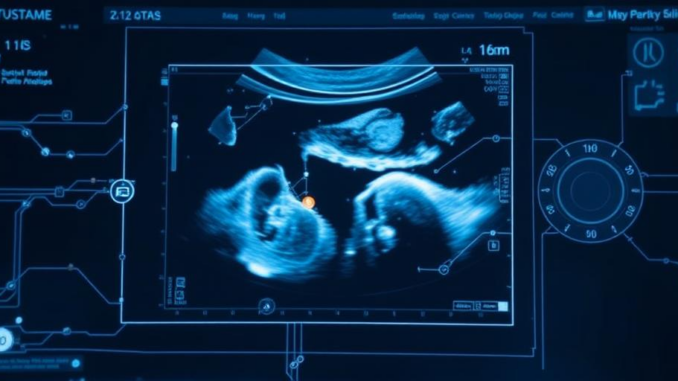
Abstract
Ultrasound technology has revolutionized medical diagnostics and therapeutics due to its non-invasive nature, real-time imaging capabilities, and relative cost-effectiveness. This report provides a comprehensive overview of ultrasound technology, exploring its fundamental principles, diverse applications across various medical specialties, and the latest advancements driving innovation in the field. We delve into the underlying physics of ultrasound wave generation, propagation, and interaction with biological tissues, examining different imaging modalities like B-mode, Doppler, and elastography. Furthermore, we analyze the therapeutic applications of ultrasound, with a particular focus on High-Intensity Focused Ultrasound (HIFU) for targeted tissue ablation and drug delivery. The report also addresses safety considerations, potential side effects, and the ongoing efforts to enhance ultrasound devices and protocols. By comparing ultrasound-based therapies with other non-invasive and minimally invasive techniques, this report aims to provide a holistic understanding of the current state and future directions of ultrasound technology in modern medicine.
Many thanks to our sponsor Esdebe who helped us prepare this research report.
1. Introduction
Ultrasound, employing sound waves with frequencies exceeding the upper limit of human hearing, has become an indispensable tool in modern medicine. Its applications span a broad spectrum, from diagnostic imaging to therapeutic interventions, offering a non-invasive and versatile approach to patient care. The technology’s evolution from simple A-mode displays to sophisticated 3D and 4D imaging modalities has significantly enhanced diagnostic accuracy and clinical decision-making. Beyond imaging, ultrasound’s therapeutic potential has been harnessed in techniques like lithotripsy, physiotherapy, and, most notably, High-Intensity Focused Ultrasound (HIFU). This report aims to provide a comprehensive overview of ultrasound technology, encompassing its fundamental principles, diverse applications, recent advancements, and future prospects.
Many thanks to our sponsor Esdebe who helped us prepare this research report.
2. Fundamental Principles of Ultrasound
2.1. Ultrasound Wave Generation and Propagation
Ultrasound waves are generated through the piezoelectric effect, where certain materials, typically crystals or ceramics, produce an electrical charge when subjected to mechanical stress and, conversely, deform when an electrical field is applied. Diagnostic ultrasound typically operates in the frequency range of 2-18 MHz, while therapeutic applications can utilize frequencies from hundreds of kHz to several MHz. The frequency of the ultrasound wave is inversely proportional to its wavelength, influencing both image resolution and penetration depth. Higher frequencies offer better resolution but are more readily attenuated by tissue, limiting penetration depth, while lower frequencies provide greater penetration at the expense of resolution.
Once generated, ultrasound waves propagate through biological tissues via compression and rarefaction cycles. The acoustic impedance of a tissue, defined as the product of its density and the speed of sound within it, determines the degree of reflection, refraction, and scattering that occurs at tissue interfaces. Differences in acoustic impedance are crucial for generating ultrasound images, as reflected waves (echoes) are detected by the transducer and processed to create a visual representation of the underlying tissue structures.
2.2. Ultrasound Interactions with Biological Tissues
The interaction of ultrasound waves with biological tissues involves several key phenomena, including reflection, refraction, scattering, and absorption. Reflection occurs at interfaces with significant differences in acoustic impedance, providing the basis for visualizing anatomical structures. Refraction, the bending of ultrasound waves as they pass from one medium to another, can introduce artifacts in ultrasound images if not properly accounted for. Scattering, a diffuse reflection of ultrasound waves in multiple directions, arises from small structures or inhomogeneities within the tissue. Absorption, the conversion of ultrasound energy into heat, contributes to attenuation and is exploited in therapeutic applications like HIFU.
2.3. Ultrasound Imaging Modalities
Several ultrasound imaging modalities have been developed to provide diverse information about tissue structure and function. The most common include:
- B-mode (Brightness Mode): This is the standard 2D ultrasound imaging mode, where the brightness of each pixel corresponds to the intensity of the reflected ultrasound wave. It provides real-time anatomical information and is widely used for various diagnostic purposes.
- Doppler Ultrasound: This technique utilizes the Doppler effect to measure the velocity of moving fluids, primarily blood. It is essential for assessing blood flow in arteries and veins, identifying vascular abnormalities, and evaluating cardiac function. Color Doppler displays blood flow direction and velocity superimposed on a B-mode image.
- Color Doppler: Visual representation of flow direction and velocity, superimposed on a B-mode image.
- Power Doppler: More sensitive to low-velocity flow than color Doppler, but provides no directional information.
- Elastography: This technique measures the stiffness or elasticity of tissues, which can be indicative of various pathological conditions, such as tumors or fibrosis. Elastography can be performed using different methods, including strain elastography (measuring tissue deformation under external compression) and shear wave elastography (measuring the propagation speed of shear waves).
- Contrast-Enhanced Ultrasound (CEUS): Involves the injection of microbubble contrast agents into the bloodstream to enhance the visualization of blood vessels and tissue perfusion. CEUS is particularly useful for evaluating tumors, detecting liver lesions, and assessing myocardial perfusion.
- 3D and 4D Ultrasound: These modalities allow for the reconstruction of 3D volumes from a series of 2D ultrasound images. 4D ultrasound adds a temporal dimension, enabling real-time visualization of moving structures in three dimensions. These techniques are commonly used in obstetrics to visualize fetal anatomy and movement.
Many thanks to our sponsor Esdebe who helped us prepare this research report.
3. Therapeutic Applications of Ultrasound
3.1. High-Intensity Focused Ultrasound (HIFU)
HIFU is a non-invasive therapeutic technique that uses focused ultrasound energy to generate localized heating and tissue ablation. By focusing a high-intensity ultrasound beam onto a specific target within the body, HIFU can precisely destroy diseased tissue while sparing surrounding healthy tissue. The mechanism of action involves rapid temperature elevation (typically to 60-90°C) at the focal point, leading to coagulative necrosis. HIFU is guided by real-time imaging, such as ultrasound or MRI, allowing for precise targeting and monitoring of the treatment.
3.2. HIFU Mechanisms of Action
The therapeutic effects of HIFU are primarily mediated by thermal ablation, but mechanical effects also contribute to tissue destruction. The rapid temperature elevation at the focal point causes protein denaturation, cell membrane disruption, and irreversible tissue damage. In addition, cavitation, the formation and collapse of microbubbles within the tissue, can generate mechanical stress and further contribute to cell death. Furthermore, HIFU can induce a cascade of biological responses, including inflammation, immune activation, and tissue remodeling.
3.3. Applications of HIFU
HIFU has emerged as a promising treatment option for various medical conditions, including:
- Prostate Cancer: HIFU is used as a primary or salvage therapy for localized prostate cancer. It offers a non-invasive alternative to radical prostatectomy or radiation therapy, with the potential for fewer side effects.
- Kidney Cancer: HIFU can be used to ablate small renal tumors in patients who are not candidates for surgery.
- Liver Cancer: HIFU is employed to treat hepatocellular carcinoma (HCC) and liver metastases, either alone or in combination with other therapies.
- Uterine Fibroids: HIFU is a non-surgical option for treating symptomatic uterine fibroids, offering relief from heavy bleeding and pelvic pain.
- Bone Tumors: HIFU can be used to palliate pain and control tumor growth in patients with bone metastases.
- Essential Tremor: Focused ultrasound thalamotomy (FUS) is approved for treating essential tremor by creating a small lesion in the thalamus, the brain region responsible for tremor control. The procedure is performed under MRI guidance, allowing for real-time monitoring of the temperature and location of the lesion.
- Parkinson’s Disease: Research is being done to evaluate the effectiveness of focused ultrasound for the treatment of the symptoms of Parkinson’s Disease.
- Cerebral Palsy: As mentioned in the original article, HIFU is being explored as a potential treatment to improve motor function in cerebral palsy patients by selectively ablating overactive muscles.
3.4. Other Therapeutic Ultrasound Applications
Besides HIFU, other therapeutic ultrasound techniques are used in various medical fields:
- Lithotripsy: Uses focused shock waves to break down kidney stones into smaller fragments that can be passed more easily.
- Physiotherapy: Low-intensity ultrasound is used to promote tissue healing, reduce pain, and improve range of motion in musculoskeletal conditions.
- Drug Delivery: Ultrasound can enhance drug delivery to specific tissues by increasing cell membrane permeability or disrupting blood-tissue barriers. This technique is being explored for cancer therapy and gene therapy.
- Sonothrombolysis: Ultrasound is used to dissolve blood clots in arteries and veins, improving blood flow and preventing tissue damage.
- Wound Healing: Low-intensity ultrasound can stimulate wound healing by promoting cell proliferation, angiogenesis, and collagen synthesis.
Many thanks to our sponsor Esdebe who helped us prepare this research report.
4. Safety and Side Effects
Ultrasound is generally considered a safe imaging modality when used appropriately. However, potential side effects can occur, particularly with therapeutic applications involving high-intensity ultrasound. The most common side effects include skin burns, pain, and damage to surrounding tissues. Careful patient selection, proper treatment planning, and real-time monitoring are essential to minimize the risk of adverse events.
Specific side effects associated with HIFU vary depending on the treated organ. For example, HIFU for prostate cancer can cause urinary incontinence, erectile dysfunction, and rectal injury. HIFU for liver cancer can lead to liver damage, bile duct injury, and skin burns. Focused ultrasound thalamotomy for essential tremor can result in gait disturbances, sensory deficits, and cognitive changes.
The use of contrast agents in CEUS can occasionally cause allergic reactions, ranging from mild skin rashes to severe anaphylaxis. The risk of contrast-related adverse events is generally low, but patients with a history of allergies or kidney disease should be carefully screened before CEUS.
Many thanks to our sponsor Esdebe who helped us prepare this research report.
5. Technological Advancements in Ultrasound Devices
Significant advancements in ultrasound technology have led to improved image quality, enhanced functionality, and expanded clinical applications. Some of the key advancements include:
- High-Frequency Transducers: These transducers offer improved resolution for imaging superficial structures, such as skin, tendons, and small vessels.
- Matrix Array Transducers: These transducers allow for electronic beam steering and focusing in three dimensions, enabling real-time 3D imaging and improved spatial resolution.
- Harmonic Imaging: This technique uses the harmonic frequencies generated by tissues when exposed to ultrasound waves, resulting in improved image quality and reduced artifacts.
- Elastography Techniques: Advancements in elastography have led to more accurate and reproducible measurements of tissue stiffness, improving the diagnosis and monitoring of various diseases.
- Contrast-Enhanced Ultrasound (CEUS): The development of new microbubble contrast agents with improved stability and imaging properties has expanded the applications of CEUS in various medical fields.
- Miniaturized Ultrasound Devices: The development of handheld and portable ultrasound devices has enabled point-of-care imaging and expanded access to ultrasound technology in resource-limited settings.
- Artificial Intelligence (AI) in Ultrasound: AI algorithms are being developed to automate image analysis, improve diagnostic accuracy, and personalize treatment planning in ultrasound applications. AI can assist in tasks such as lesion detection, image segmentation, and automated measurements.
Many thanks to our sponsor Esdebe who helped us prepare this research report.
6. Comparison with Other Treatment Modalities
Ultrasound-based therapies, particularly HIFU, offer several advantages over other non-invasive or minimally invasive treatment modalities. Compared to surgery, HIFU is non-invasive, requires no incisions, and has a shorter recovery time. Compared to radiation therapy, HIFU does not involve ionizing radiation and can be repeated multiple times if necessary. Compared to radiofrequency ablation (RFA) or microwave ablation (MWA), HIFU offers more precise targeting and can treat larger volumes of tissue.
However, ultrasound-based therapies also have some limitations compared to other modalities. HIFU can be more expensive than some other treatments, and its availability may be limited to specialized centers. The effectiveness of HIFU can be affected by factors such as tissue density, bone interference, and patient body habitus. In some cases, surgery or radiation therapy may be more appropriate for achieving complete tumor ablation or controlling disease progression.
For example, in the treatment of prostate cancer, HIFU may be preferred for patients with localized disease who are not candidates for surgery or radiation therapy. However, radical prostatectomy may be more appropriate for patients with high-risk disease or those who desire a more definitive treatment. Similarly, in the treatment of uterine fibroids, HIFU may be a good option for women who wish to avoid surgery or are not candidates for uterine artery embolization (UAE). However, hysterectomy may be necessary for women with large or multiple fibroids.
Many thanks to our sponsor Esdebe who helped us prepare this research report.
7. Future Directions
The field of ultrasound technology is rapidly evolving, with ongoing research and development focused on enhancing image quality, expanding therapeutic applications, and improving patient outcomes. Some of the key future directions include:
- Development of new ultrasound contrast agents: Researchers are working on developing new contrast agents with improved targeting capabilities, longer circulation times, and enhanced imaging properties.
- Integration of AI and machine learning: AI algorithms are being developed to automate image analysis, improve diagnostic accuracy, and personalize treatment planning in ultrasound applications.
- Development of new HIFU devices: Researchers are working on developing more advanced HIFU devices with improved targeting precision, real-time feedback control, and integrated imaging capabilities.
- Expanding the applications of HIFU: HIFU is being explored for the treatment of a wider range of medical conditions, including cardiovascular disease, neurological disorders, and autoimmune diseases.
- Development of combination therapies: Ultrasound-based therapies are being combined with other treatment modalities, such as chemotherapy, immunotherapy, and radiation therapy, to enhance therapeutic efficacy.
- Personalized Ultrasound Therapy: The future of ultrasound therapy lies in personalized medicine, where treatment protocols are tailored to individual patient characteristics and disease characteristics. This will involve integrating advanced imaging techniques, genetic profiling, and computational modeling to optimize treatment planning and predict patient outcomes.
Many thanks to our sponsor Esdebe who helped us prepare this research report.
8. Conclusion
Ultrasound technology has become an indispensable tool in modern medicine, offering a versatile and non-invasive approach to diagnostic imaging and therapeutic interventions. The ongoing advancements in ultrasound devices, imaging modalities, and therapeutic techniques are expanding the clinical applications of ultrasound and improving patient outcomes. HIFU, in particular, has emerged as a promising treatment option for various medical conditions, offering a non-invasive alternative to surgery or radiation therapy. As technology continues to advance, ultrasound will play an increasingly important role in the future of medicine, contributing to improved diagnosis, targeted therapies, and personalized patient care.
Many thanks to our sponsor Esdebe who helped us prepare this research report.
References
- ter Haar, G. (2007). Therapeutic ultrasound. European Journal of Ultrasound, 25(1), 1-6.
- Kennedy, J. E. (2005). High-intensity focused ultrasound in the treatment of solid tumours. Nature Reviews Cancer, 5(4), 321-328.
- Duck, F. A. (Ed.). (2015). Physical properties of tissue: a comprehensive reference book. Academic Press.
- Szabo, T. L. (2004). Diagnostic ultrasound imaging: inside out. Academic Press.
- Barnett, S. B., ter Haar, G. R., Ziskin, M. C., Nyborg, W. L., Maeda, K., Bang, J., & Lyons, E. A. (2000). International recommendations and guidelines for the safe use of diagnostic ultrasound in medicine. Ultrasound in Medicine & Biology, 26(3), 355-366.
- Rivens, I., & Jolesz, F. A. (2007). Image-guided focused ultrasound surgery. Journal of Magnetic Resonance Imaging, 26(5), 1088-1099.
- Wu, F., Wang, Z. B., Chen, W. Z., Bai, J., Zou, J. Z., Li, K. Q., … & Gao, Y. F. (2004). Extracorporeal high intensity focused ultrasound ablation in the treatment of patients with large hepatocellular carcinoma. Annals of Surgical Oncology, 11(12), 1061-1069.
- Illing, R. O., Kennedy, J. E., Wu, F., ter Haar, G. R., & Protheroe, A. S. (2005). The safety and feasibility of extracorporeal high-intensity focused ultrasound (HIFU) for the treatment of liver tumours. British Journal of Cancer, 93(11), 1236-1242.
- European Federation of Societies for Ultrasound in Medicine and Biology (EFSUMB). (2008). Guidelines and Recommendations for Clinical Use of Ultrasound Contrast Agents (Update 2008). Ultraschall in der Medizin-European Journal of Ultrasound, 29(03), 204-218.
- McDannold, N., Arvanitis, C. D., Vykhodtseva, N., & Livingstone, M. S. (2010). Temporary disruption of the blood–brain barrier by use of ultrasound and microbubbles: safety and efficacy evaluation in rhesus monkeys. Radiology, 256(1), 245-255.
- Aubry, J. F., Tanter, M., Pernot, M., Marquet, F., Marsac, L., & Fink, M. (2003). Temporal decorrelation of speckle pattern for ultrasound elastography. Ultrasonic Imaging, 25(4), 289-299.
- Ranjan Pushparajah, Vivek Mendiratta, Manojkumar Gopu, Anjan K Das, Binit Sureka, High Intensity Focused Ultrasound (HIFU): A Review of the Technology, Applications, and Safety, Hindawi Journal of Healthcare Engineering, Volume 2022, Article ID 1595491, 14 pages
- Lipsman N, Schwartz ML, Huang Y, et al. MR-guided focused ultrasound thalamotomy for essential tremor: a proof-of-concept study. Lancet Neurol. 2013;12(2):139-147. doi:10.1016/S1474-4422(12)70267-5
- Sinai Ali Mansoori, Morteza Akbari, Seyed Hamed Hosseini, et al. High-Intensity Focused Ultrasound for the Treatment of Parkinson’s Disease: Current Progress and Future Perspectives. J Parkinsons Dis. 2023; 13(7): 1107–1120. Published online 2023 Aug 16. doi: 10.3233/JPD-230080
The mention of AI in ultrasound is intriguing. Could AI-driven analysis of ultrasound data lead to earlier, more accurate diagnoses, potentially impacting treatment strategies and improving patient outcomes?
That’s a great point! AI’s potential to enhance diagnostic accuracy is significant. Imagine AI algorithms identifying subtle anomalies in ultrasound images that might be missed by the human eye, leading to earlier interventions and improved results. The possibilities are exciting!
Editor: MedTechNews.Uk
Thank you to our Sponsor Esdebe
The discussion on combining ultrasound with other therapies is compelling. Exploring synergistic effects, such as combining HIFU with immunotherapy to stimulate a more robust immune response against tumors, seems particularly promising for future research.