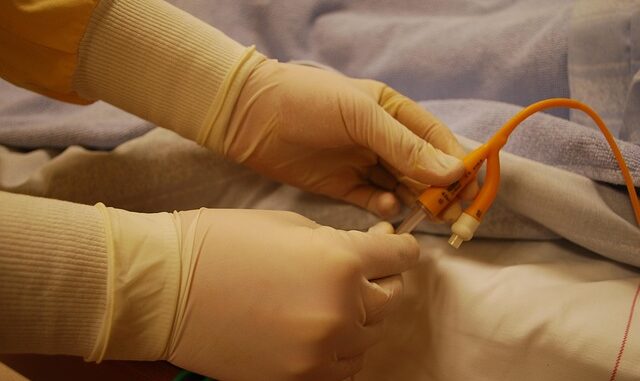
Abstract
Electrophysiology (EP) catheters are indispensable tools for diagnosing and treating cardiac arrhythmias. This report provides a comprehensive overview of the evolution, current state-of-the-art, and future directions of EP catheter technology. We delve into the design, materials, and functionality of various catheter types, including those used for mapping, ablation, and pacing. We examine advancements in catheter navigation, mapping technologies (e.g., 3D mapping systems), and energy delivery modalities (e.g., radiofrequency, cryoablation, pulsed field ablation [PFA]), with a particular focus on novel technologies like the Farapulse PFA catheter and contact force sensing catheters. The potential complications associated with catheter use are thoroughly discussed, along with strategies for minimizing these risks. Finally, we explore promising future directions in catheter development, including robotic-assisted navigation, advanced imaging integration, and personalized ablation strategies.
Many thanks to our sponsor Esdebe who helped us prepare this research report.
1. Introduction
Cardiac arrhythmias represent a significant global health burden, impacting millions worldwide and contributing to increased morbidity and mortality [1]. Electrophysiology (EP) studies and catheter ablation have emerged as cornerstone therapeutic interventions for a wide spectrum of arrhythmias, including atrial fibrillation (AF), atrial flutter, supraventricular tachycardia (SVT), and ventricular tachycardia (VT) [2]. These procedures rely heavily on the precise and effective use of EP catheters. Over the past several decades, EP catheter technology has undergone a remarkable evolution, driven by advancements in materials science, engineering, and our understanding of arrhythmia mechanisms. Early catheters were primarily diagnostic, providing limited mapping capabilities. However, modern catheters incorporate sophisticated features such as 3D mapping, contact force sensing, and diverse energy delivery modalities, enabling more precise and effective ablation procedures. This report aims to provide an in-depth review of the advancements and challenges in EP catheter technology, encompassing catheter design, materials, functionality, potential complications, and future directions. It caters to an expert audience, assuming a fundamental understanding of EP principles and catheter ablation techniques.
Many thanks to our sponsor Esdebe who helped us prepare this research report.
2. Catheter Design and Materials
The design and materials of EP catheters are critical determinants of their performance, safety, and durability. Several key factors influence catheter design, including maneuverability, steerability, visibility under fluoroscopy, biocompatibility, and the ability to transmit energy efficiently. Catheters typically consist of a flexible shaft, a distal tip containing electrodes, and a handle for manipulation. The shaft is usually constructed from biocompatible polymers such as polyurethane or polyethylene, providing flexibility and resistance to kinking. Braided stainless steel or nitinol reinforcements are often embedded within the shaft to enhance torque control and maneuverability [3].
The distal tip is the most critical component of the catheter, as it houses the electrodes responsible for recording electrical signals and delivering energy. Electrode materials commonly include platinum, platinum-iridium alloys, or gold. Platinum and platinum-iridium alloys offer a balance of electrical conductivity, biocompatibility, and durability. Gold electrodes provide excellent electrical conductivity but are more susceptible to wear. Electrode spacing and configuration influence the spatial resolution of mapping and ablation. For example, catheters with closely spaced electrodes provide higher-resolution mapping capabilities, while catheters with larger electrode surfaces deliver more efficient ablation. The introduction of irrigation channels within the distal tip has significantly improved ablation efficacy by cooling the electrode-tissue interface and preventing char formation [4].
Contact force sensing (CFS) catheters represent a significant advancement in ablation technology. These catheters incorporate miniature force sensors at the distal tip, providing real-time feedback on the force applied to the tissue during ablation. This information is crucial for achieving optimal lesion formation and minimizing the risk of complications such as perforation or steam pops. The sensors are typically based on micro-electromechanical systems (MEMS) technology, utilizing strain gauges or capacitive sensors to measure force [5].
The Farapulse PFA catheter utilizes a fundamentally different energy delivery modality: pulsed field ablation (PFA). Instead of thermal energy, PFA delivers short bursts of high-voltage electrical pulses, inducing cell death through electroporation. The Farapulse catheter employs a basket-shaped electrode array, allowing for rapid and circumferential ablation of the pulmonary veins, the primary source of AF in many patients. The non-thermal nature of PFA is believed to offer advantages in terms of safety, potentially reducing the risk of esophageal injury and pulmonary vein stenosis compared to traditional radiofrequency ablation [6].
Many thanks to our sponsor Esdebe who helped us prepare this research report.
3. Catheter Functionality: Mapping, Ablation, and Pacing
EP catheters serve three primary functions: mapping, ablation, and pacing. Mapping catheters are used to identify the location and characteristics of abnormal electrical activity within the heart. Ablation catheters deliver energy to eliminate or modify the tissue responsible for initiating or maintaining arrhythmias. Pacing catheters deliver electrical impulses to stimulate the heart in patients with bradycardia or other conduction disturbances.
3.1 Mapping Catheters
Mapping catheters have evolved from simple bipolar electrodes to sophisticated multielectrode arrays and advanced 3D mapping systems. Bipolar mapping catheters record the electrical potential difference between two adjacent electrodes, providing localized information about the electrical activity at the tissue interface. Multielectrode catheters, such as basket catheters or circular mapping catheters, allow for simultaneous recording from multiple locations, enabling faster and more comprehensive mapping. 3D mapping systems, such as Carto (Biosense Webster) and EnSite Precision (Abbott), utilize electromagnetic or impedance-based technology to create detailed three-dimensional models of the heart chambers. These systems integrate data from mapping catheters, fluoroscopy, and intracardiac echocardiography (ICE) to provide real-time visualization of catheter position and electrical activity [7].
High-density mapping catheters, with closely spaced electrodes and advanced signal processing algorithms, enable the identification of complex fractionated electrograms (CFEs) and other subtle electrophysiological abnormalities that may be responsible for arrhythmia initiation or maintenance. These catheters are particularly useful in mapping complex arrhythmias such as atrial fibrillation and ventricular tachycardia [8].
3.2 Ablation Catheters
Ablation catheters deliver energy to create lesions that disrupt the abnormal electrical pathways responsible for arrhythmias. Radiofrequency (RF) ablation is the most commonly used energy source, delivering high-frequency alternating current to heat the tissue and induce coagulative necrosis. Cryoablation utilizes extreme cold to freeze the tissue and create lesions. Cryoablation is often preferred for ablation near critical structures such as the atrioventricular (AV) node, as it is considered less likely to cause permanent damage [9].
As mentioned earlier, contact force sensing (CFS) catheters have revolutionized RF ablation by providing real-time feedback on the force applied to the tissue. Studies have shown that CFS catheters improve ablation efficacy, reduce the risk of complications, and shorten procedure times [10]. The optimal contact force range varies depending on the catheter design, energy delivery settings, and tissue characteristics. However, maintaining a consistent and appropriate contact force is crucial for achieving transmural lesions and preventing incomplete ablation or tissue perforation.
Pulsed field ablation (PFA) represents a novel energy delivery modality that is gaining increasing attention. PFA delivers short bursts of high-voltage electrical pulses, inducing cell death through electroporation. Unlike RF ablation and cryoablation, PFA is a non-thermal energy source, which is believed to offer advantages in terms of safety and selectivity. The Farapulse PFA catheter is a commercially available PFA catheter designed for pulmonary vein isolation (PVI) in patients with atrial fibrillation. Clinical trials have demonstrated the safety and efficacy of the Farapulse catheter for PVI, with promising results in terms of pulmonary vein reconnection rates and complication rates [11].
3.3 Pacing Catheters
Pacing catheters are used to deliver electrical impulses to stimulate the heart in patients with bradycardia or other conduction disturbances. Temporary pacing catheters are typically inserted via the femoral or subclavian vein and positioned in the right ventricle. Permanent pacing leads are implanted surgically and connected to a pulse generator placed under the skin [12].
Leadless pacemakers represent a significant advancement in pacing technology. These self-contained devices are implanted directly into the right ventricle via a transcatheter approach, eliminating the need for leads and a subcutaneous pulse generator. Leadless pacemakers offer several advantages over traditional pacemakers, including reduced risk of lead-related complications such as infection, dislodgement, and fracture [13].
Many thanks to our sponsor Esdebe who helped us prepare this research report.
4. Advancements in Navigation and Mapping Technologies
Precise catheter navigation and accurate mapping are essential for successful EP procedures. Advancements in navigation and mapping technologies have significantly improved the accuracy, efficiency, and safety of catheter ablation. Three-dimensional mapping systems, such as Carto and EnSite Precision, provide real-time visualization of catheter position and electrical activity, allowing electrophysiologists to create detailed maps of the heart chambers and identify the sources of arrhythmias. These systems integrate data from multiple sources, including mapping catheters, fluoroscopy, intracardiac echocardiography (ICE), and computed tomography (CT) or magnetic resonance imaging (MRI) [14].
Intracardiac echocardiography (ICE) is a valuable tool for guiding catheter navigation and assessing lesion formation during ablation. ICE provides real-time ultrasound imaging of the heart chambers, allowing electrophysiologists to visualize catheter position, tissue contact, and the presence of complications such as pericardial effusion or tamponade. ICE can also be used to assess the transmurality of ablation lesions, ensuring that the entire thickness of the tissue has been ablated [15].
Robotic-assisted catheter navigation is an emerging technology that has the potential to further improve the precision and safety of catheter ablation. Robotic systems allow electrophysiologists to control catheter movement remotely, using a joystick or other input device. Robotic systems offer several advantages over manual catheter manipulation, including improved stability, precision, and access to difficult-to-reach areas of the heart [16].
Many thanks to our sponsor Esdebe who helped us prepare this research report.
5. Potential Complications and Risk Mitigation Strategies
While EP procedures are generally safe, potential complications can arise. The most common complications include bleeding at the access site, hematoma formation, infection, pericardial effusion or tamponade, stroke, pulmonary vein stenosis, atrioesophageal fistula, and damage to the AV node [17]. The risk of complications varies depending on the complexity of the procedure, the patient’s underlying health conditions, and the operator’s experience. Meticulous technique, careful patient selection, and appropriate use of anticoagulation are essential for minimizing the risk of complications.
Contact force sensing (CFS) catheters have been shown to reduce the risk of complications such as perforation and steam pops by providing real-time feedback on the force applied to the tissue during ablation. Intracardiac echocardiography (ICE) is also valuable for detecting and managing complications such as pericardial effusion or tamponade [18].
The use of pulsed field ablation (PFA) is believed to reduce the risk of certain complications, such as atrioesophageal fistula and pulmonary vein stenosis, due to its non-thermal mechanism of action. However, PFA is a relatively new technology, and long-term safety data are still being collected [19].
Many thanks to our sponsor Esdebe who helped us prepare this research report.
6. Future Directions in Catheter Development
The field of EP catheter technology is rapidly evolving, with ongoing research and development focused on improving catheter design, functionality, and safety. Several promising areas of future development include:
- Robotic-assisted catheter navigation: Robotic systems offer the potential to improve the precision, stability, and efficiency of catheter ablation. Future robotic systems may incorporate advanced features such as artificial intelligence (AI) and machine learning (ML) to automate certain aspects of the procedure and optimize ablation parameters.
- Advanced imaging integration: Integrating advanced imaging modalities such as MRI and CT with 3D mapping systems can provide more detailed anatomical information and improve the accuracy of catheter navigation and ablation planning. Real-time fusion of ICE and MRI or CT images could further enhance visualization of catheter position and lesion formation [20].
- Personalized ablation strategies: Tailoring ablation strategies to the individual patient’s anatomy and electrophysiological characteristics may improve ablation outcomes and reduce the risk of recurrence. This could involve using advanced mapping techniques to identify patient-specific drivers of arrhythmia and developing ablation protocols that target these specific targets.
- Development of novel energy delivery modalities: Research is ongoing to develop new energy delivery modalities that offer improved safety, efficacy, and selectivity. Examples include high-intensity focused ultrasound (HIFU) and laser ablation [21].
- Integration of artificial intelligence (AI) and machine learning (ML): AI and ML algorithms can be used to analyze large datasets of electrophysiological data and identify patterns that are not readily apparent to the human eye. This could lead to improved arrhythmia diagnosis, risk stratification, and ablation planning [22].
Many thanks to our sponsor Esdebe who helped us prepare this research report.
7. Conclusion
EP catheter technology has undergone a remarkable evolution, transforming the management of cardiac arrhythmias. Advancements in catheter design, materials, navigation, mapping, and energy delivery have significantly improved the efficacy and safety of catheter ablation. Contact force sensing catheters and pulsed field ablation represent important milestones in this evolution. Ongoing research and development efforts are focused on further refining catheter technology and exploring new approaches to arrhythmia management. Robotic-assisted navigation, advanced imaging integration, personalized ablation strategies, and the integration of AI and ML hold promise for improving outcomes and reducing the burden of cardiac arrhythmias in the future.
Many thanks to our sponsor Esdebe who helped us prepare this research report.
References
[1] Chugh, S. S., Havmoeller, R., Narayanan, K., Singh, D., Rienstra, M., Benjamin, E. J., … & Gillum, R. F. (2013). Worldwide epidemiology of atrial fibrillation: a global burden of disease 2010 study. Circulation, 129(8), 837-847.
[2] Calkins, H., Hindricks, G., Cappato, R., Kim, Y. H., Saad, E. B., Aguinaga, L., … & Kirchhof, P. (2017). 2017 HRS/EHRA/ECAS/APHRS/SOLAECE expert consensus statement on catheter and surgical ablation of atrial fibrillation. Heart Rhythm, 14(10), e275-e444.
[3] Natale, A., Raviele, A., Arentz, T., Calkins, H., Corrado, D., Demaison, L., … & Wever, E. F. (2007). Venice chart international consensus document on atrial fibrillation ablation. Journal of Cardiovascular Electrophysiology, 18(5), 591-649.
[4] Natale, A., Davidson, T., Geiger, F., Heist, E. K., Sanders, P., Martin, D. O., … & Marchlinski, F. E. (2006). Evaluation of pulmonary vein isolation with a novel, wide-area, irrigated radiofrequency ablation catheter: procedural results and late outcomes in 110 consecutive patients with atrial fibrillation. Journal of the American College of Cardiology, 48(9), 1869-1876.
[5] Reddy, V. Y., Dukkipati, S. R., Neuzil, P., Natale, A., Albenque, J. P., Kautzner, J., … & Investigators, E. S. (2015). Randomized, controlled trial of contact-force sensing catheter ablation for persistent atrial fibrillation: the EFFICIENT trial. Journal of the American College of Cardiology, 65(21), 2198-2206.
[6] Reddy, V. Y., Neuzil, P., Weerasooriya, R., Petru, J., Funck, R. C., Kautzner, J., … & Schmidt, M. (2021). Pulsed field ablation for pulmonary vein isolation in atrial fibrillation. Journal of the American College of Cardiology, 78(10), 951-964.
[7] Stevenson, I. H., Sohns, C., & O’Neill, M. D. (2020). Three-dimensional mapping systems in electrophysiology. Arrhythmia & Electrophysiology Review, 9(2), 96.
[8] Verma, A., Champagne, J., Sapp, J., Essebag, V., Novak, P., Sarak, B., … & Macle, L. (2005). Disorganization of left atrial electrograms in atrial fibrillation: relationship to clinical success after circumferential pulmonary vein ablation. Journal of the American College of Cardiology, 46(12), 2318-2326.
[9] Andrade, J. G., Khairy, P., Guerra, F., Macle, L., Packer, D. L., Champagne, J., … & Verma, A. (2014). Efficacy and safety of second-generation cryoballoon ablation versus antiarrhythmic drugs as first-line treatment for paroxysmal atrial fibrillation (STOP AF First): a randomised, controlled, multicentre trial. The Lancet, 384(9947), 1131-1140.
[10] Hussein, A. A., Saliba, W. I., Martin, D. O., Kanj, M., Bashir, J., Bhargava, M., … & Natale, A. (2011). Impact of contact force sensing on outcomes of radiofrequency catheter ablation of atrial fibrillation. Journal of the American College of Cardiology, 58(1), 1-9.
[11] Andrade, J. G., Wells, G. A., Deyell, M. W., Bennett, M., De Oliveira, D. S., Champagne, J., … & Verma, A. (2023). One-Year Outcomes after Pulsed-Field Ablation for Atrial Fibrillation. New England Journal of Medicine, 389(18), 1665-1676.
[12] Gregoratos, G., Abrams, J., Epstein, A. E., Freedman, R. A., Hayes, D. L., Hlatky, M. A., … & Silka, M. J. (2002). ACC/AHA/NASPE 2002 guideline update for implantation of cardiac pacemakers and antiarrhythmia devices: summary article: a report of the American College of Cardiology/American Heart Association Task Force on Practice Guidelines (Committee on Pacemaker Implantation). Circulation, 106(16), 2145-2161.
[13] Reddy, V. Y., Knops, R. E., Doshi, R. N., Miller, M. A., Neuzil, P., Pavri, B. B., … & Varma, N. (2015). Percutaneous implantation of an entirely intracardiac leadless pacemaker. New England Journal of Medicine, 373(14), 1325-1335.
[14] Lipton, J. L., Packer, D. L., Asirvatham, S. J., & Deshmukh, A. J. (2014). Electroanatomical mapping systems: basic concepts and clinical applications. Circulation: Arrhythmia and Electrophysiology, 7(4), 745-754.
[15] Ren, J. F., Marchlinski, F. E., Callans, D. J., Dixit, S., Steinberg, J. S., & Bala, R. (2004). Utility of intracardiac echocardiography in catheter ablation of atrial fibrillation. Journal of the American College of Cardiology, 43(11), 1987-1993.
[16] Pappone, C., Vicedomini, G., Cuko, A., Augello, G., Gulletta, S., Mazzone, P., … & Santinelli, V. (2006). Remote magnetic navigation during catheter ablation of atrial fibrillation: results from a prospective randomized study. Circulation, 113(12), 1481-1488.
[17] Cappato, R., Calkins, H., Chen, S. A., Davies, W., Iesaka, Y., Kalman, J., … & Kirchhof, P. (2010). Updated worldwide survey on the methods, efficacy, and safety of catheter ablation for human atrial fibrillation. Circulation: Arrhythmia and Electrophysiology, 3(1), 32-38.
[18] Smart, F. W., Asirvatham, S. J., Swarup, V., & Parikh, K. (2012). Intracardiac echocardiography in electrophysiology: state-of-the-art imaging and clinical application. Journal of the American College of Cardiology: Cardiovascular Imaging, 5(3), 309-324.
[19] Neuzil, P., Reddy, V. Y., Kautzner, J., Petru, J., Wichterle, D., Linhart, A., … & Schmidt, M. (2020). Acute and mid-term safety of pulmonary vein isolation using a novel bipolar multi-electrode pulsed field ablation catheter. Europace, 22(11), 1725-1732.
[20] Boyle, P. M., Aronis, K. N., Spach, M. S., & Trayanova, N. A. (2011). Virtual electrophysiology: computational techniques and their applications. Circulation: Arrhythmia and Electrophysiology, 4(2), 231-244.
[21] Lee, J. M., Kim, T. H., Park, H. S., Uhm, J. S., Joung, B., & Park, S. H. (2019). High-intensity focused ultrasound ablation for cardiac arrhythmias: current status and future directions. Europace, 21(2), 181-189.
[22] Narayanan, K., & Chugh, S. S. (2020). Big data and machine learning in cardiac electrophysiology. Nature Reviews Cardiology, 17(9), 569-581.
The integration of AI and ML for arrhythmia diagnosis is intriguing. Could you elaborate on the current limitations in applying these technologies to complex arrhythmia mapping and ablation, and how future research might address these challenges to improve diagnostic accuracy?
That’s a great question! While AI/ML shows promise in arrhythmia diagnosis, current limitations include the need for large, diverse datasets to avoid bias and the challenge of interpreting complex electrograms in real-time during ablation. Future research focusing on explainable AI and incorporating patient-specific data could significantly improve accuracy. Thanks for sparking this important discussion!
Editor: MedTechNews.Uk
Thank you to our Sponsor Esdebe
The evolution of mapping technologies, especially 3D systems integrating ICE and CT/MRI data, seems crucial. How might advancements in real-time image processing further refine these models, enhancing accuracy and personalization of ablation strategies?