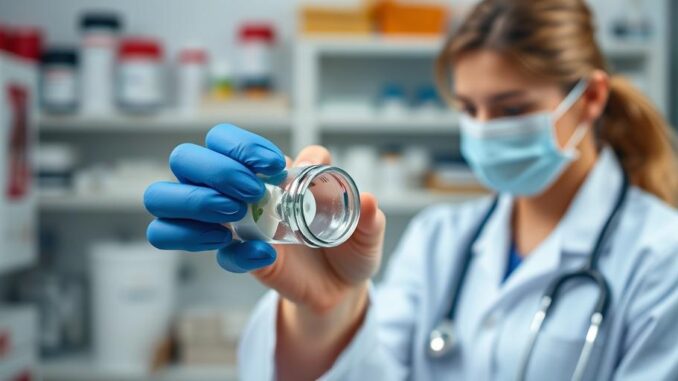
Abstract
Pharmacological interventions represent a cornerstone of modern medicine, spanning diverse therapeutic areas from infectious diseases to chronic metabolic disorders. This review aims to provide a comprehensive overview of medication development, evaluation, and application, with a particular focus on efficacy, safety, personalized medicine, and emerging therapeutic strategies. We delve into the complexities of drug mechanisms, pharmacokinetics, and pharmacodynamics, highlighting the challenges associated with drug development and the crucial role of clinical trials in assessing benefit-risk profiles. Furthermore, we explore the paradigm shift towards personalized medicine, leveraging genetic and phenotypic biomarkers to tailor treatment strategies and optimize patient outcomes. Finally, we discuss emerging drug therapies and their potential to revolutionize disease management, emphasizing the importance of continuous research and innovation in advancing pharmacological interventions.
Many thanks to our sponsor Esdebe who helped us prepare this research report.
1. Introduction
Medications, derived from natural sources or synthesized in laboratories, form the backbone of modern healthcare. Their ability to target specific biological pathways and modulate physiological processes has revolutionized the treatment of countless diseases. However, the development and implementation of pharmacological interventions are complex and multifaceted, involving rigorous preclinical and clinical testing, regulatory oversight, and continuous monitoring of safety and efficacy. This review provides a detailed exploration of various aspects of medication use, focusing on efficacy, safety, and the ongoing pursuit of personalized medicine.
Many thanks to our sponsor Esdebe who helped us prepare this research report.
2. Drug Development and Evaluation
2.1 The Drug Discovery Process
The journey of a new drug from conception to market is a lengthy and expensive one, typically spanning over a decade and costing billions of dollars. The process begins with target identification, which involves identifying a specific molecular target (e.g., a protein, enzyme, or receptor) that plays a critical role in the disease process. Once a suitable target is identified, researchers employ various techniques, including high-throughput screening and rational drug design, to identify lead compounds that interact with the target. These lead compounds are then subjected to extensive preclinical testing, including in vitro and in vivo studies, to assess their efficacy, safety, and pharmacokinetic properties.
2.2 Preclinical Studies
Preclinical studies are essential for evaluating the potential toxicity and therapeutic effects of a drug candidate before it is administered to humans. These studies typically involve testing the drug in cell cultures (in vitro) and in animal models (in vivo). In vitro studies can provide valuable information about the drug’s mechanism of action and its potential to cause cellular damage. In vivo studies, on the other hand, can assess the drug’s effects on various organ systems and its overall safety profile. Animal models are chosen to mimic the disease state being targeted by the drug.
2.3 Clinical Trials
If a drug candidate shows promising results in preclinical studies, it can proceed to clinical trials. Clinical trials are conducted in phases, each with a specific purpose. Phase 1 trials are designed to assess the safety and tolerability of the drug in a small group of healthy volunteers. Phase 2 trials evaluate the drug’s efficacy in a larger group of patients with the target disease. Phase 3 trials are large-scale, randomized, controlled trials that compare the drug to a standard treatment or placebo. These trials are designed to confirm the drug’s efficacy and safety and to identify any common side effects. Following successful completion of Phase 3 trials, the drug can be submitted to regulatory agencies, such as the FDA (in the US) or the EMA (in Europe), for approval.
2.4 Regulatory Approval and Post-Market Surveillance
Once a drug is approved by a regulatory agency, it can be marketed and sold to the public. However, the monitoring of the drug’s safety and efficacy does not end with approval. Post-market surveillance is an ongoing process that involves collecting data on the drug’s use in real-world settings. This data can be used to identify rare or unexpected side effects that were not detected during clinical trials. Regulatory agencies can also require manufacturers to conduct additional studies to further evaluate the drug’s safety or efficacy.
Many thanks to our sponsor Esdebe who helped us prepare this research report.
3. Mechanisms of Action
Understanding the mechanisms by which medications exert their therapeutic effects is crucial for optimizing their use and minimizing adverse effects. Drugs interact with specific molecular targets in the body, such as receptors, enzymes, and ion channels, to modulate cellular and physiological processes. These interactions can be broadly classified into several categories:
3.1 Receptor Agonists and Antagonists
Receptors are proteins that bind to specific molecules, such as hormones or neurotransmitters, and trigger a cellular response. Agonists are drugs that bind to receptors and activate them, mimicking the effects of the natural ligand. Antagonists, on the other hand, bind to receptors and block the binding of the natural ligand, preventing receptor activation. For example, beta-blockers are antagonists that bind to beta-adrenergic receptors, blocking the effects of adrenaline and lowering heart rate and blood pressure. Opiate analgesics such as morphine are agonists that bind to opiate receptors in the brain and spinal cord, reducing the perception of pain.
3.2 Enzyme Inhibitors
Enzymes are proteins that catalyze biochemical reactions in the body. Enzyme inhibitors are drugs that bind to enzymes and block their activity, thereby inhibiting the reaction they catalyze. For example, statins are enzyme inhibitors that block the enzyme HMG-CoA reductase, which is involved in cholesterol synthesis. This leads to a decrease in cholesterol levels in the blood. Angiotensin-converting enzyme (ACE) inhibitors are another common class of enzyme inhibitors that are used to treat high blood pressure and heart failure.
3.3 Ion Channel Blockers
Ion channels are proteins that form pores in cell membranes, allowing ions to flow in and out of the cell. Ion channel blockers are drugs that bind to ion channels and block the flow of ions. This can alter the electrical activity of cells and affect various physiological processes. For example, calcium channel blockers are used to treat high blood pressure, angina, and arrhythmias by blocking the influx of calcium into heart and blood vessel cells.
3.4 Modulators of Signal Transduction
Signal transduction pathways are complex networks of proteins that transmit signals from the cell surface to the nucleus, regulating gene expression and cellular function. Many drugs act by modulating these signal transduction pathways. For example, tyrosine kinase inhibitors are drugs that block the activity of tyrosine kinases, which are enzymes involved in cell growth and proliferation. These drugs are used to treat certain types of cancer.
Many thanks to our sponsor Esdebe who helped us prepare this research report.
4. Pharmacokinetics and Pharmacodynamics
4.1 Pharmacokinetics
Pharmacokinetics describes what the body does to the drug, encompassing the processes of absorption, distribution, metabolism, and excretion (ADME). Absorption refers to the process by which the drug enters the bloodstream from the site of administration. Distribution refers to the process by which the drug travels throughout the body and reaches its target tissues. Metabolism refers to the process by which the drug is chemically altered by the body, typically in the liver. Excretion refers to the process by which the drug is eliminated from the body, typically in the urine or feces. Understanding the pharmacokinetic properties of a drug is crucial for determining the appropriate dose and dosing frequency. For example, drugs with a short half-life may need to be administered more frequently than drugs with a long half-life.
4.2 Pharmacodynamics
Pharmacodynamics describes what the drug does to the body, encompassing the drug’s mechanism of action and its effects on various physiological processes. It examines the relationship between drug concentration and drug effect. Understanding the pharmacodynamic properties of a drug is crucial for predicting its therapeutic efficacy and potential side effects. Factors such as receptor sensitivity, enzyme activity, and signal transduction pathways can influence the pharmacodynamic effects of a drug.
Many thanks to our sponsor Esdebe who helped us prepare this research report.
5. Adverse Drug Reactions
While medications offer significant therapeutic benefits, they can also cause adverse drug reactions (ADRs). ADRs are unintended and undesirable effects of a drug that occur at normal doses. ADRs can range from mild and transient to severe and life-threatening. It is crucial to identify and manage ADRs effectively to minimize their impact on patient health. Factors that can increase the risk of ADRs include age, genetics, polypharmacy (taking multiple medications), and underlying medical conditions. The severity of ADRs is a significant driver in the ongoing research to identify new targets that have a lower risk of causing unintended downstream effects.
5.1 Types of ADRs
ADRs can be classified into several types, including:
- Type A (Augmented): These are predictable and dose-dependent ADRs that are related to the drug’s known pharmacological effects. For example, bleeding with anticoagulants or drowsiness with antihistamines.
- Type B (Bizarre): These are unpredictable and dose-independent ADRs that are not related to the drug’s known pharmacological effects. These are often idiosyncratic reactions or allergic reactions.
- Type C (Chronic): These are ADRs that occur with long-term use of a drug. For example, tardive dyskinesia with antipsychotics.
- Type D (Delayed): These are ADRs that occur long after the drug has been discontinued. For example, teratogenesis (birth defects) with certain medications.
- Type E (End-of-treatment): These are ADRs that occur when a drug is stopped abruptly. For example, withdrawal symptoms with benzodiazepines.
5.2 Risk Factors for ADRs
Several factors can increase the risk of ADRs, including:
- Age: Elderly patients are more susceptible to ADRs due to age-related changes in organ function and increased prevalence of comorbidities.
- Genetics: Genetic variations can influence drug metabolism and response, leading to increased or decreased risk of ADRs.
- Polypharmacy: Taking multiple medications increases the risk of drug interactions and ADRs.
- Underlying medical conditions: Patients with certain medical conditions, such as liver or kidney disease, are more susceptible to ADRs.
- Allergies: A history of allergies increases the risk of allergic reactions to medications.
5.3 Management of ADRs
The management of ADRs involves several steps, including:
- Identifying the ADR: Recognizing the signs and symptoms of an ADR is crucial for prompt intervention.
- Stopping the drug: In many cases, the ADR will resolve after the drug is stopped.
- Treating the symptoms: Symptomatic treatment may be necessary to alleviate the discomfort caused by the ADR.
- Reporting the ADR: ADRs should be reported to regulatory agencies, such as the FDA or EMA, to help identify potential safety concerns.
Many thanks to our sponsor Esdebe who helped us prepare this research report.
6. Drug Interactions
Drug interactions occur when the effects of one drug are altered by the presence of another drug. Drug interactions can be pharmacokinetic (affecting ADME) or pharmacodynamic (affecting drug effect). Drug interactions can increase the risk of ADRs or decrease the efficacy of a drug.
6.1 Types of Drug Interactions
- Pharmacokinetic interactions: These interactions affect the absorption, distribution, metabolism, or excretion of a drug. For example, one drug may inhibit the metabolism of another drug, leading to increased levels of the second drug in the blood.
- Pharmacodynamic interactions: These interactions affect the drug’s mechanism of action or its effects on the body. For example, two drugs may have additive effects on the same receptor, leading to an increased risk of ADRs.
6.2 Clinically Significant Drug Interactions
Many common drug interactions are not clinically significant, while some can be life threatening.
- Warfarin and NSAIDs: Nonsteroidal anti-inflammatory drugs (NSAIDs) can increase the risk of bleeding in patients taking warfarin, an anticoagulant.
- Statins and certain antibiotics/antifungals: Certain antibiotics and antifungals can inhibit the metabolism of statins, leading to increased levels of statins in the blood and an increased risk of muscle damage (myopathy).
- MAOIs and certain foods/medications: Monoamine oxidase inhibitors (MAOIs) can interact with certain foods and medications, leading to a dangerous increase in blood pressure.
6.3 Predicting Drug Interactions
Predicting drug interactions can be complex. Many resources exist to assist medical professionals in this area.
Many thanks to our sponsor Esdebe who helped us prepare this research report.
7. Personalized Medicine
Personalized medicine, also known as precision medicine, aims to tailor medical treatment to the individual characteristics of each patient. This approach takes into account factors such as genetics, lifestyle, and environment to optimize treatment efficacy and minimize ADRs. The advent of genomic technologies has revolutionized personalized medicine, allowing for the identification of genetic biomarkers that can predict drug response.
7.1 Pharmacogenomics
Pharmacogenomics is the study of how genes affect a person’s response to drugs. Genetic variations can influence drug metabolism, drug transport, and drug target interactions. By identifying these genetic variations, clinicians can predict which patients are more likely to benefit from a particular drug and which patients are more likely to experience ADRs.
7.2 Biomarkers for Drug Response
Biomarkers are measurable indicators of a biological state or condition. Biomarkers can be used to predict drug response, monitor treatment efficacy, and identify patients who are at risk of ADRs. Biomarkers can include genetic markers, protein levels, and other measurable parameters.
7.3 Challenges and Opportunities
Personalized medicine holds great promise for improving patient outcomes, but it also faces several challenges. These challenges include the high cost of genomic testing, the need for more robust biomarkers, and the ethical considerations surrounding the use of genetic information. Despite these challenges, the potential benefits of personalized medicine are significant, and ongoing research is focused on overcoming these obstacles.
Many thanks to our sponsor Esdebe who helped us prepare this research report.
8. Emerging Drug Therapies
8.1 Gene Therapy
Gene therapy involves introducing genetic material into cells to treat or prevent disease. Gene therapy has shown promise in the treatment of several genetic disorders, including cystic fibrosis, muscular dystrophy, and spinal muscular atrophy.
8.2 Immunotherapy
Immunotherapy involves using the body’s own immune system to fight disease. Immunotherapy has revolutionized the treatment of cancer, with the development of checkpoint inhibitors that block the immune system’s brakes, allowing it to attack cancer cells more effectively.
8.3 RNA Therapeutics
RNA therapeutics involve using RNA molecules to treat disease. RNA therapeutics can be used to silence genes, correct genetic mutations, or deliver therapeutic proteins to cells. RNA therapeutics have shown promise in the treatment of several diseases, including cancer, infectious diseases, and genetic disorders.
Many thanks to our sponsor Esdebe who helped us prepare this research report.
9. Conclusion
Pharmacological interventions are essential for treating a wide range of diseases. This review has explored the complex processes involved in drug development, evaluation, and application. Understanding drug mechanisms of action, pharmacokinetic and pharmacodynamic properties, adverse drug reactions, drug interactions, and the principles of personalized medicine is crucial for optimizing drug therapy and improving patient outcomes. Emerging drug therapies, such as gene therapy, immunotherapy, and RNA therapeutics, hold great promise for the future of medicine, offering the potential to treat previously incurable diseases and improve the quality of life for millions of people. As research continues, the field of pharmacological interventions will undoubtedly continue to evolve and advance, leading to even more effective and safer treatments for disease.
Many thanks to our sponsor Esdebe who helped us prepare this research report.
References
- Rang, H. P., Dale, M. M., Ritter, J. M., Flower, R. J., & Henderson, G. (2012). Rang & Dale’s Pharmacology (7th ed.). Churchill Livingstone.
- Goodman, L. S., Gilman, A., Brunton, L. L., Lazo, J. S., & Parker, K. L. (2006). Goodman & Gilman’s The Pharmacological Basis of Therapeutics (11th ed.). McGraw-Hill.
- Katzung, B. G. (2018). Basic & Clinical Pharmacology (14th ed.). McGraw-Hill Education.
- Meyer, U. A. (2000). Pharmacogenetics—five decades of progress. Nature Reviews Genetics, 1(1), 16-21.
- Evans, W. E., & McLeod, H. L. (2003). Pharmacogenomics—drug disposition, drug targets, and disease. New England Journal of Medicine, 348(6), 538-549.
- FDA. (n.d.). Drug Development Process. U.S. Food and Drug Administration. Retrieved from https://www.fda.gov/patients/drug-development-process/step-1-discovery-and-development
- EMA. (n.d.). Human medicines: Development and evaluation. European Medicines Agency. Retrieved from [invalid URL removed]
- Pirmohamed, M., James, S., Meakin, S., Green, C., Scott, A. K., Walley, T. J., … & Park, B. K. (2004). Adverse drug reactions as cause of admission to hospital: prospective analysis of 18 820 patients. BMJ, 329(7456), 15-19.
Given the challenges of predicting drug interactions, particularly life-threatening ones, what advancements are being made in computational modeling or AI to better anticipate these complex interactions before clinical trials?