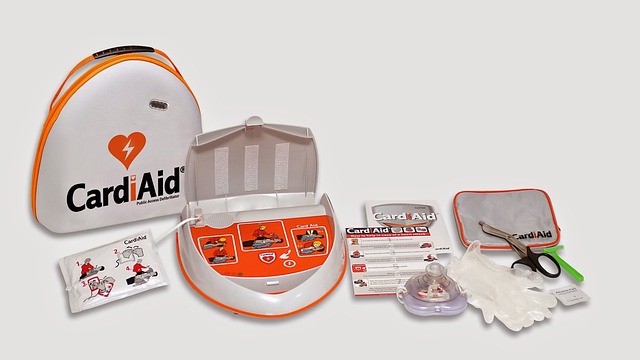
Advancements and Challenges in Defibrillation Therapy: A Comprehensive Review
Abstract
Sudden cardiac death (SCD) remains a significant global health challenge, and defibrillation therapy, particularly using implantable cardioverter-defibrillators (ICDs), is a cornerstone of its prevention. This review provides a comprehensive overview of defibrillation, encompassing its mechanisms of action, various device types and configurations (transvenous, subcutaneous, leadless), evolving indications based on risk stratification, advanced programming strategies to minimize inappropriate shocks, the critical issue of battery longevity, and the multifaceted spectrum of complications, including lead-related failures, infections, and inappropriate therapy delivery. We delve into recent advancements in lead technology, exploring both incremental improvements and radical departures from traditional designs. Furthermore, we critically assess the contemporary challenges facing the field, such as the need for improved risk prediction models, the impact of new antiarrhythmic drugs and ablation techniques, and the optimization of device therapy in the context of heart failure and other comorbidities. The future directions of defibrillation therapy are also discussed, including novel energy delivery methods, enhanced sensing algorithms, and the integration of artificial intelligence to personalize device management.
1. Introduction
Sudden cardiac death (SCD), most commonly due to ventricular arrhythmias, poses a significant threat to global health. Defibrillation, the delivery of a controlled electrical shock to the heart, remains the most effective intervention for terminating these life-threatening arrhythmias. The advent of implantable cardioverter-defibrillators (ICDs) has revolutionized the prevention of SCD, providing continuous monitoring and immediate therapy delivery. However, ICD therapy is not without its limitations. The choice of device type, careful patient selection, meticulous device programming, and the potential for complications all necessitate a nuanced understanding of the field. This review aims to provide a comprehensive overview of defibrillation therapy, exploring its evolution, current state, and future directions, with a particular focus on advancements and ongoing challenges.
2. Mechanisms of Defibrillation
The fundamental principle of defibrillation is to deliver an electrical shock that depolarizes a critical mass of myocardial tissue, effectively terminating the chaotic electrical activity characteristic of ventricular fibrillation (VF) or rapid ventricular tachycardia (VT). The precise mechanisms underlying successful defibrillation are complex and not fully elucidated, but several key factors are believed to be involved. These include the critical mass hypothesis, the upper limit of vulnerability (ULV) phenomenon, and the role of myocardial refractoriness.
The critical mass hypothesis posits that successful defibrillation requires the simultaneous depolarization of a sufficient proportion of myocardial cells to extinguish the arrhythmogenic wavefront. The size and location of this critical mass depend on various factors, including the underlying substrate, the shock vector, and the waveform characteristics.
The upper limit of vulnerability (ULV) refers to the maximum shock strength that, when delivered during a vulnerable period of the cardiac cycle, will successfully terminate the arrhythmia without inducing a new one. Understanding the ULV is crucial for optimizing shock efficacy and minimizing the risk of proarrhythmia.
Myocardial refractoriness, the period during which myocardial cells are unresponsive to stimulation, also plays a significant role. The effectiveness of defibrillation depends on the ability of the shock to depolarize enough cells that are not refractory, thereby interrupting the re-entrant circuits that sustain the arrhythmia. Differences in the refractory periods and conduction velocities across the myocardium contribute to the complexity of defibrillation.
Advancements in computational modeling and experimental studies have shed light on the intricate interplay of these factors. For example, research has shown that the success of defibrillation is not solely determined by the total energy delivered but also by the waveform shape, pulse duration, and polarity of the shock [1]. Biphasic waveforms, which deliver current in two phases, have been shown to be more effective than monophasic waveforms, requiring lower energy levels and resulting in less myocardial damage [2].
3. Types of Implantable Cardioverter-Defibrillators (ICDs)
ICDs have evolved significantly since their introduction, with various types available to suit different patient needs and anatomical considerations. The primary classifications are based on lead configuration: transvenous ICDs (TV-ICDs), subcutaneous ICDs (S-ICDs), and leadless ICDs.
3.1 Transvenous ICDs (TV-ICDs)
TV-ICDs are the traditional and most widely used type of ICD. They involve the placement of one or more leads through the venous system into the right atrium, right ventricle, and/or coronary sinus (for left ventricular pacing). These leads serve both sensing and pacing/defibrillation functions. TV-ICDs offer the capability of antitachycardia pacing (ATP) for terminating slower VTs, which is a significant advantage in reducing the frequency of painful shocks. Furthermore, dual-chamber and biventricular TV-ICDs can provide rate-adaptive pacing and cardiac resynchronization therapy (CRT), respectively, addressing bradycardia and heart failure symptoms in addition to SCD prevention. However, TV-ICDs are associated with potential lead-related complications, including lead dislodgement, fracture, insulation breaches, and venous thrombosis [3]. Lead extraction, often necessary to address these complications or infections, carries inherent risks.
3.2 Subcutaneous ICDs (S-ICDs)
The S-ICD represents a significant advancement, avoiding the need for intravascular leads. The S-ICD lead is placed subcutaneously along the left sternal border and lateral chest wall, with the pulse generator implanted in the left axillary region. The S-ICD senses the cardiac electrical activity and delivers defibrillation shocks without directly contacting the heart. This approach eliminates the risk of venous thrombosis, perforation, and endocarditis associated with TV-ICD leads. However, S-ICDs lack pacing capabilities, meaning they cannot deliver ATP or provide bradycardia pacing. Furthermore, the sensing algorithm of the S-ICD may be less specific than that of TV-ICDs, potentially leading to a higher risk of inappropriate shocks, particularly due to T-wave oversensing [4]. Newer S-ICD models have incorporated enhanced sensing algorithms to mitigate this risk.
3.3 Leadless ICDs
Leadless ICDs represent the newest frontier in defibrillation therapy. These devices are self-contained units implanted directly into the right ventricle via a femoral vein approach, eliminating the need for any leads. Currently, only single chamber ventricular sensing and defibrillation is available. The elimination of leads theoretically reduces the risk of lead-related complications. However, leadless ICDs have limitations, including the lack of pacing capabilities, a larger device size, and the need for a more complex implantation procedure. Early clinical experience with leadless ICDs has been promising, but long-term data and comparative studies are needed to fully assess their safety and efficacy [5].
4. Indications for ICD Implantation
ICD implantation is indicated for both primary and secondary prevention of SCD. Primary prevention refers to the implantation of an ICD in patients who have not experienced a life-threatening arrhythmia but are at high risk for developing one. Secondary prevention involves ICD implantation in patients who have already survived a cardiac arrest or sustained VT/VF.
4.1 Primary Prevention
The primary prevention indications for ICD implantation are primarily based on the presence of structural heart disease and reduced left ventricular ejection fraction (LVEF). Major clinical trials, such as MADIT-II and SCD-HeFT, have demonstrated the benefit of ICD therapy in patients with ischemic or non-ischemic cardiomyopathy and an LVEF of 30% or less [6, 7]. The current guidelines recommend ICD implantation for primary prevention in patients with LVEF ≤35% and NYHA class II or III heart failure, despite optimal medical therapy [8].
However, the decision to implant an ICD for primary prevention is not always straightforward. The benefits of ICD therapy must be weighed against the risks of complications and inappropriate shocks. Furthermore, some patients with low LVEF may not benefit from ICD therapy due to advanced age, significant comorbidities, or a low likelihood of surviving a shockable event. Risk stratification remains an area of ongoing research, with efforts focused on identifying additional predictors of SCD risk, such as microvolt T-wave alternans, heart rate variability, and late gadolinium enhancement on cardiac MRI [9].
4.2 Secondary Prevention
ICD implantation is strongly recommended for secondary prevention in patients who have survived a cardiac arrest due to VT/VF or who have sustained symptomatic VT. These patients are at very high risk for recurrent arrhythmias and SCD. The AVID trial demonstrated a significant survival benefit with ICD therapy compared to antiarrhythmic drug therapy in this population [10]. The presence of reversible causes for the initial arrhythmia, such as electrolyte imbalances or drug toxicity, should be carefully evaluated and addressed before considering ICD implantation.
5. ICD Programming
ICD programming plays a crucial role in optimizing device performance and minimizing the risk of inappropriate shocks. The primary programming parameters include detection criteria (heart rate cutoffs, morphology algorithms), therapy settings (ATP sequences, shock energy levels), and pacing modes. The goal of ICD programming is to accurately detect and terminate life-threatening arrhythmias while avoiding unnecessary interventions.
5.1 Detection Criteria
The detection criteria determine when the ICD will recognize an arrhythmia and initiate therapy. Setting the heart rate cutoffs too low can lead to inappropriate detection of sinus tachycardia or supraventricular tachycardia (SVT). Morphology algorithms, which analyze the shape of the QRS complex, can help differentiate between VT and SVT. However, morphology algorithms are not always perfect and can be influenced by factors such as conduction abnormalities and heart rate variability. Recent advancements include dual chamber discrimination algorithms, improved VT/SVT discrimination using probability density functions and more sophisticated pattern recognition techniques.
5.2 Therapy Settings
ATP is a valuable feature of TV-ICDs that can terminate slower VTs without delivering a shock. ATP involves the delivery of a series of rapid pacing pulses that overdrive the arrhythmia. ATP is typically programmed as the first-line therapy for slower VTs, with shocks reserved for faster or refractory arrhythmias. The shock energy levels should be programmed to the lowest effective level to minimize myocardial damage and discomfort. Biphasic waveforms have allowed for lower energy shocks while maintaining efficacy. Step-up programming, where the shock energy is gradually increased if the initial shock is unsuccessful, can also be used to optimize energy delivery.
5.3 Pacing Modes
In patients with bradycardia or heart failure, pacing modes can be programmed to provide rate support or cardiac resynchronization therapy (CRT). Rate-adaptive pacing adjusts the pacing rate based on the patient’s activity level, while CRT coordinates the contraction of the left and right ventricles to improve cardiac function. Dual-chamber ICDs can provide both atrial and ventricular pacing, while biventricular ICDs deliver pacing pulses to both ventricles.
5.4 Optimizing Programming to Reduce Inappropriate Shocks
Inappropriate shocks are a major concern with ICD therapy, leading to anxiety, pain, and reduced quality of life. Several strategies can be employed to minimize the risk of inappropriate shocks, including: higher heart rate cutoffs, morphology algorithms, ATP as the first-line therapy, and careful consideration of underlying SVT mechanisms. The SMART trial demonstrated that tailored ICD programming based on individual patient characteristics can significantly reduce the incidence of inappropriate shocks [11].
6. Battery Life and Device Longevity
ICD battery life is a critical consideration, as battery depletion necessitates device replacement. Battery longevity is influenced by several factors, including the frequency of shocks delivered, the pacing burden, and the device programming parameters. Frequent shocks significantly deplete the battery, while ATP and pacing have a lesser impact. Modern ICDs typically have a battery life of 5-7 years, but this can vary depending on usage patterns. Regular device interrogation is essential to monitor battery voltage and ensure timely device replacement. Remote monitoring systems can facilitate frequent device checks and provide early warning of battery depletion [12].
7. Complications of ICD Therapy
ICD therapy is associated with a variety of potential complications, including lead-related issues, infections, inappropriate shocks, and device malfunction. The risk of complications varies depending on the type of device, the patient’s underlying health status, and the experience of the implanting physician.
7.1 Lead-Related Issues
Lead-related complications are a major concern with TV-ICDs. These complications include lead dislodgement, fracture, insulation breaches, and venous thrombosis. Lead dislodgement can result in loss of sensing or pacing function, while lead fracture or insulation breaches can lead to inappropriate shocks or failure to deliver therapy. Venous thrombosis can cause arm swelling and pain. Lead extraction, often necessary to address these complications, carries inherent risks, including vascular injury, cardiac perforation, and death [13].
7.2 Infections
Infections are another serious complication of ICD therapy. Device infections can range from localized pocket infections to systemic infections involving the heart valves (endocarditis). Device infections typically require complete device removal and antibiotic therapy. Prevention strategies include meticulous sterile technique during implantation, prophylactic antibiotics, and careful management of wound care. S-ICDs have a lower risk of endocarditis compared to TV-ICDs due to the absence of intravascular leads [14].
7.3 Inappropriate Shocks
Inappropriate shocks, as previously mentioned, are a significant concern for patients receiving ICD therapy. Inappropriate shocks can be triggered by sinus tachycardia, SVT, or T-wave oversensing. Careful device programming and optimization of sensing algorithms are essential to minimize the risk of inappropriate shocks. Patient education and psychological support are also important in managing the anxiety and distress associated with inappropriate shocks.
7.4 Device Malfunction
Device malfunction can occur due to battery depletion, component failure, or programming errors. Regular device interrogation is essential to detect and address any device malfunctions promptly. Remote monitoring systems can facilitate early detection of device malfunctions and allow for timely intervention.
8. Contemporary Challenges and Future Directions
Despite the significant advances in defibrillation therapy, several challenges remain. One major challenge is the need for improved risk prediction models to identify patients who are most likely to benefit from ICD therapy. Current risk stratification strategies based on LVEF and NYHA class have limitations, and additional predictors of SCD risk are needed. Another challenge is the management of patients with complex comorbidities, such as heart failure, atrial fibrillation, and renal dysfunction. These patients often have a higher risk of complications and require individualized treatment strategies. The role of S-ICD and leadless ICDs in specific patient populations also warrants further investigation. Clinical trials comparing these devices to TV-ICDs are needed to determine their relative safety and efficacy.
Future directions in defibrillation therapy include the development of novel energy delivery methods, such as low-energy defibrillation and high-frequency chest wall oscillation [15]. These techniques aim to reduce the myocardial damage and discomfort associated with traditional defibrillation shocks. Enhancements to sensing algorithms using artificial intelligence and machine learning could improve the accuracy of arrhythmia detection and reduce the risk of inappropriate shocks [16]. Closed-loop ICD systems that automatically adjust therapy based on real-time physiological data are also under development. These systems could potentially optimize therapy delivery and improve patient outcomes. Personalized medicine approaches that tailor ICD programming and management based on individual patient characteristics are also gaining traction. Integration of genetic information, biomarkers, and imaging data could help identify patients who are most likely to benefit from specific ICD therapies. Finally, improved lead technology focusing on increased durability, reduced thrombogenicity, and enhanced biocompatibility are crucial to minimize lead-related complications and extend device longevity.
9. Conclusion
Defibrillation therapy has transformed the management of SCD, and ICDs have become a life-saving intervention for many patients. However, ICD therapy is not without its challenges, and ongoing research is focused on addressing these challenges and further improving the safety and efficacy of defibrillation therapy. Improved risk stratification, optimized device programming, novel energy delivery methods, and personalized medicine approaches hold promise for enhancing the outcomes of patients receiving ICD therapy. Continued innovation in lead technology is essential to minimize lead-related complications and extend device longevity. As the field of defibrillation therapy continues to evolve, it is crucial to remain vigilant in addressing the ongoing challenges and embracing new advancements to improve the lives of patients at risk for SCD.
References
[1] Ideker RE, et al. The relationship between shock polarity, shape, and location on the success of defibrillation. Circulation. 1998;97(25):2585-2590.
[2] Bardy GH, et al. Multicenter experience with implantable cardioverter-defibrillators using biphasic waveforms. Circulation. 1996;94(5):945-952.
[3] Wilkoff BL, et al. Complications of implantable cardioverter-defibrillator therapy: a prospective, randomized, multicenter trial. J Am Coll Cardiol. 2004;43(6):1003-1010.
[4] Gold MR, et al. Primary results of the subcutaneous implantable cardioverter-defibrillator trial. Circulation. 2013;128(9):941-948.
[5] Reddy VY, et al. Percutaneous Implantation of an Entirely Intracardiac Leadless Pacemaker. N Engl J Med. 2015;373(12):1125-1135.
[6] Moss AJ, et al. Prophylactic implantation of a defibrillator in patients with myocardial infarction and low ejection fraction. N Engl J Med. 2002;346(12):877-883.
[7] Kadish A, et al. Prophylactic defibrillator implantation in patients with nonischemic dilated cardiomyopathy. N Engl J Med. 2004;350(21):2151-2158.
[8] Al-Khatib SM, et al. 2017 AHA/ACC/HRS guideline for management of patients with ventricular arrhythmias and the prevention of sudden cardiac death: A Report of the American College of Cardiology/American Heart Association Task Force on Clinical Practice Guidelines and the Heart Rhythm Society. Circulation. 2018;138(13):e272-e391.
[9] Piccini JP, et al. Risk stratification for sudden cardiac death. Circulation. 2013;128(16):1756-1765.
[10] The Antiarrhythmics Versus Implantable Defibrillators (AVID) Investigators. A comparison of antiarrhythmic-drug therapy with implantable defibrillators in patients at high risk for sudden death. N Engl J Med. 1997;337(22):1576-1583.
[11] Gasparini M, et al. Long-term comparison of conventional and optimized dual-chamber implantable cardioverter-defibrillator programming: the ADVANCE III trial. Circulation. 2009;120(25):2565-2573.
[12] Crossley GH, et al. Benefits of remote monitoring in implantable cardioverter-defibrillator patients. J Am Coll Cardiol. 2011;57(19):2029-2035.
[13] Klug D, et al. Lead extraction in the modern era–indications, techniques, and results. Europace. 2008;10(3):291-300.
[14] Weiss R, et al. Safety and efficacy of a totally subcutaneous implantable cardioverter-defibrillator. Circulation. 2013;128(9):934-940.
[15] Neumar RW, et al. 2015 American Heart Association Guidelines Update for Cardiopulmonary Resuscitation and Emergency Cardiovascular Care. Circulation. 2015;132(18 Suppl 2):S315-S367.
[16] Rajkomar A, et al. Artificial intelligence in healthcare. Nat Rev Clin Oncol. 2019;16(10):599-614.
Given the discussion on battery longevity, what advancements are being made in energy harvesting technologies to potentially prolong the lifespan of implantable cardioverter-defibrillators, and how might these impact device size and patient comfort?
That’s a great point! The developments in energy harvesting, like piezoelectric or biomechanical methods, are exciting. If successful, these could dramatically extend ICD battery life, potentially reducing the frequency of device replacements. The impact on device size is a crucial consideration – we’re aiming for smaller, more comfortable devices for patients.
Editor: MedTechNews.Uk
Thank you to our Sponsor Esdebe
The discussion on ICD programming highlights the critical balance between accurate arrhythmia detection and avoiding inappropriate shocks. It would be interesting to explore the ethical considerations surrounding the use of AI in refining these algorithms and its potential impact on patient autonomy and trust in device therapy.
That’s an excellent point about ethical considerations! The integration of AI in ICD programming presents a fascinating challenge. Balancing enhanced accuracy with patient understanding and control is key. Transparency in how these algorithms function will be vital to maintaining trust and ensuring patient autonomy in therapy decisions. It is an important debate.
Editor: MedTechNews.Uk
Thank you to our Sponsor Esdebe
Considering the documented complications, what advancements are being made to improve the biocompatibility of ICD leads, thereby reducing the incidence of lead-related failures and the necessity for extraction procedures?
That’s a really important question! There’s a lot of exciting research focused on new lead materials and coatings designed to minimize the body’s inflammatory response. Some studies are exploring porous materials to encourage tissue ingrowth for better lead stabilization and reduced micromotion, which contributes to failure. It is a great question for discussion.
Editor: MedTechNews.Uk
Thank you to our Sponsor Esdebe
Intriguing review! Considering the push for personalized medicine, how far away are we from ICDs that learn and adapt programming *in real time* based on a patient’s unique physiology and arrhythmia patterns? Think ‘set it and forget it’ but for keeping hearts happy!
That’s a fantastic vision! The idea of ICDs dynamically adapting to a patient’s specific needs is definitely the future. AI and machine learning are paving the way, but rigorous validation and data security protocols are paramount as we move towards these ‘smart’ devices. It’s exciting to think about!
Editor: MedTechNews.Uk
Thank you to our Sponsor Esdebe