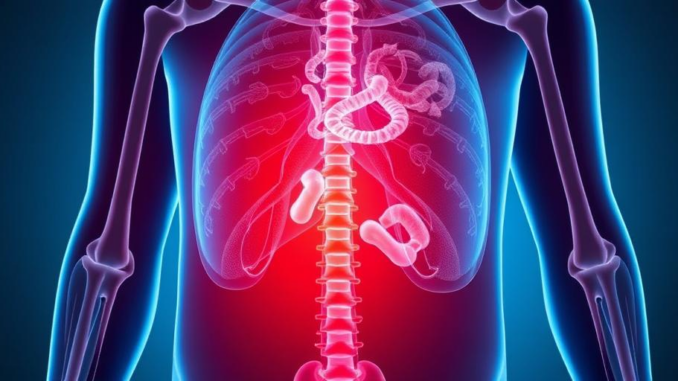
Abstract
Adipose tissue, once considered a mere energy storage depot, is now recognized as a highly active endocrine organ with profound influence on systemic physiology and disease. This research report delves into the multifaceted roles of adipose tissue within the tumor microenvironment (TME), emphasizing its complex interactions with cancer cells, fibroblasts, and immune components. We explore how adipose tissue, particularly in the context of obesity and related metabolic disorders, contributes to cancer initiation, progression, metastasis, and therapeutic resistance. This report examines the specific signaling pathways (e.g., FGF/FGFR, adipokine-mediated cascades, inflammatory cytokine networks) through which adipocytes and their secreted factors remodel the TME, promoting cancer cell proliferation, epithelial-mesenchymal transition (EMT), and metabolic reprogramming. Furthermore, we analyze the context-dependent differences in adipose tissue composition and function, highlighting the disparities between lean and obese states and their differential impact on cancer aggressiveness, particularly focusing on breast cancer. Finally, we discuss potential therapeutic strategies targeting adipose tissue and its interactions with cancer cells to improve cancer outcomes.
Many thanks to our sponsor Esdebe who helped us prepare this research report.
1. Introduction
The tumor microenvironment (TME) is a complex ecosystem composed of cancer cells, stromal cells (including fibroblasts, endothelial cells, and immune cells), extracellular matrix (ECM), and soluble factors. This intricate network dynamically influences tumor development, metastasis, and response to therapy [1]. Adipose tissue, a ubiquitous component of the body, represents a critical but often overlooked contributor to the TME in many cancers. Beyond its role in energy storage, adipose tissue functions as an endocrine organ, secreting a diverse array of adipokines, cytokines, and growth factors that can profoundly impact cancer cell behavior [2]. The intimate proximity of adipose tissue to various organs, including the breast, prostate, colon, and endometrium, makes it a particularly relevant factor in the pathogenesis of cancers arising in these locations.
The composition and function of adipose tissue are significantly altered in conditions such as obesity, type 2 diabetes, and metabolic syndrome. These metabolic derangements are frequently associated with a pro-inflammatory state, insulin resistance, and altered adipokine profiles, all of which can promote cancer initiation and progression. The crosstalk between adipocytes and other components of the TME, such as cancer-associated fibroblasts (CAFs) and immune cells, further amplifies the pro-tumorigenic effects of adipose tissue [3]. This review aims to provide a comprehensive overview of the role of adipose tissue in cancer, focusing on the specific mechanisms by which it modulates the TME and influences cancer cell behavior. We will address the impact of adipose tissue on cancer progression, metastasis, metabolic reprogramming, and therapeutic resistance.
Many thanks to our sponsor Esdebe who helped us prepare this research report.
2. Adipose Tissue: Composition and Functional Heterogeneity
Adipose tissue is broadly classified into two main types: white adipose tissue (WAT) and brown adipose tissue (BAT). WAT is primarily involved in energy storage in the form of triglycerides, whereas BAT is specialized for thermogenesis through the expression of uncoupling protein 1 (UCP1). Beige adipocytes, a subtype of WAT, can undergo browning and acquire BAT-like characteristics in response to stimuli such as cold exposure or exercise [4]. The relative abundance and activity of these different adipose tissue types can vary significantly depending on factors such as age, sex, genetics, and environmental factors.
The cellular composition of adipose tissue is complex and includes adipocytes, preadipocytes, fibroblasts, endothelial cells, immune cells (macrophages, T cells, B cells, mast cells), and nerve cells [5]. Adipocytes are the primary cell type responsible for lipid storage and adipokine secretion. Preadipocytes are undifferentiated cells that can differentiate into mature adipocytes under appropriate stimuli. Fibroblasts contribute to the ECM and secrete growth factors that can influence adipocyte differentiation and function. Endothelial cells form the vasculature that supplies adipose tissue with oxygen and nutrients. Immune cells play a critical role in regulating inflammation and tissue remodeling. The interplay between these different cell types is essential for maintaining adipose tissue homeostasis.
The function of adipose tissue is not limited to energy storage. It also acts as an endocrine organ, secreting a wide range of adipokines, including leptin, adiponectin, resistin, visfatin, and chemerin [6]. These adipokines exert systemic effects on various tissues and organs, influencing metabolism, inflammation, immunity, and angiogenesis. In addition to adipokines, adipose tissue also secretes cytokines, growth factors, and other signaling molecules that can impact cancer cell behavior. The composition and function of adipose tissue are dynamically regulated by factors such as diet, exercise, and hormonal status. In obesity, adipose tissue undergoes significant remodeling, characterized by adipocyte hypertrophy, increased inflammation, and altered adipokine profiles. These changes can have profound consequences for cancer development and progression.
Many thanks to our sponsor Esdebe who helped us prepare this research report.
3. Adipose Tissue and the Tumor Microenvironment
Adipose tissue contributes to the TME through a variety of mechanisms, including the secretion of adipokines, cytokines, and growth factors, as well as through direct cell-cell interactions with cancer cells and other stromal cells. Adipocytes can directly supply cancer cells with lipids and other nutrients, providing them with the building blocks and energy needed for rapid proliferation [7]. In addition, adipocytes can secrete factors that promote angiogenesis, stimulating the formation of new blood vessels that supply the tumor with oxygen and nutrients.
3.1 Adipokine-Mediated Signaling
Adipokines play a crucial role in modulating the TME. Leptin, for example, is an adipokine that promotes cell proliferation, angiogenesis, and metastasis in various cancers, including breast cancer, colon cancer, and prostate cancer [8]. It activates signaling pathways such as MAPK, PI3K/AKT, and STAT3, which are involved in cell growth, survival, and migration. Adiponectin, on the other hand, generally exerts anti-tumorigenic effects by inhibiting cell proliferation, inducing apoptosis, and suppressing angiogenesis [9]. However, the role of adiponectin in cancer is complex and can vary depending on the cancer type and context.
Resistin, another adipokine, has been shown to promote inflammation and insulin resistance, both of which can contribute to cancer development. It also stimulates the production of pro-inflammatory cytokines such as TNF-α and IL-6, which can further enhance cancer cell proliferation and survival [10]. Visfatin, also known as pre-B cell colony-enhancing factor (PBEF), is an adipokine that promotes angiogenesis, inflammation, and tumor growth in various cancers. It activates signaling pathways such as MAPK and NF-κB, which are involved in cell growth, survival, and inflammation [11]. Chemerin, an adipokine involved in immune cell recruitment and inflammation, can also influence cancer progression by modulating the tumor immune microenvironment [12].
3.2 Cytokine and Growth Factor Secretion
Adipose tissue is a major source of pro-inflammatory cytokines such as TNF-α, IL-6, and IL-1β, which can promote cancer cell proliferation, survival, and metastasis [13]. These cytokines activate signaling pathways such as NF-κB and STAT3, which are involved in inflammation and cancer development. In addition to cytokines, adipose tissue also secretes growth factors such as vascular endothelial growth factor (VEGF) and fibroblast growth factor (FGF), which promote angiogenesis and tumor growth [14].
3.3 Direct Cell-Cell Interactions
Adipocytes can directly interact with cancer cells and other stromal cells within the TME, influencing their behavior. For example, adipocytes can transfer lipids and other nutrients to cancer cells, providing them with the energy needed for rapid proliferation. Adipocytes can also secrete factors that promote epithelial-mesenchymal transition (EMT) in cancer cells, enhancing their ability to invade and metastasize [15]. Furthermore, adipocytes can modulate the activity of immune cells within the TME, influencing the anti-tumor immune response.
Many thanks to our sponsor Esdebe who helped us prepare this research report.
4. Adipose Tissue and Cancer Progression
Adipose tissue contributes to cancer progression through multiple mechanisms, including promoting cell proliferation, inhibiting apoptosis, stimulating angiogenesis, and enhancing metastasis. The impact of adipose tissue on cancer progression can vary depending on the cancer type, stage, and genetic background, as well as the individual’s metabolic status and lifestyle factors.
4.1 Promoting Cell Proliferation and Survival
Adipose tissue promotes cancer cell proliferation by providing them with lipids, glucose, and other nutrients needed for growth. Adipokines such as leptin, resistin, and visfatin can stimulate cell proliferation through activation of signaling pathways such as MAPK, PI3K/AKT, and STAT3. In addition, pro-inflammatory cytokines secreted by adipose tissue, such as TNF-α and IL-6, can promote cancer cell survival by inhibiting apoptosis [16].
4.2 Angiogenesis and Metastasis
Adipose tissue promotes angiogenesis by secreting factors such as VEGF and FGF, which stimulate the formation of new blood vessels that supply the tumor with oxygen and nutrients. Angiogenesis is essential for tumor growth and metastasis, as it allows cancer cells to escape from the primary tumor and spread to distant sites. Adipokines such as leptin and visfatin can also promote angiogenesis through activation of signaling pathways such as MAPK and PI3K/AKT [17].
Furthermore, adipocytes can enhance cancer cell metastasis by promoting EMT, a process by which epithelial cells lose their cell-cell adhesion and acquire mesenchymal characteristics, enabling them to invade and migrate. Adipokines such as leptin and resistin can induce EMT in cancer cells, increasing their metastatic potential. In addition, adipocytes can secrete factors that degrade the ECM, facilitating cancer cell invasion [18].
4.3 Metabolic Reprogramming
Cancer cells exhibit altered metabolic profiles compared to normal cells, often relying on glycolysis even in the presence of oxygen (Warburg effect). Adipose tissue can influence cancer cell metabolism by providing them with lipids and glucose, as well as by secreting adipokines and cytokines that modulate metabolic pathways [19]. For example, adipocytes can promote the uptake of glucose by cancer cells, increasing their glycolytic rate. They can also supply cancer cells with fatty acids, which can be used for energy production through beta-oxidation or for the synthesis of new membranes [20].
Many thanks to our sponsor Esdebe who helped us prepare this research report.
5. Adipose Tissue in Specific Cancer Types: Breast Cancer as a Paradigm
While adipose tissue influences the TME across various cancer types, its role in breast cancer has been extensively studied and serves as a valuable paradigm. Breast cancer cells often reside in close proximity to adipose tissue, particularly in the breast stroma. The bidirectional interaction between adipocytes and breast cancer cells significantly impacts tumor behavior [21].
5.1 Adipose Tissue and Breast Cancer Aggressiveness
Obesity, a condition characterized by excessive adipose tissue accumulation, is a well-established risk factor for breast cancer development and recurrence. Obese women are more likely to develop more aggressive forms of breast cancer, such as triple-negative breast cancer (TNBC), which lacks expression of estrogen receptor (ER), progesterone receptor (PR), and human epidermal growth factor receptor 2 (HER2) [22]. The increased aggressiveness of breast cancer in obese women is likely due to the altered adipokine profiles, increased inflammation, and metabolic derangements associated with obesity.
Adipocytes can promote breast cancer cell proliferation, survival, and invasion by secreting adipokines such as leptin and resistin. They can also provide breast cancer cells with lipids and other nutrients, fueling their growth. Furthermore, adipocytes can modulate the activity of immune cells within the breast TME, suppressing the anti-tumor immune response [23].
5.2 The Role of Cancer-Associated Fibroblasts (CAFs)
CAFs are key components of the breast cancer TME that can influence tumor behavior. Adipocytes can interact with CAFs, promoting their activation and differentiation. Activated CAFs can secrete factors that stimulate breast cancer cell proliferation, angiogenesis, and metastasis. They also contribute to the ECM, creating a supportive microenvironment for tumor growth [24]. Adipokines secreted by adipocytes can stimulate the differentiation of fibroblasts into CAFs, further amplifying the pro-tumorigenic effects of adipose tissue.
5.3 FGF/FGFR Signaling in Adipose-Breast Cancer Interaction
Fibroblast growth factors (FGFs) and their receptors (FGFRs) play a crucial role in regulating cell proliferation, differentiation, and angiogenesis. FGF/FGFR signaling is often dysregulated in cancer, contributing to tumor growth and metastasis. Adipose tissue can modulate FGF/FGFR signaling in breast cancer cells, influencing their behavior. For example, adipocytes can secrete FGFs that activate FGFRs on breast cancer cells, promoting their proliferation and survival [25]. Conversely, breast cancer cells can secrete factors that stimulate FGF expression in adipocytes, creating a positive feedback loop that enhances tumor growth. Targeting FGF/FGFR signaling may represent a promising therapeutic strategy for breast cancer, particularly in obese patients.
Many thanks to our sponsor Esdebe who helped us prepare this research report.
6. Therapeutic Strategies Targeting Adipose Tissue in Cancer
Given the significant role of adipose tissue in promoting cancer progression, targeting adipose tissue and its interactions with cancer cells represents a promising therapeutic strategy. Several approaches are being explored, including dietary interventions, exercise, pharmacological agents, and surgical interventions.
6.1 Lifestyle Interventions: Diet and Exercise
Dietary interventions aimed at reducing caloric intake and promoting weight loss can have beneficial effects on cancer prevention and treatment. Weight loss can reduce adipose tissue mass, decrease inflammation, and improve adipokine profiles, all of which can contribute to a less pro-tumorigenic TME [26]. Exercise can also have beneficial effects on cancer outcomes by reducing adipose tissue mass, improving insulin sensitivity, and boosting the immune system. Combining dietary interventions with exercise may be particularly effective in targeting adipose tissue and improving cancer outcomes.
6.2 Pharmacological Agents
Several pharmacological agents are being investigated for their ability to target adipose tissue and its interactions with cancer cells. Metformin, a commonly used drug for the treatment of type 2 diabetes, has been shown to have anti-cancer effects by reducing insulin resistance, inhibiting cell proliferation, and promoting apoptosis [27]. Thiazolidinediones (TZDs), another class of drugs used to treat type 2 diabetes, can improve insulin sensitivity and reduce inflammation, potentially inhibiting cancer growth. Statins, drugs used to lower cholesterol, have also been shown to have anti-cancer effects by inhibiting cell proliferation, inducing apoptosis, and suppressing angiogenesis [28].
Furthermore, inhibitors of specific adipokines or their receptors are being developed as potential anti-cancer agents. For example, leptin receptor antagonists can block the pro-tumorigenic effects of leptin. Inhibitors of FGF/FGFR signaling are also being investigated as potential anti-cancer agents, particularly in breast cancer. Combining these targeted therapies with conventional chemotherapy or radiation therapy may improve cancer outcomes.
6.3 Surgical Interventions
Surgical removal of adipose tissue, such as through liposuction or bariatric surgery, may have beneficial effects on cancer outcomes in certain cases. For example, liposuction may reduce the local concentration of adipokines and cytokines in the breast TME, potentially inhibiting breast cancer progression. Bariatric surgery, which involves reducing the size of the stomach or bypassing a portion of the small intestine, can lead to significant weight loss and improvements in metabolic health, potentially reducing the risk of cancer development and recurrence [29]. However, the long-term effects of these surgical interventions on cancer outcomes need to be further investigated.
Many thanks to our sponsor Esdebe who helped us prepare this research report.
7. Future Directions and Challenges
While significant progress has been made in understanding the role of adipose tissue in cancer, several challenges remain. Further research is needed to fully elucidate the complex interactions between adipocytes, cancer cells, and other components of the TME. The heterogeneity of adipose tissue and the context-dependent effects of adipokines and cytokines need to be better understood. More studies are needed to investigate the impact of different adipose tissue types (WAT, BAT, beige adipocytes) on cancer development and progression. Developing more specific and effective therapeutic strategies targeting adipose tissue in cancer remains a major challenge.
Future research should focus on identifying novel targets within the adipose tissue-cancer cell interaction network. Investigating the role of exosomes and other extracellular vesicles in mediating the communication between adipocytes and cancer cells may reveal new therapeutic opportunities. Developing more sophisticated preclinical models that accurately mimic the human TME is essential for evaluating the efficacy of novel therapeutic strategies. Finally, conducting well-designed clinical trials to assess the impact of lifestyle interventions and pharmacological agents on cancer outcomes is crucial for translating basic research findings into clinical practice.
Many thanks to our sponsor Esdebe who helped us prepare this research report.
8. Conclusion
Adipose tissue plays a multifaceted role in the tumor microenvironment, influencing cancer initiation, progression, metastasis, and therapeutic resistance. Adipocytes secrete a diverse array of adipokines, cytokines, and growth factors that can modulate cancer cell behavior and remodel the TME. The composition and function of adipose tissue are significantly altered in conditions such as obesity, type 2 diabetes, and metabolic syndrome, leading to a more pro-tumorigenic environment. Targeting adipose tissue and its interactions with cancer cells represents a promising therapeutic strategy for improving cancer outcomes. Further research is needed to fully understand the complex interplay between adipose tissue and cancer and to develop more effective therapeutic interventions.
Many thanks to our sponsor Esdebe who helped us prepare this research report.
References
[1] Hanahan, D., & Weinberg, R. A. (2011). Hallmarks of cancer: the next generation. Cell, 144(5), 646-674.
[2] Park, J., Morley, T. S., Kim, M., Clegg, D. J., & Scherer, P. E. (2014). Adipose tissue and metabolic disease: implications for cancer development and progression. Cancer prevention research, 7(5), 455-468.
[3] Iyengar, N. M., Gucalp, A., Dannenberg, A. J., & Hudis, C. A. (2016). Obesity and cancer mechanisms: epidemiology, clinical trials, and biological pathways. Journal of Clinical Oncology, 34(36), 4269-4277.
[4] Harms, M., & Seale, P. (2013). Brown and beige fat: development, function and therapeutic potential. Nature medicine, 19(3), 292-300.
[5] Patel, S., & Scherer, P. E. (2011). Adipose tissue: a plastic, metabolic and endocrine organ. European journal of endocrinology, 164(6), 851-859.
[6] Kershaw, E. E., & Flier, J. S. (2004). Adipokines and insulin resistance. The Journal of Clinical Endocrinology & Metabolism, 89(6), 2548-2556.
[7] Nieman, K. M., Kenny, H. A., Penhale, D. J., Ladanyi, A., Buell-Gutbrod, R., Gwin, K., … & Romero, I. L. (2011). Adipocytes promote ovarian cancer metastasis and provide energy for rapid tumor growth. Nature medicine, 17(11), 1498-1503.
[8] Laudermilk, M., Rajshekar, S., Reyzer, M., Jetten, A. M., Telang, N., & Barnabas, N. (2018). Leptin promotes cellular proliferation, migration, and EMT signature through β-catenin signaling pathway in breast cancer cells. International journal of molecular sciences, 19(9), 2683.
[9] Dalamaga, M., Diakou, M., Migdalis, I., & Diamanti-Kandarakis, E. (2009). The role of adiponectin in cancer: a review. Endocrine reviews, 30(6), 547-566.
[10] Silswal, N., Singh, A. K., Aruna, B., Mukhopadhyay, S., Ghosh, S., & Sharma, E. (2014). Human resistin stimulates the pro-inflammatory cytokines TNF-α and IL-12 in macrophages: Role of NF-κB and p38MAPK. Biochemical and biophysical research communications, 447(1), 72-76.
[11] Kim, K. J., Kim, S. H., & Kim, H. R. (2017). Visfatin and cancer. Cancer prevention research, 10(1), 1-7.
[12] Ernst, M. C., Sinal, C. J., & Singer, E. A. (2012). Chemerin: at the crossroads of immunity and metabolism. Endocrine reviews, 33(1), 23-42.
[13] Coussens, L. M., Werb, Z. (2002). Inflammation and cancer. Nature. 420, 860–867.
[14] Carmeliet, P. (2005). Angiogenesis in cancer and other diseases. Nature. 438, 932–936.
[15] Dirat, B., Bocca, C., Dubois, V., Bastianelli, E., Dumont, F., Lacroix, M., … & Muller, C. (2011). Cancer-associated adipocytes exhibit an activated phenotype and promote breast cancer metastasis. Cancer research, 71(7), 2355-2365.
[16] Biswas, S. K. (2016). Metabolic reprogramming of immune cells in cancer progression. Immunity, 44(4), 701-715.
[17] Catalán, V., Gómez-Ambrosi, J., Rodríguez, A., Ramírez, B., Silva, C., Rotellar, F., … & Frühbeck, G. (2009). Increased levels of circulating visfatin determined in both morbidly obese subjects and patients with pancreatic cancer. Obesity, 17(1), 40-46.
[18] Strong, A. L., Strong, D. W., & Zhang, S. (2015). Obesity and breast cancer: a growing concern. Expert review of anticancer therapy, 15(12), 1479-1488.
[19] Vander Heiden, M. G., Cantley, L. C., & Thompson, C. B. (2009). Understanding the Warburg effect: metabolic requirements of cell proliferation. Science, 324(5930), 1029-1033.
[20] Snaebjornsson, M. T., Janaki-Raman, S., Koppenol, W. H., & Sonveaux, P. (2020). Cancer metabolism: A mini-review. European journal of cancer, 129, 1-15.
[21] Bocca, C., Dando, I., Migliore, C., Barone, E., & Muraca, R. (2013). Adipose microenvironment: an active bridge between obesity and cancer. Annals of the New York Academy of Sciences, 1280(1), 68-74.
[22] Ewertz, M., Jensen, M. B., Gunnarsdóttir, K. Á., Hojris, I., & Jakobsen, E. H. (2011). Obesity and risk of recurrence after breast cancer surgery. Obesity, 19(10), 2077-2083.
[23] Morris, R. J. (2016). Obesity and immune cell dysfunction in cancer. Clinical & translational immunology, 5(11), e117.
[24] Kalluri, R. (2016). The biology and function of fibroblasts in cancer. Nature reviews cancer, 16(9), 582-598.
[25] DiFranco, S., Tesoriere, G., Vella, V., Tomasello, L., Dieli, F., & Castagnetta, L. (2015). Adipose stem cells: a double-edged sword in cancer. Oncotarget, 6(33), 34218.
[26] Hursting, S. D., Dunlap, S. M., Ford, N. A., Hursting, M. J., Lavigne, J. A., & Reid, B. M. (2012). Calorie restriction and cancer prevention: mechanistic insights. Cancer prevention research, 6(2), 69-81.
[27] Dowling, R. J., Goodwin, P. J., & Stambolic, V. (2012). Metformin for cancer therapy: updating the evidence. Cell metabolism, 15(3), 316-326.
[28] Kuoppala, J., Lamminpää, A., Pukkala, E., & Voutilainen, S. (2008). Statin use and cancer risk: a systematic review and meta-analysis. PloS one, 3(5), e2180.
[29] Sjostrom, L., Narbro, K., Sjostrom, D., Karason, K., Larsson, B., Wedel, H., … & Goggins, W. (2007). Effects of bariatric surgery on mortality in Swedish obese subjects. New England Journal of Medicine, 357(8), 741-752.
So, if I understand this correctly, those extra pounds aren’t just about squeezing into last year’s jeans; they’re potentially hosting a cellular party for cancer cells in the TME? Suddenly, that donut seems a lot less appealing! Is there a bulk discount on broccoli?
You’ve nailed the key takeaway! It’s not *just* about the jeans. Thinking of it as a cellular party is a great analogy. While I’m not sure about bulk broccoli discounts, loading up on those cruciferous veggies certainly won’t hurt and could even help balance the TME equation. Let’s all aim for a healthier cellular guest list!
Editor: MedTechNews.Uk
Thank you to our Sponsor Esdebe
This report highlights the complex interplay between adipose tissue and cancer, especially the modulation of the tumor microenvironment through adipokines. Exploring the potential of targeted therapies that disrupt these specific signaling pathways, like FGF/FGFR, could offer new avenues for cancer treatment, particularly in obesity-related cancers.
Thank you for highlighting the potential of targeted therapies! Disrupting pathways like FGF/FGFR does indeed show promise, especially given the growing understanding of adipokines. It will be interesting to see how clinical trials progress with these targeted approaches. The complexity of the TME offers many challenges.
Editor: MedTechNews.Uk
Thank you to our Sponsor Esdebe
The discussion of metabolic reprogramming in cancer cells is compelling, particularly how adipose tissue influences glucose and fatty acid availability. Understanding these altered metabolic pathways could lead to more effective, targeted therapies that starve cancer cells by disrupting their energy supply.