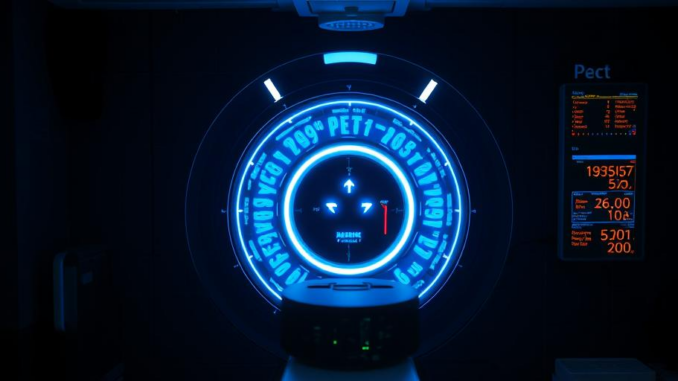
Abstract
Positron Emission Tomography (PET) has revolutionized medical imaging, offering unparalleled insights into in vivo biochemical processes. While its initial impact was primarily in oncology, PET’s applications have expanded significantly, encompassing neurology, cardiology, and immunology. This research report provides a comprehensive overview of the current state of PET imaging, focusing on its underlying principles, diverse radiotracer development, comparative analysis with other modalities (MRI, CT), clinical applications in neurological disorders and oncology, safety considerations pertaining to radiation exposure, and promising future directions, including advancements in scanner technology and the exploration of novel tracers targeting previously inaccessible biological pathways. The report critically evaluates the strengths and limitations of PET, highlighting areas where further research and technological innovation are crucial to maximizing its clinical utility and pushing the boundaries of biomedical discovery. Finally, we discuss the integration of PET with other imaging modalities and the potential of artificial intelligence to enhance data analysis and interpretation.
Many thanks to our sponsor Esdebe who helped us prepare this research report.
1. Introduction
Positron Emission Tomography (PET) is a highly sensitive and quantitative molecular imaging technique that allows for the visualization and measurement of biochemical and physiological processes in vivo. Unlike structural imaging modalities such as computed tomography (CT) and magnetic resonance imaging (MRI), PET provides functional information by detecting gamma rays emitted indirectly by a positron-emitting radionuclide (radiotracer) introduced into the body. This unique capability enables the study of a wide range of biological phenomena, including glucose metabolism, neurotransmitter activity, receptor binding, and protein synthesis, providing valuable insights into disease mechanisms and treatment responses. The ability to quantify these processes non-invasively has made PET an indispensable tool in both clinical diagnosis and biomedical research.
The development of PET technology has been marked by continuous advancements in scanner design, radiochemistry, and image reconstruction algorithms. Early PET scanners were limited by low spatial resolution and sensitivity, hindering their ability to visualize small structures and detect subtle changes in tracer distribution. However, the introduction of dedicated PET/CT and PET/MRI scanners, coupled with sophisticated iterative reconstruction techniques and improved radiotracer design, has significantly enhanced the quality and accuracy of PET imaging. These advancements have expanded the clinical applications of PET and opened new avenues for research, particularly in the fields of oncology and neurology.
Despite its many advantages, PET imaging also faces several challenges, including the relatively high cost of radiotracer production and scanner maintenance, the limited availability of certain radiotracers, and the inherent radiation exposure associated with the procedure. Furthermore, the interpretation of PET images can be complex and requires specialized expertise, particularly when dealing with novel radiotracers or heterogeneous disease states. Addressing these challenges is crucial to ensuring the widespread adoption of PET and maximizing its potential to improve patient outcomes.
Many thanks to our sponsor Esdebe who helped us prepare this research report.
2. Principles of PET Imaging
The fundamental principle underlying PET imaging is the detection of gamma rays produced during positron-electron annihilation. A radiotracer, consisting of a positron-emitting radionuclide attached to a biologically relevant molecule, is administered to the patient. As the radionuclide decays, it emits a positron, which travels a short distance (typically a few millimeters) before encountering an electron. This encounter results in annihilation, producing two 511 keV gamma rays that are emitted in approximately opposite directions (180 degrees).
These gamma rays are detected by an array of detectors surrounding the patient. The detectors are typically composed of scintillators, which convert the gamma rays into light, and photomultiplier tubes (PMTs), which amplify the light signal. By identifying coincident pairs of gamma rays (i.e., those detected simultaneously and traveling along a line of response), the location of the annihilation event can be determined. This information is then used to reconstruct a three-dimensional image of the radiotracer distribution within the body.
The spatial resolution of PET imaging is limited by several factors, including the positron range (the distance the positron travels before annihilation), the size and arrangement of the detectors, and the statistical noise inherent in the data. Modern PET scanners typically achieve a spatial resolution of 4-6 mm, which is sufficient for visualizing many anatomical structures but may be insufficient for detecting very small lesions or subtle changes in tracer uptake. Advancements in detector technology, such as the development of silicon photomultipliers (SiPMs) and time-of-flight (TOF) PET, are improving the spatial resolution and sensitivity of PET imaging.
TOF-PET is a technique that measures the time difference between the detection of the two annihilation photons. This information can be used to further refine the location of the annihilation event along the line of response, improving image quality and reducing noise. The accuracy of TOF-PET depends on the timing resolution of the detectors, with better timing resolution leading to improved image quality. In recent years, significant progress has been made in developing detectors with picosecond timing resolution, paving the way for ultra-high resolution TOF-PET scanners.
Many thanks to our sponsor Esdebe who helped us prepare this research report.
3. Radiotracers in PET Imaging
The choice of radiotracer is critical to the success of PET imaging. The radiotracer must be chemically stable, biologically inert (to avoid altering the process being studied), and have a high affinity for the target of interest. Furthermore, the radiotracer must be easily synthesized and have a suitable half-life for imaging. The most commonly used radionuclide in PET imaging is fluorine-18 (¹⁸F), which has a half-life of 109.8 minutes and can be readily incorporated into a wide range of molecules. Other commonly used radionuclides include carbon-11 (¹¹C, half-life 20.4 minutes), nitrogen-13 (¹³N, half-life 9.97 minutes), oxygen-15 (¹⁵O, half-life 2.03 minutes), and gallium-68 (⁶⁸Ga, half-life 67.6 minutes).
3.1 Oncology
In oncology, ¹⁸F-fluorodeoxyglucose (¹⁸F-FDG) is the most widely used radiotracer. ¹⁸F-FDG is an analog of glucose that is taken up by cells but cannot be metabolized, resulting in its accumulation in metabolically active tissues, such as tumors. ¹⁸F-FDG PET is used to detect, stage, and monitor the response to therapy in a wide range of cancers. The increased glucose metabolism in cancer cells allows for increased uptake and detection compared to normal cells. Beyond FDG, research is actively developing targeted tracers that bind with greater specificity to certain cancers. Examples include tracers for prostate-specific membrane antigen (PSMA) in prostate cancer and somatostatin receptor analogues for neuroendocrine tumors.
3.2 Neurology
In neurology, PET is used to study a variety of brain disorders, including Alzheimer’s disease, Parkinson’s disease, and epilepsy. Several radiotracers have been developed for imaging amyloid plaques and tau tangles, the pathological hallmarks of Alzheimer’s disease. These tracers allow for the early detection and diagnosis of Alzheimer’s disease, as well as the monitoring of disease progression and the evaluation of potential therapies. Tracers for dopamine transporters are also valuable in diagnosing and monitoring Parkinson’s disease and other movement disorders. Additionally, ¹⁸F-FDG PET is used to identify seizure foci in patients with epilepsy.
3.3 Cardiology
In cardiology, PET is used to assess myocardial perfusion and viability. Rubidium-82 (⁸²Rb) and nitrogen-13 ammonia (¹³N-NH₃) are commonly used radiotracers for myocardial perfusion imaging. These tracers are taken up by cardiomyocytes in proportion to blood flow, allowing for the detection of coronary artery disease. ¹⁸F-FDG PET can also be used to assess myocardial viability, differentiating between ischemic but viable tissue and infarcted tissue.
3.4 Novel Radiotracers
The development of novel radiotracers is a rapidly growing area of research. Researchers are developing tracers that target a wide range of biological processes, including inflammation, angiogenesis, and apoptosis. These tracers have the potential to provide new insights into disease mechanisms and to guide the development of more effective therapies. For example, tracers targeting the programmed cell death protein 1 (PD-1) and its ligand PD-L1 are being developed to assess the efficacy of immunotherapy in cancer patients. The development of radiotracers that can cross the blood-brain barrier is particularly important for studying neurological disorders. The design and synthesis of these novel tracers requires multidisciplinary expertise in chemistry, biology, and medicine.
Many thanks to our sponsor Esdebe who helped us prepare this research report.
4. PET vs. Other Imaging Modalities
PET offers several advantages over other imaging modalities, such as CT and MRI. PET is more sensitive than CT and MRI for detecting subtle changes in tissue metabolism and function. PET can also provide quantitative information about tracer uptake, allowing for the accurate measurement of biological processes. However, PET has lower spatial resolution than CT and MRI, limiting its ability to visualize fine anatomical details. Furthermore, PET involves exposure to ionizing radiation, whereas MRI does not.
4.1 PET/CT
The combination of PET and CT in a single scanner provides both functional and anatomical information. CT images can be used to correct for attenuation of the gamma rays emitted during PET imaging, improving the accuracy of PET quantification. CT images can also be used to localize areas of abnormal tracer uptake seen on PET images. PET/CT is widely used in oncology for staging and monitoring cancer, as well as in cardiology for assessing myocardial perfusion and viability. The registration of the PET and CT images is crucial for accurate interpretation and requires sophisticated image processing algorithms. Artifacts arising from metal implants or respiratory motion can pose challenges for accurate registration and attenuation correction.
4.2 PET/MRI
PET/MRI is a more recent development that combines the high soft tissue contrast of MRI with the functional information provided by PET. MRI provides detailed anatomical information and can be used to assess tissue perfusion, diffusion, and metabolism. PET/MRI offers several advantages over PET/CT, including lower radiation exposure and improved soft tissue contrast. However, PET/MRI scanners are more expensive than PET/CT scanners, and the presence of the MRI scanner can interfere with the performance of the PET detectors. Furthermore, the integration of PET and MRI requires sophisticated hardware and software solutions. PET/MRI is particularly useful in neurology for studying brain disorders, as well as in oncology for imaging soft tissue tumors.
4.3 Hybrid Imaging
The trend in medical imaging is towards hybrid imaging, which combines the strengths of different modalities to provide a more complete picture of disease. In addition to PET/CT and PET/MRI, other hybrid imaging techniques include SPECT/CT and ultrasound/MRI. These hybrid imaging techniques have the potential to improve diagnostic accuracy, guide treatment decisions, and accelerate drug development. The development of new hybrid imaging techniques requires close collaboration between physicists, engineers, and clinicians.
Many thanks to our sponsor Esdebe who helped us prepare this research report.
5. Clinical Applications in Neurological Disorders
PET imaging plays a crucial role in the diagnosis, prognosis, and management of various neurological disorders. Its ability to visualize and quantify brain function at the molecular level provides valuable insights into disease mechanisms and treatment responses. Specific applications include:
5.1 Alzheimer’s Disease
As mentioned earlier, amyloid and tau PET imaging are used to detect and quantify the presence of amyloid plaques and tau tangles in the brain, the pathological hallmarks of Alzheimer’s disease. These tracers allow for the early diagnosis of Alzheimer’s disease, even before the onset of clinical symptoms. Amyloid PET imaging is also used to identify individuals at risk of developing Alzheimer’s disease and to monitor the effectiveness of disease-modifying therapies. The advent of tau PET imaging has been particularly significant, as it is more closely correlated with cognitive decline than amyloid PET. However, standardization of acquisition and analysis protocols remains a challenge in the field.
5.2 Parkinson’s Disease
PET imaging is used to assess dopamine transporter function in the brain, which is reduced in Parkinson’s disease. Tracers such as ¹⁸F-DOPA and ¹¹C-CFT are used to image the dopamine transporter, allowing for the diagnosis of Parkinson’s disease and the differentiation between Parkinson’s disease and other movement disorders. PET imaging can also be used to monitor the progression of Parkinson’s disease and to evaluate the effectiveness of dopaminergic therapies. Furthermore, research is underway to develop tracers that target other pathological processes in Parkinson’s disease, such as alpha-synuclein aggregation and neuroinflammation.
5.3 Epilepsy
¹⁸F-FDG PET is used to identify seizure foci in patients with epilepsy. During the interictal period (the period between seizures), the glucose metabolism in the seizure focus is often reduced, which can be detected by ¹⁸F-FDG PET. This information can be used to guide surgical resection of the seizure focus, improving seizure control in patients with drug-resistant epilepsy. The sensitivity of ¹⁸F-FDG PET for detecting seizure foci can be improved by using specialized imaging protocols and advanced image analysis techniques. Furthermore, PET imaging can be used to study the underlying mechanisms of epilepsy, such as alterations in neurotransmitter activity and neuronal excitability.
5.4 Other Neurological Disorders
PET imaging is also used to study other neurological disorders, such as multiple sclerosis, Huntington’s disease, and stroke. In multiple sclerosis, PET imaging can be used to assess neuroinflammation and demyelination. In Huntington’s disease, PET imaging can be used to assess striatal metabolism and to monitor disease progression. In stroke, PET imaging can be used to assess tissue viability and to guide thrombolytic therapy. The application of PET in these disorders is often more complex and requires careful consideration of the specific pathophysiology and clinical context.
Many thanks to our sponsor Esdebe who helped us prepare this research report.
6. Safety Aspects and Radiation Exposure
PET imaging involves exposure to ionizing radiation, which carries a potential risk of long-term health effects, such as cancer. The radiation dose from a typical PET scan is comparable to that from a CT scan. However, the radiation dose can vary depending on the radiotracer used, the imaging protocol, and the patient’s body size. It is important to minimize radiation exposure by using the lowest possible dose of radiotracer that provides adequate image quality, optimizing the imaging protocol, and shielding the patient during the scan. Also, the impact of PET scans on women of child-bearing age is a high consideration, and doctors will take appropriate steps when dealing with these patients.
The risks and benefits of PET imaging must be carefully considered before each scan. The benefits of PET imaging, such as improved diagnosis and treatment planning, often outweigh the risks of radiation exposure. However, in some cases, alternative imaging modalities, such as MRI, may be preferred to avoid radiation exposure. It is important to inform patients about the risks and benefits of PET imaging and to obtain their informed consent before the scan. The establishment of standardized radiation safety protocols and training programs for PET technologists and physicians is crucial to minimizing radiation exposure and ensuring patient safety.
Many thanks to our sponsor Esdebe who helped us prepare this research report.
7. Future Advancements
PET imaging is a rapidly evolving field, with ongoing advancements in scanner technology, radiotracer development, and image analysis techniques. Future advancements are expected to further improve the spatial resolution, sensitivity, and accuracy of PET imaging, as well as to expand its clinical applications.
7.1 Higher Resolution Scanners
Ongoing research is focused on developing PET scanners with higher spatial resolution. These scanners will allow for the visualization of smaller structures and the detection of more subtle changes in tracer uptake. One approach to improving spatial resolution is to use smaller detectors and to arrange them in a more compact configuration. Another approach is to use silicon photomultipliers (SiPMs), which have higher sensitivity and faster timing resolution than traditional photomultiplier tubes. Furthermore, the development of novel image reconstruction algorithms can improve the spatial resolution of PET images.
7.2 Novel Tracers
The development of novel radiotracers is a major focus of research. Researchers are developing tracers that target a wide range of biological processes, including inflammation, angiogenesis, and apoptosis. These tracers have the potential to provide new insights into disease mechanisms and to guide the development of more effective therapies. For example, tracers targeting the immune system are being developed to assess the response to immunotherapy in cancer patients. The development of radiotracers that can cross the blood-brain barrier is particularly important for studying neurological disorders. Artificial Intelligence is showing great potential in streamlining this process.
7.3 Artificial Intelligence
Artificial intelligence (AI) is playing an increasingly important role in PET imaging. AI algorithms can be used to improve image reconstruction, reduce noise, and automate image analysis. AI can also be used to predict treatment response and to personalize therapy. For example, AI algorithms can be trained to identify patterns in PET images that are associated with treatment response. These algorithms can then be used to predict which patients are most likely to benefit from a particular therapy. AI is also being used to accelerate radiotracer discovery and development. For example, AI algorithms can be used to predict the binding affinity of new radiotracers to their targets.
7.4 Multimodal Imaging
The integration of PET with other imaging modalities, such as MRI and CT, is expected to continue to grow in the future. Multimodal imaging provides both functional and anatomical information, allowing for a more complete picture of disease. Future multimodal imaging systems may integrate even more modalities, such as ultrasound and optical imaging. The development of new multimodal imaging techniques requires close collaboration between physicists, engineers, and clinicians.
Many thanks to our sponsor Esdebe who helped us prepare this research report.
8. Conclusion
Positron Emission Tomography (PET) is a powerful molecular imaging technique that has revolutionized medical diagnosis and research. Its ability to visualize and quantify biochemical processes in vivo provides invaluable insights into disease mechanisms and treatment responses. While PET has made significant strides, challenges remain, including the need for higher spatial resolution, the development of novel radiotracers, and the reduction of radiation exposure. Future advancements in scanner technology, radiochemistry, and image analysis techniques, coupled with the integration of artificial intelligence and multimodal imaging, hold the promise of further expanding the clinical utility of PET and pushing the boundaries of biomedical discovery. Overcoming the limitations and capitalizing on the emerging opportunities will ensure that PET continues to play a central role in shaping the future of personalized medicine.
Many thanks to our sponsor Esdebe who helped us prepare this research report.
References
- Bailey, D. L., Townsend, D. W., Valk, P. E., & Maisey, M. N. (2005). Positron emission tomography: basic sciences. Springer.
- Cherry, S. R., Sorenson, J. A., & Phelps, M. E. (2012). Physics in nuclear medicine. Elsevier health sciences.
- Phelps, M. E. (2006). Positron emission tomography provides molecular imaging of biological processes. Proceedings of the National Academy of Sciences, 103(48), 17971-17978.
- Rahmim, A., Zaidi, H., Tsoumpas, C., Kontaxakis, G., & Burger, C. (2013). Principles of time-of-flight PET: technology and clinical applications. European Journal of Nuclear Medicine and Molecular Imaging, 40(2), 241-258.
- Levin, C. S. (2018). Instrumentation for positron emission tomography. Seminars in Nuclear Medicine, 48(4), 275-290.
- Kepe, V., Bordelon, Y., Boxer, A., Huang, S. C., & Coppola, G. (2013). PET imaging of neuropathology in neurodegenerative diseases. Neurotherapeutics, 10(4), 659-672.
- Joshi, A. D., Pontecorvo, M. J., Clark, T., Navitsky, M., Carpenter, A. P., Jennings, D., … & Skovronsky, D. M. (2012). Performance of amyloid PET with florbetapir F 18 in the detection of Alzheimer’s disease pathology. JAMA neurology, 69(10), 1290-1297.
- Villemagne, V. L., & Okamura, N. (2019). In vivo tau imaging: obstacles and progress. Alzheimer’s research & therapy, 11(1), 1-12.
- Jennings, D. L., & Van Brocklin, H. F. (2021). Radiochemistry and radiopharmaceutical development for immuno-PET. Journal of Nuclear Medicine, 62(3), 309-317.
- Zaidi, H., Tsoumpas, C., Mawlawi, O., & Behe, M. (2012). The emerging role of hybrid PET/MRI in clinical oncology. Seminars in Nuclear Medicine, 42(2), 75-88.
- Gillies, R. J., Schirner, G. B., & Gatenby, R. A. (2018). The role of positron emission tomography in personalized medicine. Nature Reviews Clinical Oncology, 15(9), 569-580.
- Ljungberg, M., Strand, S. E., & King, M. A. (2020). SPECT and PET instrumentation. CRC press.
- Blazey, T. M., & Benzinger, T. L. S. (2018). Amyloid and tau imaging in the clinical evaluation of Alzheimer’s disease. Seminars in Neurology, 38(1), 060-067.
- Brooks, D. J., & Piccini, P. (2006). Imaging in Parkinson’s disease: the role of PET and MRI. Journal of Neurology, Neurosurgery & Psychiatry, 77(6), 709-717.
- Spencer, S. S. (2002). The relative contributions of MRI, SPECT, and PET imaging in epilepsy. Epilepsia, 43(1), 26-42.
The exploration of AI to streamline radiotracer discovery is particularly exciting. Accelerating the development of targeted tracers could significantly impact personalized medicine and treatment efficacy across various diseases.
Thank you for highlighting the role of AI in radiotracer development. The potential to accelerate the discovery of targeted tracers is indeed transformative. This could revolutionize personalized medicine, making treatments more effective and tailored to individual patient needs, significantly improving outcomes across various diseases.
Editor: MedTechNews.Uk
Thank you to our Sponsor Esdebe
This report highlights the remarkable progress in PET imaging for neurology, particularly in early detection of diseases like Alzheimer’s using amyloid and tau tracers. Further research standardizing acquisition and analysis protocols will be crucial to improving diagnostic accuracy and monitoring therapeutic interventions.
Thank you for your comment! I agree that standardizing acquisition and analysis protocols is key. Wider adoption of consistent methodologies will ensure more reliable diagnostic outcomes, particularly as PET imaging becomes more integrated into clinical trials for therapeutic interventions. This is an exciting area of development!
Editor: MedTechNews.Uk
Thank you to our Sponsor Esdebe
So, PET scans can now see inside us on a *molecular* level? Does this mean my dodgy excuses for skipping gym class (“My mitochondria are acting up!”) might actually be verifiable… or worse, proven false?