sets the requirements for medical devices sold in Europe. The MDR classifies medical devices into four classes based on the level of risk they pose to patients:
-
Class I: Low-risk devices, such as bandages and examination gloves, are subject to general requirements.
-
Class IIa: Moderate-risk devices, such as surgical instruments and hearing aids, are subject to conformity assessment by a notified body.
-
Class IIb: Higher moderate-risk devices, such as bone grafts and infusion pumps, are subject to more stringent conformity assessment by a notified body.
-
Class III: High-risk devices, such as implantable pacemakers and heart valves, are subject to the most stringent conformity assessment by a notified body.
Notified bodies are independent organizations that are authorized to assess the conformity of medical devices with the requirements of the MDR. The EMA also has post-market surveillance programs to monitor the performance of medical devices after they have been placed on the market. These programs include adverse event reporting and recalls.
5.3 The impact of faulty manufacturing
Faulty manufacturing of medical implants can have devastating consequences for patients. Substandard materials, inadequate quality control, and errors in the manufacturing process can lead to implant failure, infection, biocompatibility issues, and other serious complications. The financial impact on patients and the healthcare system can be significant, including the costs of revision surgery, medical treatment, and lost productivity. Beyond the immediate physical and economic toll, faulty manufacturing erodes patient trust in the medical device industry and regulatory bodies. This can lead to a reluctance to undergo necessary procedures or participate in clinical trials, potentially hindering medical advancements.
In response to instances of faulty manufacturing, regulatory bodies such as the FDA and EMA have increased their scrutiny of manufacturing processes, demanding more robust quality control systems and audits. Additionally, stricter penalties for manufacturers who fail to meet quality standards are being implemented to deter negligence and prioritize patient safety.
6. Advancements in Implant Technology
Medical implant technology is constantly evolving, with ongoing research and development efforts focused on improving biocompatibility, longevity, and functionality. This section discusses some of the recent advancements in implant technology.
6.1 Advanced Biomaterials
Research is ongoing to develop new biomaterials with improved properties, such as enhanced biocompatibility, corrosion resistance, and mechanical strength. Examples of advanced biomaterials include:
-
Surface-Modified Materials: Surface modification techniques, such as plasma spraying, ion implantation, and chemical vapor deposition, are used to improve the biocompatibility and corrosion resistance of implant materials. These techniques can create a thin layer of bioactive material on the implant surface, promoting bone ingrowth or reducing inflammation.
-
Nanomaterials: Nanomaterials, such as nanoparticles and nanofibers, have unique properties that can be used to improve implant performance. For example, nanoparticles can be incorporated into implant coatings to enhance drug delivery or antimicrobial activity. Nanofibers can be used to create scaffolds for tissue engineering.
-
Bioinspired Materials: Bioinspired materials are designed to mimic the structure and properties of natural tissues. For example, bone-like materials can be created by incorporating calcium phosphate into a polymer matrix. These materials can promote bone ingrowth and integration with the surrounding tissue.
6.2 3D Printing (Additive Manufacturing)
3D printing, also known as additive manufacturing, is a revolutionary technology that allows for the creation of complex three-dimensional objects from digital designs. 3D printing is being used to create customized medical implants that are tailored to the individual patient’s anatomy. This technology can improve implant fit, reduce surgical time, and enhance patient outcomes. 3D printing is also being used to create scaffolds for tissue engineering and drug delivery systems.
6.3 Smart Implants
Smart implants are medical devices that incorporate sensors, actuators, and communication systems to monitor implant performance and provide feedback to the patient or clinician. Smart implants can be used to monitor implant temperature, pressure, strain, and other parameters. This information can be used to detect early signs of implant failure or infection. Smart implants can also be used to deliver medication or electrical stimulation to the surrounding tissue.
6.4 Minimally Invasive Implantation Techniques
Minimally invasive surgical techniques are being developed to reduce the trauma associated with implant surgery. These techniques involve the use of small incisions and specialized instruments to place the implant. Minimally invasive surgery can reduce pain, scarring, and recovery time.
7. Conclusion
Medical implants have transformed healthcare, providing effective solutions for a wide range of medical conditions. The development and application of medical implants require a multidisciplinary approach, involving materials science, engineering, surgery, and regulatory affairs. While medical implants offer significant benefits, they are also associated with potential risks and complications. Therefore, ongoing research and development efforts are essential to improve implant design, materials, manufacturing processes, and surgical techniques. Advancements in biomaterials, 3D printing, and smart implants hold promise for enhancing implant performance and patient outcomes. Furthermore, rigorous regulatory oversight is crucial to ensure the safety and efficacy of medical implants. By addressing these challenges and embracing innovation, we can continue to improve the lives of patients who rely on medical implants.
References
- Anderson, J. M. (2001). Biological responses to materials. Annual Review of Materials Research, 31(1), 81-110.
- Ratner, B. D., Hoffman, A. S., Schoen, F. J., & Lemons, J. E. (2013). Biomaterials science: An introduction to materials in medicine. Academic press.
- Black, J. (2015). Biological performance of materials: Fundamentals of biocompatibility. CRC press.
- Langer, R., & Tirrell, D. A. (2004). Designing materials for biology and medicine. Nature, 428(6982), 487-492.
- Disegi, J. A., Eschbach, L., & Craig, B. (2009). Medical device regulatory pathways in the United States. Journal of Bone and Joint Surgery, 91(Suppl 3), 8-13.
- U.S. Food and Drug Administration. (n.d.). Medical devices. Retrieved from https://www.fda.gov/medical-devices
- European Medicines Agency. (n.d.). Medical devices. Retrieved from https://www.ema.europa.eu/en/human-regulatory/overview/medical-devices
- Williams, D. F. (2008). On the mechanisms of biocompatibility. Biomaterials, 29(20), 2941-2953.
- Hollander, J. E., & Kirk, J. D. (2006). Contamination in medical device manufacturing. Medical Device and Diagnostic Industry.
- Bose, S., Roy, M., & Bandyopadhyay, A. (2013). Recent advances in bone scaffold fabrication via additive manufacturing techniques. Trends in Biotechnology, 31(5), 295-304.
- Chia, H. N., & Wu, B. M. (2015). Recent advances in 3D printing of biomaterials. Journal of Biological Engineering, 9(1), 4.
- Kim, D. H., & Kim, P. (2018). Smart implantable medical devices: Recent advances and future perspectives. Micromachines, 9(3), 115.
- Mahor, S., Allen, J., & Ghista, D. (2020). Implantable medical devices: Design, development, and clinical applications. Materials, 13(18), 4062.
This is an excellent review, particularly the section on 3D printing for customized implants. The potential for personalized medicine through tailored implant design offers exciting possibilities for improved patient outcomes and reduced complications. Further exploration of biocompatible materials compatible with 3D printing would be valuable.
Thanks so much for your kind words! I’m glad you found the section on 3D printing interesting. I agree that further research into biocompatible materials optimized for additive manufacturing is essential. This could really unlock the full potential of personalized implants and dramatically improve patient care. What specific materials do you think hold the most promise?
Editor: MedTechNews.Uk
Thank you to our Sponsor Esdebe