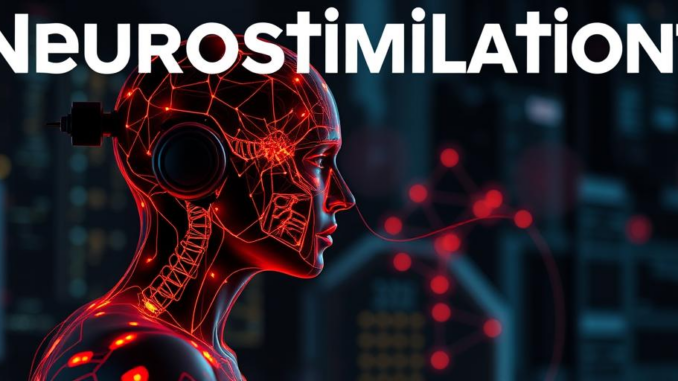
Abstract
Neurostimulation, encompassing a range of techniques that modulate neural activity through electrical or magnetic means, has emerged as a powerful tool in treating a diverse array of neurological and psychiatric disorders. This review provides a comprehensive overview of the current state of neurostimulation, exploring its underlying mechanisms of action, examining the efficacy and limitations of various techniques, and discussing future directions. We delve into the biophysical principles governing neurostimulation, focusing on how different modalities interact with neuronal tissue to induce changes in excitability and plasticity. A comparative analysis of commonly used techniques, including transcranial magnetic stimulation (TMS), transcranial direct current stimulation (tDCS), deep brain stimulation (DBS), vagus nerve stimulation (VNS), and spinal cord stimulation (SCS), is presented, highlighting their respective strengths and weaknesses across different clinical applications. Furthermore, we address the challenges associated with optimizing neurostimulation parameters, predicting individual responses, and mitigating potential side effects. Finally, we explore promising avenues for future research, including closed-loop systems, personalized stimulation protocols, and the integration of neurostimulation with other therapeutic modalities, ultimately aiming to enhance the precision and effectiveness of neurostimulation in clinical practice.
Many thanks to our sponsor Esdebe who helped us prepare this research report.
1. Introduction
Neurostimulation represents a rapidly evolving field within neuroscience and clinical neurology, offering a non-pharmacological approach to modulate brain activity and treat a wide spectrum of neurological and psychiatric conditions. Unlike traditional pharmacological interventions that rely on systemic drug delivery, neurostimulation techniques allow for targeted modulation of specific neural circuits, offering the potential for more precise and personalized therapies. The resurgence of interest in neurostimulation is driven by several factors, including a deeper understanding of brain plasticity, advancements in neuroimaging techniques that enable the identification of specific neural targets, and the development of sophisticated stimulation devices capable of delivering precise and controlled electrical or magnetic pulses.
This review aims to provide a comprehensive overview of neurostimulation, focusing on its mechanisms of action, clinical applications, challenges, and future directions. We will delve into the biophysical principles underlying different neurostimulation modalities, examining how they interact with neuronal tissue to induce changes in excitability and plasticity. We will then compare and contrast various neurostimulation techniques, including transcranial magnetic stimulation (TMS), transcranial direct current stimulation (tDCS), deep brain stimulation (DBS), vagus nerve stimulation (VNS), and spinal cord stimulation (SCS), highlighting their respective strengths and limitations across different clinical applications. A critical aspect of this review will be the discussion of the challenges associated with optimizing neurostimulation parameters, predicting individual responses, and mitigating potential side effects. Finally, we will explore promising avenues for future research, including closed-loop systems, personalized stimulation protocols, and the integration of neurostimulation with other therapeutic modalities, ultimately aiming to enhance the precision and effectiveness of neurostimulation in clinical practice. While the field of epilepsy management has seen significant advances through neurostimulation, as evidenced by techniques like VNS, RNS, and DBS, this review aims to broaden the scope, exploring the application of neurostimulation across diverse neurological and psychiatric disorders, and focusing on the fundamental principles and overarching challenges relevant to the entire field.
Many thanks to our sponsor Esdebe who helped us prepare this research report.
2. Mechanisms of Action
The mechanisms by which neurostimulation techniques modulate neural activity are complex and multifaceted, involving a cascade of events at the cellular, circuit, and systems levels. The specific mechanisms of action vary depending on the stimulation modality, parameters, and target region. However, some common principles underlie the effects of different neurostimulation techniques.
2.1 Biophysical Principles
Neurostimulation techniques, whether electrical or magnetic, ultimately exert their effects by altering the electrical activity of neurons. Electrical stimulation, as used in tDCS, DBS, VNS, and SCS, directly injects current into the neural tissue, causing changes in the transmembrane potential of neurons. This, in turn, affects neuronal excitability, making neurons more or less likely to fire action potentials. The polarity of the stimulation (anodal vs. cathodal in tDCS) determines whether the neurons are depolarized (more excitable) or hyperpolarized (less excitable), respectively. The magnitude and duration of the current, as well as the electrode configuration, influence the spatial extent and intensity of the stimulation effects.
Magnetic stimulation, as used in TMS, generates a brief, high-intensity magnetic pulse that induces an electrical current in the underlying neural tissue. This induced current can depolarize neurons and trigger action potentials, depending on the stimulation parameters and the excitability of the target neurons. TMS can be delivered in single pulses, paired pulses, or repetitive trains, allowing for different types of stimulation protocols that can either enhance or suppress neural activity.
2.2 Cellular and Circuit Level Effects
At the cellular level, neurostimulation can influence neuronal excitability, synaptic transmission, and gene expression. Changes in transmembrane potential induced by electrical stimulation can affect the gating of voltage-gated ion channels, altering the flow of ions across the neuronal membrane and influencing neuronal firing patterns. Neurostimulation can also modulate synaptic plasticity, the ability of synapses to strengthen or weaken over time, which is thought to be a crucial mechanism underlying learning and memory. Long-term potentiation (LTP) and long-term depression (LTD), two forms of synaptic plasticity, can be induced by specific neurostimulation protocols, leading to sustained changes in synaptic strength.
At the circuit level, neurostimulation can modulate the activity of specific neural networks, influencing information processing and behavior. For example, TMS applied to the motor cortex can evoke muscle contractions, while TMS applied to the prefrontal cortex can affect cognitive functions such as working memory and decision-making. DBS, which involves the implantation of electrodes deep within the brain, can modulate the activity of specific brain structures, such as the basal ganglia in Parkinson’s disease, to alleviate motor symptoms.
2.3 Systems Level Effects
At the systems level, neurostimulation can influence large-scale brain networks and affect overall brain function. For example, VNS, which involves the stimulation of the vagus nerve, can modulate the activity of the brainstem, hypothalamus, and amygdala, affecting autonomic function, mood, and arousal. TMS and tDCS can also modulate the activity of distributed brain networks, influencing cognitive and emotional processing. The effects of neurostimulation on large-scale brain networks can be measured using neuroimaging techniques such as EEG, fMRI, and PET, providing insights into the mechanisms of action and the clinical effects of neurostimulation.
2.4 Plasticity and Long-Term Effects
A key aspect of neurostimulation is its ability to induce long-term changes in brain function through mechanisms of neural plasticity. Repetitive stimulation protocols, such as repetitive TMS (rTMS) and long-term tDCS, can induce sustained changes in synaptic strength and neuronal excitability, leading to lasting improvements in symptoms. The mechanisms underlying neurostimulation-induced plasticity are thought to involve changes in gene expression, protein synthesis, and dendritic spine morphology. However, the precise mechanisms of plasticity induction and maintenance are still not fully understood and are an active area of research.
It’s important to note that the response to neurostimulation is highly variable across individuals, and the factors that contribute to this variability are not fully understood. Genetic factors, age, sex, disease state, and concurrent medications can all influence the response to neurostimulation. Furthermore, the state of the brain at the time of stimulation, including factors such as sleep, attention, and mood, can also affect the outcome of neurostimulation.
Many thanks to our sponsor Esdebe who helped us prepare this research report.
3. Neurostimulation Techniques: A Comparative Analysis
A variety of neurostimulation techniques are currently available, each with its own strengths, weaknesses, and clinical applications. This section provides a comparative analysis of commonly used techniques, including TMS, tDCS, DBS, VNS, and SCS.
3.1 Transcranial Magnetic Stimulation (TMS)
TMS is a non-invasive brain stimulation technique that uses magnetic pulses to induce electrical currents in the brain. It can be used to stimulate specific brain regions or to modulate the activity of neural circuits. TMS is typically delivered using a coil placed on the scalp, which generates a brief, high-intensity magnetic pulse that induces an electrical current in the underlying neural tissue. The frequency, intensity, and pattern of TMS pulses can be varied to produce different effects on brain activity. Single-pulse TMS can be used to probe the excitability of cortical circuits, while repetitive TMS (rTMS) can be used to induce longer-lasting changes in brain function.
Advantages:
- Non-invasive and relatively painless
- Precise targeting of specific brain regions
- Can be used to both stimulate and inhibit brain activity
- Well-established safety profile
Disadvantages:
- Limited penetration depth
- Potential for seizures (rare)
- Effects can be variable and short-lived
Clinical Applications:
- Treatment of depression, obsessive-compulsive disorder (OCD), and chronic pain
- Mapping of cortical function
- Research into cognitive processes
3.2 Transcranial Direct Current Stimulation (tDCS)
tDCS is a non-invasive brain stimulation technique that uses weak electrical currents to modulate neuronal excitability. It involves applying a constant, low-intensity current to the scalp through two or more electrodes. The polarity of the electrodes (anodal vs. cathodal) determines whether the underlying neurons are depolarized (more excitable) or hyperpolarized (less excitable), respectively. tDCS is generally well-tolerated and has a relatively low risk of side effects.
Advantages:
- Non-invasive and painless
- Easy to administer and relatively inexpensive
- Potential for long-lasting effects
Disadvantages:
- Limited spatial resolution
- Effects can be subtle and variable
- Limited penetration depth
Clinical Applications:
- Treatment of depression, chronic pain, and stroke rehabilitation
- Enhancement of cognitive functions, such as learning and memory
3.3 Deep Brain Stimulation (DBS)
DBS is an invasive neurosurgical procedure that involves implanting electrodes deep within the brain to stimulate specific brain structures. The electrodes are connected to a pulse generator implanted in the chest, which delivers electrical pulses to the target brain region. DBS is typically used to treat movement disorders such as Parkinson’s disease, essential tremor, and dystonia. It is also being investigated for the treatment of other conditions, such as depression, OCD, and epilepsy.
Advantages:
- Highly targeted stimulation of specific brain structures
- Reversible and adjustable stimulation parameters
- Significant improvement in symptoms for many patients
Disadvantages:
- Invasive surgical procedure with potential risks
- Potential for hardware-related complications, such as infection or lead fracture
- Can be expensive
Clinical Applications:
- Treatment of Parkinson’s disease, essential tremor, and dystonia
- Treatment of depression, OCD, and epilepsy (investigational)
3.4 Vagus Nerve Stimulation (VNS)
VNS is a neurostimulation technique that involves stimulating the vagus nerve, a major cranial nerve that connects the brain to the body. A small device is implanted in the chest, which delivers electrical pulses to the vagus nerve. VNS is approved for the treatment of epilepsy and depression. It is also being investigated for the treatment of other conditions, such as anxiety, migraine, and heart failure.
Advantages:
- Minimally invasive surgical procedure
- Relatively safe and well-tolerated
- Potential for systemic effects, influencing autonomic function, mood, and arousal
Disadvantages:
- Non-specific stimulation of the vagus nerve
- Potential for side effects, such as hoarseness, cough, and shortness of breath
- Effectiveness can be variable
Clinical Applications:
- Treatment of epilepsy and depression
- Treatment of anxiety, migraine, and heart failure (investigational)
3.5 Spinal Cord Stimulation (SCS)
SCS is a neurostimulation technique that involves implanting electrodes near the spinal cord to deliver electrical pulses. SCS is primarily used to treat chronic pain conditions, such as neuropathic pain, failed back surgery syndrome, and complex regional pain syndrome. The electrical pulses delivered by SCS can block pain signals from reaching the brain, providing pain relief.
Advantages:
- Effective for treating chronic pain conditions
- Reversible and adjustable stimulation parameters
- Can improve quality of life for patients with chronic pain
Disadvantages:
- Invasive surgical procedure with potential risks
- Potential for hardware-related complications, such as infection or lead migration
- Effectiveness can be variable
Clinical Applications:
- Treatment of chronic pain conditions, such as neuropathic pain, failed back surgery syndrome, and complex regional pain syndrome
Many thanks to our sponsor Esdebe who helped us prepare this research report.
4. Challenges and Future Directions
Despite the significant progress made in the field of neurostimulation, several challenges remain that need to be addressed to improve the efficacy and safety of these techniques. This section discusses some of these challenges and explores promising avenues for future research.
4.1 Optimizing Stimulation Parameters
A major challenge in neurostimulation is optimizing the stimulation parameters, such as frequency, intensity, duration, and waveform, to achieve the desired therapeutic effects. The optimal stimulation parameters can vary depending on the individual, the target brain region, and the clinical condition being treated. Developing methods to personalize stimulation parameters based on individual brain characteristics and clinical profiles is crucial for improving the efficacy of neurostimulation. This may involve using neuroimaging techniques to guide stimulation targeting and to monitor brain activity during stimulation.
4.2 Predicting Individual Responses
The response to neurostimulation is highly variable across individuals, and the factors that contribute to this variability are not fully understood. Genetic factors, age, sex, disease state, concurrent medications, and brain state at the time of stimulation can all influence the response to neurostimulation. Developing methods to predict individual responses to neurostimulation is essential for selecting the most appropriate treatment for each patient and for optimizing stimulation parameters. This may involve using machine learning algorithms to analyze clinical and neuroimaging data to identify predictors of treatment response.
4.3 Mitigating Potential Side Effects
While neurostimulation is generally considered safe, it can be associated with potential side effects. These side effects can range from mild and transient, such as headache, fatigue, and skin irritation, to more serious and persistent, such as seizures, mood changes, and cognitive impairment. Developing methods to mitigate potential side effects is crucial for ensuring the safety and tolerability of neurostimulation. This may involve using lower stimulation intensities, carefully monitoring patients for adverse effects, and developing strategies to prevent or manage side effects.
4.4 Closed-Loop Systems
Closed-loop neurostimulation systems, which adjust stimulation parameters based on real-time feedback from brain activity, hold great promise for improving the precision and effectiveness of neurostimulation. These systems can automatically adjust stimulation parameters to maintain a desired level of brain activity or to respond to specific events, such as seizures or mood fluctuations. Closed-loop systems have the potential to provide more personalized and adaptive neurostimulation therapies.
4.5 Integration with Other Therapeutic Modalities
The integration of neurostimulation with other therapeutic modalities, such as pharmacological interventions, cognitive behavioral therapy, and physical therapy, may enhance the efficacy of treatment. Combining neurostimulation with other therapies can potentially synergize the effects of each modality, leading to greater improvements in symptoms. For example, combining tDCS with cognitive training may enhance learning and memory, while combining DBS with pharmacological therapy may improve motor control in Parkinson’s disease.
4.6 Advanced Neuroimaging Techniques
Advanced neuroimaging techniques, such as high-resolution fMRI, diffusion tensor imaging (DTI), and magnetoencephalography (MEG), can provide valuable insights into the mechanisms of action of neurostimulation and can be used to guide stimulation targeting. These techniques can reveal the structural and functional connectivity of brain networks, allowing for more precise targeting of specific neural circuits. Furthermore, neuroimaging can be used to monitor brain activity during stimulation, providing real-time feedback on the effects of stimulation and allowing for adaptive adjustments of stimulation parameters.
4.7 Development of Novel Neurostimulation Techniques
The development of novel neurostimulation techniques, such as optogenetics and sonogenetics, holds great promise for the future of neurostimulation. Optogenetics involves using light to control the activity of genetically modified neurons, while sonogenetics involves using ultrasound to modulate neuronal activity. These techniques offer the potential for highly precise and targeted stimulation of specific neurons or neural circuits.
Many thanks to our sponsor Esdebe who helped us prepare this research report.
5. Conclusion
Neurostimulation has emerged as a powerful tool for modulating brain activity and treating a wide range of neurological and psychiatric disorders. While significant progress has been made in the field, several challenges remain that need to be addressed to improve the efficacy and safety of these techniques. Optimizing stimulation parameters, predicting individual responses, mitigating potential side effects, developing closed-loop systems, integrating neurostimulation with other therapeutic modalities, utilizing advanced neuroimaging techniques, and developing novel neurostimulation techniques are all promising avenues for future research. By addressing these challenges and pursuing these research directions, we can unlock the full potential of neurostimulation to improve the lives of patients with neurological and psychiatric disorders.
Many thanks to our sponsor Esdebe who helped us prepare this research report.
References
- Dayan, E., & Cohen, L. G. (2011). Neuroplasticity subserving motor skill learning. Neuron, 72(3), 443-454.
- Fox, M. D., Buckner, R. L., White, M. P., Greicius, M. D., Pascual-Leone, A., & Bestmann, S. (2012). Resting-state networks link invasive and noninvasive brain stimulation across diverse psychiatric and neurological diseases. Proceedings of the National Academy of Sciences, 109(31), E1953-E1962.
- Hallett, M. (2000). Transcranial magnetic stimulation and the human motor cortex. Trends in Neurosciences, 23(10), 429-431.
- Lefaucheur, J. P., Antal, A., Ayache, S. S., Benninger, D. H., Brunelin, J., Cogiamanian, F., … & Ziemann, U. (2017). Evidence-based guidelines on the therapeutic use of repetitive transcranial magnetic stimulation (rTMS). Clinical Neurophysiology, 128(1), 56-92.
- McIntyre, C. C., Grill, W. M., Sherman, D. L., & Thakor, N. V. (2004). Cellular effects of deep brain stimulation: model-based analysis of activation and inhibition. Journal of Neurophysiology, 91(4), 1487-1502.
- Perlmutter, J. S., & Mink, J. W. (2006). Deep brain stimulation. Annual Review of Neuroscience, 29, 229-257.
- Priori, A. (2003). Transcranial magnetic stimulation: a tool for functional exploration and therapy. Clinical Neurophysiology, 114(3), 589-595.
- Schiff, N. D., Giacino, J. T., Kalmar, K., Victor, J. D., Baker, K., Gerber, M., … & Rezai, A. R. (2007). Behavioural improvements with thalamic stimulation after severe traumatic brain injury. Nature, 448(7153), 600-603.
- Woods, A. J., Antal, A., Bikson, M., Boggio, P. S., Brunoni, A. R., Celnik, P., … & Cohen, L. G. (2016). A technical guide to tDCS, and related non-invasive brain stimulation tools. Clinical Neurophysiology, 127(2), 1031-1048.
- Ben-Menachem, E. (2002). Vagus-nerve stimulation for the treatment of epilepsy. The Lancet Neurology, 1(8), 477-482.
This is a great overview of neurostimulation techniques. The discussion of closed-loop systems is particularly interesting, especially considering the potential for personalized and adaptive therapies based on real-time feedback. How might advances in AI and machine learning further enhance the sophistication and efficacy of these closed-loop systems?
Thank you for your insightful comment! You’re spot on about the potential of closed-loop systems. AI and machine learning could drastically improve these systems by enabling more sophisticated real-time analysis of patient data, leading to highly personalized and responsive stimulation protocols. This could optimize therapeutic outcomes significantly!
Editor: MedTechNews.Uk
Thank you to our Sponsor Esdebe