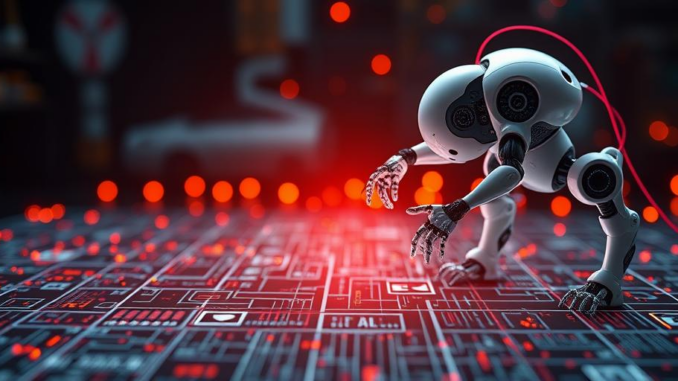
Advanced Surgical Robotics: Miniaturization, Magnetic Actuation, and In-Vivo Imaging Synergies for Next-Generation Medical Procedures
Abstract
Surgical robotics has revolutionized medical interventions, offering enhanced precision, minimally invasive approaches, and improved patient outcomes. This research report explores the cutting-edge advancements in surgical robotics, focusing on the synergistic integration of miniaturization, magnetic actuation, and in-vivo imaging capabilities. The report provides a comprehensive review of diverse robot designs, advanced control systems, biocompatible materials, and imaging modalities employed in similar applications. Challenges and opportunities in the field of micro-robotics for medical procedures are also discussed, with particular attention paid to the integration of artificial intelligence (AI) and machine learning (ML) for autonomous control and real-time image processing. This report aims to present an expert-level perspective on the state-of-the-art technologies and future directions in surgical robotics, highlighting the potential of these advancements to transform diagnostic and therapeutic interventions.
1. Introduction
The field of surgical robotics has witnessed remarkable progress in recent decades, driven by the demand for less invasive, more precise, and more efficient surgical procedures. Traditional surgical robots, while offering significant advantages over conventional open surgery, are often limited by their size, complexity, and accessibility to deep or confined anatomical regions. In response, the development of miniaturized surgical robots, often referred to as micro-robots, has emerged as a promising avenue for accessing previously inaccessible areas and performing intricate tasks with unparalleled precision. The integration of magnetic actuation and in-vivo imaging further enhances the capabilities of these micro-robots, enabling remote control and real-time monitoring within the human body. This convergence of technologies has the potential to revolutionize various medical fields, including diagnostics, targeted drug delivery, and minimally invasive surgery.
This research report provides a comprehensive overview of the current state-of-the-art in surgical micro-robotics, focusing on the key enabling technologies of miniaturization, magnetic actuation, and in-vivo imaging. The report delves into the design considerations, control strategies, material selection, and imaging modalities employed in these systems. Furthermore, it explores the challenges and opportunities associated with the development and clinical translation of surgical micro-robots, with a particular emphasis on the integration of AI and ML for autonomous control and real-time image processing.
2. Miniaturization Strategies in Surgical Robotics
Miniaturization is a crucial aspect of surgical robotics, enabling access to confined anatomical spaces and reducing the invasiveness of surgical procedures. Several approaches have been employed to achieve miniaturization in surgical robots, each with its own advantages and limitations. These approaches can be broadly categorized into: scaling down existing robotic systems, developing novel micro-fabrication techniques, and employing modular or distributed robotic architectures.
2.1. Scaling Down Existing Robotic Systems
One approach to miniaturization involves scaling down existing robotic systems while maintaining their functionality. This approach typically involves the use of smaller actuators, sensors, and structural components. While this approach can leverage existing robotic designs and control algorithms, it often faces challenges related to the scaling of mechanical properties, such as stiffness and strength, as well as the miniaturization of power sources and communication systems. For example, standard laparoscopic robots can be scaled down, but internal force feedback and dexterity are often compromised. The development of advanced materials with high strength-to-weight ratios and efficient micro-actuators is crucial for overcoming these challenges.
2.2. Micro-Fabrication Techniques
Micro-fabrication techniques, such as micro-milling, laser micromachining, and soft lithography, offer the ability to create complex micro-structures with high precision. These techniques are particularly well-suited for creating micro-robots with intricate geometries and integrated functionalities. Soft lithography, for example, allows for the fabrication of micro-fluidic channels and flexible structures, which are essential for applications such as drug delivery and tissue manipulation. Shape memory alloys (SMAs) and polymers with embedded micro-structures can be precisely manufactured using micro-fabrication processes, leading to highly specialized robot designs. The main limitation of micro-fabrication techniques is the cost and time associated with prototyping and manufacturing complex micro-robots.
2.3. Modular and Distributed Robotic Architectures
Modular and distributed robotic architectures offer an alternative approach to miniaturization by dividing the functionality of a surgical robot into multiple smaller modules that can be assembled and controlled independently. This approach allows for greater flexibility and scalability, as well as the ability to reconfigure the robot for different surgical tasks. For example, a swarm of micro-robots could be deployed to perform a complex surgical procedure in a collaborative manner. Each micro-robot could be responsible for a specific task, such as tissue resection, drug delivery, or imaging. The key challenge in this approach is the development of robust communication and coordination strategies for the individual modules. Furthermore, ensuring the biocompatibility and long-term stability of these micro-robots within the body remains a significant concern.
3. Magnetic Actuation for Precise Control
Magnetic actuation offers a non-contact and precise method for controlling the movement and manipulation of surgical micro-robots. This technique utilizes external magnetic fields to exert forces and torques on magnetic materials integrated into the micro-robot design. Magnetic actuation offers several advantages over traditional actuation methods, including: reduced invasiveness, enhanced maneuverability, and the ability to access confined anatomical spaces. Different magnetic actuation strategies have been developed, each with its own strengths and weaknesses.
3.1. Electromagnetic Actuation Systems
Electromagnetic actuation systems (EMAS) utilize electromagnets to generate magnetic fields that can be precisely controlled in three dimensions. These systems typically consist of multiple electromagnets arranged around the patient, allowing for the generation of complex magnetic field gradients. EMAS offer high precision and force control, but they can be bulky and expensive. Furthermore, the magnetic field strength is limited by the power consumption and heat dissipation of the electromagnets. Recent advancements in EMAS technology include the development of smaller, more energy-efficient electromagnets, as well as the integration of real-time feedback control to compensate for magnetic field inhomogeneities and disturbances. [1,2]
3.2. Permanent Magnet Actuation
Permanent magnet actuation utilizes permanent magnets to generate the magnetic field. This approach is simpler and more cost-effective than EMAS, but it offers less flexibility in terms of magnetic field control. Permanent magnets can be attached to robotic arms or mounted on rotating stages to generate specific magnetic field patterns. The strength and direction of the magnetic field can be adjusted by changing the position and orientation of the permanent magnets. Permanent magnet actuation is well-suited for applications where precise control of the magnetic field is not critical. However, controlling force application can be challenging compared to EMAS systems. Novel designs are emerging which combine permanent and electromagnets for hybrid systems.
3.3. Hybrid Magnetic Actuation
Hybrid magnetic actuation combines the advantages of both EMAS and permanent magnet actuation. These systems typically utilize a combination of electromagnets and permanent magnets to generate the magnetic field. The permanent magnets provide a constant magnetic field, while the electromagnets are used to fine-tune the field and provide precise control over the micro-robot’s movement. Hybrid magnetic actuation offers a good balance between precision, cost, and complexity. Research into optimized magnet placements and control algorithms is ongoing to maximize the benefits of these systems.
4. In-Vivo Imaging for Real-Time Monitoring
In-vivo imaging plays a crucial role in surgical micro-robotics, enabling real-time monitoring of the robot’s position, orientation, and interaction with the surrounding tissue. Different imaging modalities have been employed for in-vivo imaging, each with its own advantages and limitations. These modalities can be broadly categorized into: magnetic resonance imaging (MRI), ultrasound imaging, optical coherence tomography (OCT), and X-ray imaging.
4.1. Magnetic Resonance Imaging (MRI)
Magnetic resonance imaging (MRI) offers high-resolution, three-dimensional imaging of soft tissues. MRI can be used to track the position and orientation of micro-robots in real-time, as well as to visualize the surrounding anatomical structures. However, MRI is relatively slow and expensive, and it requires the use of MRI-compatible materials for the micro-robot. Furthermore, the strong magnetic fields used in MRI can interfere with the operation of certain micro-robot components, such as actuators and sensors. Gradient echo sequences can be used for fast imaging but often suffer from lower spatial resolution. Developments in faster MRI sequences and specialized micro-robot designs are addressing these limitations.
4.2. Ultrasound Imaging
Ultrasound imaging is a real-time, cost-effective imaging modality that can be used to track the position of micro-robots within the body. Ultrasound imaging offers good spatial resolution and penetration depth, but it is limited by its sensitivity to air bubbles and bone. Furthermore, the acoustic impedance mismatch between the micro-robot and the surrounding tissue can make it difficult to visualize the robot. Contrast-enhanced ultrasound imaging can improve the visibility of micro-robots, but it requires the injection of contrast agents into the bloodstream. Novel ultrasound transducer technologies are being developed to improve image quality and reduce artifacts.
4.3. Optical Coherence Tomography (OCT)
Optical coherence tomography (OCT) is a high-resolution imaging modality that can be used to visualize the microstructure of tissues. OCT offers excellent spatial resolution, but it is limited by its shallow penetration depth. OCT can be integrated into surgical micro-robots to provide real-time imaging of the tissue being manipulated. However, the integration of OCT into micro-robots can be challenging due to the size and complexity of the optical components. Micro-OCT probes are being developed to overcome these limitations and enable endoscopic imaging with micro-robots.
4.4. X-Ray Imaging
X-ray imaging is a widely used imaging modality that can be used to track the position of micro-robots within the body. X-ray imaging is relatively fast and inexpensive, but it exposes the patient to ionizing radiation. Furthermore, X-ray imaging offers limited soft tissue contrast, making it difficult to visualize the surrounding anatomical structures. Fluoroscopy, a real-time X-ray imaging technique, can be used to track the movement of micro-robots, but it requires the use of radiopaque materials in the micro-robot design. Recent advances include low-dose X-ray imaging and cone-beam computed tomography (CBCT) which can improve image quality while minimizing radiation exposure.
5. Materials for Surgical Micro-Robots
The selection of appropriate materials is crucial for the successful development of surgical micro-robots. The materials must be biocompatible, mechanically robust, and capable of withstanding the harsh environment within the human body. Furthermore, the materials must be amenable to micro-fabrication techniques and compatible with the chosen actuation and imaging modalities. Common materials used in surgical micro-robots include polymers, metals, and ceramics.
5.1. Polymers
Polymers offer a wide range of properties that make them suitable for use in surgical micro-robots. Polymers can be easily micro-fabricated into complex shapes and can be functionalized with various bioactive molecules. Biocompatible polymers, such as poly(lactic-co-glycolic acid) (PLGA) and poly(ethylene glycol) (PEG), are commonly used in biomedical applications. However, polymers typically have lower mechanical strength compared to metals and ceramics. The mechanical properties of polymers can be improved by reinforcing them with nanoparticles or fibers. Biodegradable polymers are particularly attractive for applications where long-term implantation is not desired. [3]
5.2. Metals
Metals offer high mechanical strength and durability, making them suitable for use in structural components of surgical micro-robots. Common metals used in biomedical applications include titanium, stainless steel, and nickel-titanium alloys (nitinol). Titanium is biocompatible and corrosion-resistant, but it is relatively difficult to micro-fabricate. Stainless steel is less expensive than titanium, but it is more prone to corrosion. Nitinol is a shape memory alloy that can be used to create micro-robots with complex movements. However, nickel is potentially toxic, and the use of nitinol in long-term implants is limited. New alloys are constantly being researched to provide improved biocompatibility and manufacturability.
5.3. Ceramics
Ceramics offer high hardness and wear resistance, making them suitable for use in cutting tools and abrasive components of surgical micro-robots. Biocompatible ceramics, such as alumina and zirconia, are commonly used in biomedical applications. Ceramics are typically brittle and difficult to micro-fabricate, but they can be processed using techniques such as laser micromachining and powder metallurgy. Hydroxyapatite, a calcium phosphate ceramic, is similar to the mineral component of bone and can be used to promote bone ingrowth. Recent advances involve using ceramics in nanocomposite form to improve fracture toughness.
6. Control Systems and Algorithms
The development of robust and efficient control systems is crucial for the precise and reliable operation of surgical micro-robots. The control system must be able to compensate for uncertainties in the robot’s dynamics, as well as disturbances from the surrounding environment. Different control strategies have been developed for surgical micro-robots, including: open-loop control, closed-loop control, and AI-based control.
6.1. Open-Loop Control
Open-loop control is the simplest control strategy, where the control input is determined solely by the desired trajectory, without any feedback from the robot’s actual position or orientation. Open-loop control is suitable for applications where the robot’s dynamics are well-known and the disturbances are minimal. However, open-loop control is highly sensitive to uncertainties and disturbances, and it is not suitable for precise manipulation tasks. This type of control is rarely used alone in advanced surgical robotics applications.
6.2. Closed-Loop Control
Closed-loop control utilizes feedback from sensors to continuously adjust the control input and minimize the error between the desired trajectory and the robot’s actual position or orientation. Closed-loop control is more robust to uncertainties and disturbances than open-loop control, and it is suitable for precise manipulation tasks. Common closed-loop control algorithms include proportional-integral-derivative (PID) control, model predictive control (MPC), and adaptive control. PID control is a simple and widely used control algorithm, but it can be difficult to tune for complex systems. MPC is a more advanced control algorithm that can predict the robot’s future behavior and optimize the control input accordingly. Adaptive control can adjust the control parameters in real-time to compensate for changes in the robot’s dynamics or the environment. [4]
6.3. AI-Based Control
AI-based control utilizes artificial intelligence and machine learning techniques to develop autonomous control systems for surgical micro-robots. AI-based control can learn from experience and adapt to changing environments, making it suitable for complex and unstructured tasks. Reinforcement learning is a popular AI technique that can be used to train robots to perform tasks by rewarding desired behaviors and penalizing undesired behaviors. Deep learning can be used to analyze images and sensor data to extract relevant information for control. AI-based control has the potential to significantly improve the autonomy and precision of surgical micro-robots, but it requires large amounts of training data and robust validation methods. The integration of AI also raises ethical considerations regarding the responsibility and accountability of autonomous surgical systems.
7. Challenges and Opportunities
Surgical micro-robotics offers tremendous potential for revolutionizing medical procedures, but it also faces several challenges that must be addressed before widespread clinical adoption. These challenges include: power autonomy, biocompatibility, control complexity, and regulatory hurdles. Addressing these challenges will require interdisciplinary collaboration between engineers, scientists, and clinicians.
7.1. Power Autonomy
Providing sufficient power to surgical micro-robots is a major challenge, particularly for long-duration procedures. Traditional batteries are often too large and bulky for micro-robots, and they can be difficult to recharge within the body. Alternative power sources, such as wireless power transfer and energy harvesting, are being explored. Wireless power transfer utilizes electromagnetic fields to transmit power to the micro-robot, while energy harvesting utilizes vibrations, temperature gradients, or chemical reactions to generate electricity. However, these technologies are still in their early stages of development and require further optimization. Power scavenging from biological sources represents a promising area of research, however, efficiency and long-term stability are still issues to overcome.
7.2. Biocompatibility
Ensuring the biocompatibility of surgical micro-robots is essential for minimizing the risk of adverse reactions and promoting tissue integration. The materials used in the micro-robot must be non-toxic, non-immunogenic, and non-thrombogenic. Furthermore, the surface properties of the micro-robot must be carefully controlled to prevent protein adsorption and cell adhesion. Surface coatings, such as PEG and heparin, can be used to improve the biocompatibility of micro-robots. Long-term in-vivo studies are necessary to assess the biocompatibility of micro-robots and identify potential risks.
7.3. Control Complexity
Controlling the movement and manipulation of surgical micro-robots is a complex task, due to the uncertainties in the robot’s dynamics and the disturbances from the surrounding environment. Developing robust and efficient control systems requires advanced modeling techniques, sensor fusion algorithms, and AI-based control strategies. Furthermore, the control system must be user-friendly and intuitive for surgeons to operate. Haptic feedback can be used to provide surgeons with a sense of touch and improve their control over the micro-robot. The increasing complexity of control systems necessitates rigorous testing and validation to ensure safety and reliability.
7.4. Regulatory Hurdles
The clinical translation of surgical micro-robots is subject to stringent regulatory requirements. The FDA and other regulatory agencies require extensive preclinical testing and clinical trials to demonstrate the safety and efficacy of new medical devices. The regulatory pathway for surgical micro-robots is particularly challenging due to their novelty and complexity. Addressing the regulatory hurdles requires close collaboration between engineers, scientists, clinicians, and regulatory experts. Standardization of testing methods and data reporting can facilitate the regulatory process.
8. Conclusion
Surgical micro-robotics is a rapidly evolving field with the potential to transform medical interventions. The synergistic integration of miniaturization, magnetic actuation, and in-vivo imaging capabilities enables the development of minimally invasive and highly precise surgical tools. While significant progress has been made in recent years, several challenges remain before widespread clinical adoption. Addressing these challenges requires interdisciplinary collaboration between engineers, scientists, and clinicians, as well as continued investment in research and development. The future of surgical micro-robotics is bright, with the potential to revolutionize diagnostics, targeted drug delivery, and minimally invasive surgery. The integration of AI and ML will play a crucial role in enabling autonomous control and real-time image processing, paving the way for next-generation medical procedures.
References
[1] Kummer, M. P., Abbott, J. J., Kratochvil, B. E., Volgyesi, P., Errani, R., Nelson, B. J., & Sitti, M. (2010). Octomag: an electromagnetic system for precise wireless manipulation of magnetic microdevices. IEEE Transactions on Robotics, 26(6), 1006-1017.
[2] Mahoney, A. W., Patel, R. V., & Nikumb, S. V. (2013). Miniature mobile robot with magnetic adhesion and actuation. IEEE/ASME Transactions on Mechatronics, 19(2), 645-654.
[3] Nair, L. S., & Laurencin, C. T. (2007). Biodegradable polymers as biomaterials. Progress in Polymer Science, 32(8-9), 762-798.
[4] Khalil, H. K. (2002). Nonlinear systems. Prentice hall.
This report highlights exciting possibilities. The discussion of in-vivo imaging modalities is particularly interesting. Integrating AI for real-time image processing could significantly improve a surgeon’s ability to navigate and precisely target areas during minimally invasive procedures.
Thanks for your comment! The potential for AI to enhance surgical precision through real-time imaging is definitely a game-changer. Further development in AI algorithms will be key to improving accuracy and efficiency of the robotic surgery. This has the potential to improve patient outcomes in the future!
Editor: MedTechNews.Uk
Thank you to our Sponsor Esdebe